Abstract
Low aqueous solubility of drug candidates is an ongoing challenge and pharmaceutical manufacturers pay close attention to amorphization (AMORP) technology to improve the solubility of drugs that dissolve poorly. Amorphous drug typically exhibits much higher apparent solubility than their crystalline form due to high energy state that enable them to produce a supersaturated state in the gastrointestinal tract and thereby improve bioavailability. The stability and augmented solubility in co-amorphous (COA) formulations is influenced by molecular interactions. COA are excellent carriers-based drug delivery systems for biopharmaceutical classification system (BCS) class II and class IV drugs. The three important critical quality attributes, such as co-formability, physical stability, and dissolution performance, are necessary to illustrate the COA systems. New amorphous-stabilized carriers-based fabrication techniques that improve drug loading and degree of AMORP have been the focus of emerging AMORP technology. Numerous low-molecular-weight compounds, particularly amino acids such as glutamic acid, arginine, isoleucine, leucine, valine, alanine, glycine, etc., have been employed as potential co-formers. The review focus on the prevailing drug AMORP strategies used in pharmaceutical research, including in situ AMORP, COA systems, and mesoporous particle-based methods. Moreover, brief characterization techniques and the application of the different amino acids in stabilization and solubility improvements have been related.
Graphical Abstract

Similar content being viewed by others
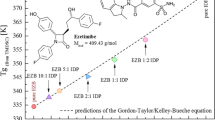
Explore related subjects
Discover the latest articles, news and stories from top researchers in related subjects.Avoid common mistakes on your manuscript.
Introduction
An increasing number of new pharmaceutical drugs exhibit poor aqueous solubility with insufficient and unpredictable bioavailability as well as gastrointestinal mucosal irritation, which is likely to cause challenges during their development into oral drug products. The most crucial rate-limiting factor for drugs taken orally is solubility, which allows to achieve the desired concentration of the drug in the systemic circulation for pharmacological response [1]. The pharmaceutically active ingredient with low solubility indicates a higher chance of failure for drug innovation and development. Poor solubility also has a considerable negative effect on pharmacodynamics, pharmacokinetics, drug distribution, absorption, and protein binding, which significantly effect drug delivery [2]. Moreover, the poor water-soluble drugs diminish the rate of in vitro dissolution and give a significant adverse impact on the delivery of drugs. Over 40% of the new chemical entities (NCEs) formulated by pharmaceutical manufacturers are practically encountering solubility issues [3]. This solubility concerns of NCEs can be addressed by amorphization (AMORP) of drug. The use of modified molecular architecture such as polymeric amorphous solid dispersions (PASDs) has been offered a novel substitute to overcome solubility restrictions, giving the amorphous drug high-energy and thermodynamic properties; however, such approach drives them to devitrification and limiting their market applicability [4]. Designing effective and reliable amorphous drug delivery systems require a deeper knowledge of thermodynamics and molecular-level phenomena such as fragility, glass transition (Tg), molecular mobility, devitrification, and molecular interactions between polymer and drug [5]. When amorphous pharmaceuticals are molecularly engineered employing polymers as carriers, the solid dispersion products are stabilized with greater control [6]. Recently, researchers had significantly improved control over the formation and maintenance of super saturations as well as solid-state stability that can develop delivery systems with desirable and predictable features as well as a workable solution for solubilizing difficult-to-solubilize pharmaceuticals. A prospective replacement for PASDs is the co-amorphous (COA) system, a newcomer to the amorphous field. The COA are single-phase and amorphous solid systems composed of two or more tiny molecules that include a mixture of drugs and an excipient [7] (Fig. 1). Among the several excipients investigated and reported for COA production, amino acid have gained significant attention. This might be due to the increase in Tg and to the homogeneous molecular-level dispersions achieved by high energy mixing. In addition, impregnation with small molecules such as amino acid is considered critical for preventing recrystallization. In most of the studies, the physical stability of such systems is attributed to intermolecular interactions such as hydrogen bonds, π-π, or even ionic interaction [8] (Fig. 2). Moreover, COAs dispersion led to improve stability even in the absence of interactions due to molecular mixing.
Classification of amorphous mixtures based on co-formers. Reproduce with permission from [9] Copyright 2022, Elsevier
Improved solubility of COA systems due to intermolecular interactions. Reproduce from [10] under CCBY 4.0
The COA offer several benefits including enhanced physical stability and water solubility of amorphous drugs, which have the potential to improve therapeutic efficacy. In addition to dissolution improvement, COA help to prevent recrystallization during storage or upon contact with biological fluids, through molecular interactions. Although a high dose is preferred for a COA in case of solid dispersion-based approach; however, in case of amino acids (AAs) based COA, a small quantity of co-formers is required due to their low molecular weight, biocompatibility, and anti-plasticizers property by increasing the Tg, while blocking drug-drug interaction and delay in recrystallization. Therefore, the selection of AAs is based on knowledge of possible drug-receptor interactions at biological binding sites. Henceforth, improved dissolution rate and physical stability of COA drug-AAs systems, compared with amorphous drug been reported, due to molecular interaction [11, 12]. However, in some case, stable systems with non-interacting components are also possible [13].
There are several approaches adopted to improve solubility and bioavailability. Among them, one common approach to enhance the amorphous drug's stability is by incorporating amorphous drugs into amorphous polymers to form glass solutions, defined as molecular mixers consisting drug and a hydrophilic polymer [14]. The competent physical stability of the amorphous systems is revealed by their superior Tg, compared to pure or native drug Tg [15]. Amino acids are capable to surge the dissolution behavior of the poorly water-soluble drugs over their individual and crystalline amorphous forms and stabilize the amorphous state of a drug [16]. Amino acids such as tyrosine (Tyr), phenylalanine (Phel) tryptophan (Trph), arginine (Arg), etc., were reported to significantly improved the dissolution behavior of indomethacin (IND) and carbamazepine (CRB) [11]. While, non-polar amino acids [17] such as leucine [18], Trph, isoleucine (Iso), valine (Val), PHEL, methionine (Met), and proline (Pro) are first-generation amino acids used as a co-former for a specific medication in a COA formulation. In the case of an acidic active entity, basic AAs are ideal to produce amorphous salts. However, acidic AAs are typically poor co-formers for COA [19].
Nikola et al. investigated AAs including Trph, lysine (Ly), and Phel to improve the carvedilol (CRD) dissolution properties using ball milling (BM) techniques to fabricate the COA of CRD. To determine whether formulations have the potential to cause CRD to become supersaturated, the dissolution performance of milled mixtures was compared with untreated CRD under non-sink conditions. The researchers observed that among tested excipients Trph was found suitable to fabricate COA with CRD. Samples containing CRD and Ly in ratios of 1:0.5, 1:1, and 1:2 M produced the highest level of carvedilol supersaturation [20]. In another study, an attempt was made to improve the solubility and bioavailability of piroxicam using encapsulation. The researchers used solvent evaporation and electro-spraying approach to formulate different samples consisting of gelatin and piroxicam. The gelatin nanocontainers loaded with piroxicam showed improvement in solubility and payload release in water. In addition, the optimized formulation released 86.32 ± 11.15% of the payload in 10 min, which is higher than those pure piroxicam (12.58 ± 6.38%). Additionally, the nanocontainers comprising gelatin and piroxicam (8:1) ratio demonstrated approximately 600-fold higher drug solubility, compared to pure piroxicam indicating gelatin-based nano-formulations are also a promising method for improving the drug's biopharmaceutical performance [21].
The dissolution rate of most of mixtures consisting of COA drugs and AAs is much faster as compared to their crystalline and amorphous drug. As a result, AAs are an attractive excipient for addressing issues related to the dissolution and stability of amorphous drugs [11]. The objective of the review is to discuss different amino acids responsible for achieving AMRP. Moreover, the review also presents the recent techniques employed for AMORP to improve the poorly soluble drug solubility and bioavailability including co-amorphization and in situ AMORP. Additionally, the formulation aspects of AAs-based excipients dosage forms and different techniques employed to characterize those formulations are also part of this review.
Classification of Drugs Based on the Biopharmaceutical Classification System
A predictive method for evaluating potential formulation effects on oral medication bioavailability in humans is the biopharmaceutical classification system (BCS) [22]. The BCS is a crucial tool for facilitating both regulatory and product development choices [23]. The rate-limiting factors influencing the oral drug absorption rate that can be determined by knowing a compound’s solubility and permeability in relevant fluids through biological membranes [24]. This knowledge is extremely helpful in forecasting the potential impact of formulation and physiological factors on the bioavailability of oral medications [25]. The classification of drugs based on solubility and permeability is depicted in Fig. 3.
Solubility challenges that plague the oral drug-delivery system. The uses of BCS to define drug permeability and solubility as metrics for oral absorption. The four categories include BCS Class I (high solubility, high permeability), Class II (low solubility, high permeability), Class III (high solubility, low permeability), and Class IV (low solubility, low permeability). The pie charts to the right show the estimated distribution of marketed and pipeline drugs by BCS classes. Adapted with permission from [26] Copyright 2018 Bioconjugate chemistry
The BCS class IV drugs demonstrate very low oral bioavailability and are more likely to demonstrate extremely high inter- and intra-subject variability due to their weak solubility and permeability profile [27, 28]. Therefore, they are typically poor candidates for oral drugs, unless the drug dose is very low. However, other estimates claim that only 5% of the top oral medications in the world fall into this category. The liquid-solid technology [29], polymeric nanoparticulate systems [30], crystal engineering [31], lipid-based delivery system [32], and P-efflux inhibition strategies [33] are employed to surge the bioavailability of such class IV drugs [34].
The co-amorphous systems (COAs) benefits include enhanced amorphous drugs water solubility and physical stability that have the potential to improve therapeutic efficacy [35, 36]. The COAs is a homogeneous single-phase system combining two compatible drugs with a compatible small-molecule co-former. Due to the higher energy present in an amorphous state and minimum energy required for crystal lattice rearrangement at the time of dissolution, COA solid dispersions (SDs) offer excellent improvement of drug solubility [37]. COAs enhance stability by inhibiting individual component crystallization, resulting in a stable, non-crystalline blend. This molecular arrangement prevents phase separation, ensuring prolonged storage stability. Deng and co-worker study investigated the co-amorphous salt systems of Norfloxacin, assessing the influence of molecular descriptors on their formation and physical stability. Based on quantitative calculations using Pearson correlation coefficient and Ridge regression, it is evident that molecular descriptors significantly influence Norfloxacin co-amorphous formation, with structural parameters (Weight, Rings) demonstrating stronger effects than the miscibility parameter Δ and pKa. The molecular descriptors associated with π···π interactions, molecular miscibility, and ionic interactions play a pivotal role in determining physical stability [38]. Correspondingly, the AMORP offers several advantages including superior conformational flexibility, anti-plasticizing effect, molecular level mixing, higher miscibility, phase separation effect, and prevent crystallization with counter part of demerits (13) [39]. However, AMORP suffers with some drawbacks such as drug polymer missibility, drug recrystallization due to poor micibility, and variable chain extension during preparation.
Several COA mixture of curcumin with artemisinin prepared by rota-vaporization have successfully indicated improved Cmax (1.4 to 35 times) and the area under the curve (1 to 4 times) with reduced time for peak drug concentration (Tmax) (40). The major cause of these developments is due to strong solid-state interactions between the components. Additionally, the COA mixtures’ stability is a result of an increase in Tg and the homogenous molecular-level dispersions produced by high-energy mixing [40, 41]. Even in the absence of interactions brought on by molecule mixing, COA dispersions result in improved stability. Although there were no discernible intermolecular interactions in the COA SDs system of glipizide (GLP)/simvastatin (SIM), improvements concerning solubility and stability were displayed after cryo-milling [42] and ball milling (BM) [13]. Moreover, COA have been used as a promising formulation approach to enhance the performances of poorly water-soluble drugs including dissolution, solubility, bioavailability stability, and mechanical characteristics [43].
In Anacetrapib, a BCS class IV drug, the bioavailability and dissolution rate were enhanced by formulating a COA-based solid dispersion with chito-oligosaccharide [44]. Anand and co-worker studied the potential of COA materials comprising lopinavir and ritonavir to produce a supersaturated state with a reduced amorphous solubility ascribed to the mole fraction of the compound present in the COA substance. Interestingly, the drug reflux from the COA material at a ratio of 2:1 was found greater than the flux of the individual drugs in the amorphous form. A considerable surge in flux was attributed to superior drug release characteristics brought on by the drug-rich nano-sized phases precipitation [45].
Amorphization of Low Soluble Drugs Using Amino Acids
The active state of a solid material is represented by the amorphous form of pharmaceuticals with benefits of bioavailability and excellent dissolution [46]. The substances is referred to as being in an amorphous shape or glassy condition when the drugs solid from is devoid of a long-range, three-dimensional molecular order [47]. It is expected that the amorphous form of active materials demonstrates improvement in thermodynamic properties in terms of vapor pressure and solubility since it has a higher internal energy and specific volume than that of crystalline form. Poorly soluble drugs demonstrate higher solubility in their amorphous form than in crystalline form [48]. Therefore, AMORP is the transformation of a crystalline substance into an amorphous state, characterized by the disorganized drug molecules arrangement in solid form [49]. Amorphous solids are comprised of short-range random molecules, whereas crystalline solids are made up of long-range organized molecules. Drug molecules present in the amorphous form exhibit superior Gibb’s free energy [50], compared to molecules present in a crystalline state due to the absence of a long-range repeating structure that causes weaker intermolecular attraction forces as well as loose packing of molecules [51].
Amino acids as carriers offer advantages in improving the solubility and dissolution properties of drugs, thereby enhancing their bioavailability. This is especially beneficial for drugs with low water solubility, as it improves their absorption in the gastrointestinal tract. These carriers, including albumin, gelatin, and casein, effectively enhance the solubility and dissolution rate of drugs with limited water solubility. Moreover, through the formation of amorphous SDs with amino acid, drug molecules are uniformly dispersed, resulting in amplified surface area and improved kinetics of drug release [52].
Nadaf and coworkers investigated a porous starch (POS) derived from Vigna radiata as a solubilizer for albendazole (ALBZ), which is low soluble [53] drug using a solvent exchange method. Additionally, the solubilization capacity of poloxamer (POXR) was evaluated by formulating tablets with SDs of ALBZ-POS at various ratios, ranging from 1:0.5 to 1:2. POS was found to possess favorable compressibility properties, as indicated by good compressibility index of 6.58. A solid dispersion formulation with a drug-to-carrier ratio of 1:2 exhibited a significant enhancement in both the dissolution rate, with a remarkable 37-fold increase, and the anthelmintic activity of ALBZ, displaying an improvement ranging from 1.7 to 3.25 times. The amorphous nature of ALBZ was confirmed through differential scanning calorimetry (DSC) and P-XRD analysis. Furthermore, the dissolution rate of SD-ALBZ-POS was approximately 2.15-fold higher than that of the commercially available formulation [54]. The oral bioavailability of Decoquinate (DCQ) was improved via SDs using mechanochemical technology, to encapsulate the drug and improve its absorption when taken orally. The SD-DCQ developed through self-assembly in water, resulting in the fabrication of micelles composed of nanoparticles (NPs) containing disodium glycyrrhizinate were characterized by outer layers consisting of protamine and anionic hyaluronic acid. The rate at which SD-DCQ dissolves and the released overtime were greater, compared to pure DCQ indicating higher bioavailability of 6.5-folds (SD-DCQ) more than that of pure DCQ. Furthermore, the biodistribution and pharmacokinetic analyses revealed that SD-DCQ exhibited superior levels in the bloodstream and a preference for accumulating in liver tissue, compared to pure DCQ [55].
Techniques of Amorphization to Improve Solubility and Bioavailability
The most widely used approaches to prepare amorphous forms in pharmaceuticals systems include polymer glass solutions or pure drugs, classified based on principal transformation mechanisms [56]. Often, crystalline material undergoes an intermediate transformation into a non-crystalline state that is thermodynamically unstable (either a melt or a solution) and the thermodynamically unstable amorphous solid material is then prepared by quench-cooling of the melt or rapid precipitation from solution, e.g., during spray drying. A second transformation mechanism involves direct solid conversions from the crystalline to the amorphous forms, such as in mechanical activation (milling). While in melt and solution mechanical activation may not cause complete disruption of the molecular order [57]. Mesoporous particle-based AMORP, COA, and in situ, AMORP are emerging approaches to AMORP for poorly water-soluble substances [58] (Fig. 4).
Fabrication processing techniques involved with COA systems. Reproduce from [10] under CCBY 4.0
Co-Amorphization Techniques
The COAs have evolved as a substitute for the traditional PASD that has been thoroughly examined to enhance the solubility and bioavailability of poorly water-soluble drugs for decades. The term “co-amorphous” was concocted to distinguish amorphous systems made up of low-molecular-weight compounds from PASDs [59, 60]. https://doi.org/10.1016/j.ejpb.2008.06.022The drug delivery systems with COA techniques demonstrated the potential to improve the bioavailability of poorly soluble drugs by surging the drug dissolution due to the material’s amorphous character. The COAs are known as the single-phase homogeneous system that uses numerous low molecular weight components rather than high molecular weight polymers [61]. In comparison to PASD, COAs exhibit significant conformational flexibility and excellent molecular level mixing that enhance the miscibility of different components with each other [62]. COA are prepared using a variety of techniques including milling, solvent evaporation, and quench cooling (Fig. 6(b)). An often-employed approach is the milling method that employs machine-driven activation to change substances into an amorphous state. Additionally, it proves high yield recovery and has minimal chemical deterioration. Despite these benefits, it is time-consuming, and labor-intensive, leaving crystalline impurities that could contaminate the combination. Moreover, the major scope of COA as the requirement of the drug and co-former should be soluble in the same solvent when solvent evaporation techniques are used, whereas stability at high temperature is required in case of milling and melt quenching. Hence, selection and optimization process are critical in fabricating COAs.
Cryogenic milling and BM are two dissimilar methods of milling. In contrast to the conventional milling method, cryo-milling (CM) is carried out under cryogenic conditions by introducing liquid nitrogen to milling bowls and grinding spheres [63]. Lobmann and colleagues conducted a milling investigation to develop stable, amorphous systems made of IND, carbamazepine (CBZ), and AAs, at a low temperature (6°C). The sum of amorphous and COAs binary amino acid mixture Tg demonstrated significant improvement, compared to individual drugs. Additionally, solid-state characterization using XRD revealed that blends were homogenous with an amorphous spectrum (Fig. 5). The COAs formulations exhibited good physical stability with a higher rate of dissolution than the corresponding crystalline and amorphous pure drugs [64].
X-ray diffraction spectrum co-amorphous formulations. Individual milled bulk drug (a), slight existence of crystalline form after cryo-milled for 120 min (b), co-amorphous IND mixture (c), co-amorphous CBZ mixture together with CBZ-ARG and CBZ-PHE (d), XRD spectrum of ball milled amorphous drug after 6 months. Reproduce with permission from [64] Copyright 2022, Elsevier
The amorphous system of poorly water-soluble drug hydrochlorothiazide (HDT) and atenolol developed using CM showed that the co-administration of atenolol and HDT offers therapeutic benefits. The results of infrared spectroscopy indicated that the COAs of atenolol-HDT samples with a molar ratio (1:1) exhibited exceptional physical stability explicated by the development of potent molecular connections between atenolol and HDT in a pharmacokinetic investigation, the HDT in the COAs form displayed a considerably higher intrinsic rate of dissolution as well as an improved bioavailability, compared with pure crystalline form, physical combination, and amorphous form, due to collaborative interaction of amorphized HDT and the water-soluble co-former atenolol [63].
Renuka and co-worker investigated the solubility profile of the COAs GLP-atorvastatin (ATS) binary mixture using CM for the treatment of hyperlipidemia and hyperglycemia simultaneously. The CM technique was used to develop the COAs of GLP-ATS in different combinations (1:1, 1:2, and 2:1). By using different tested ratios of the two drugs during CM, AMORP was found to be achievable. The results of the Tg and Raman spectrum suggested that no interaction between the drug with a significant improvement in dissolution profile [65].
The melt quenching technique is one of the common approaches to transform crystalline physical mixtures into COA materials, in addition to milling and solvent evaporation procedures. Quench cooling involves heating the drug mixture, followed by chilling the molten drug. In this method, the cooling period is held short, which prevents the molecules from rearranging into a crystalline lattice. This method is useful for fabricating small-quantity amorphous solid dispersion (ASDs) formulations, especially for thermostable drug molecules. However, quench cooling has significant limitations, such as the potential for chemical substance degradation and the requirement for exact freezing rate control [66]. Joana and coworkers revealed that AMORP of ranolazine through quench-cooling and cryo-milling demonstrated amorphous phases, with Tg values lower than room temperature. Moreover, two new forms of ranolazine (II and III) were generated within several hours after grinding and identified from the relaxation of the ranolazine amorphous phase produced by cryo-milling. Furthermore, the binary COA mixture of drug with tryptophan was developed, with higher Tg demonstrated improved kinetic stability preventing relaxation of the amorphous to crystalline phases for at least 60 days and improved aqueous solubility [67]. In another investigation, a COA mixture of CRD with maleic acid were developed with enhance solubility and stability. The study demonstrated disappearance of carboxy group peak in co-amorphous system (2:1) and endothermic peaks associated with drug and amino acid with 3-fold increase in solubility profile. Moreover, the stability study resulted with no clear diffraction peaks originating from crystals after three months of storage [68].
Recently, COA were prepared effectively employing the supercritical fluid method to fabricate PASDs. A substance is referred to as a supercritical fluid when the temperature and pressure reach above its critical point. The carbon dioxide in its supercritical form is mostly used as an antisolvent for COA particle precipitation or as a solvent to dissolve co-formers and drugs [69]. The binary systems of l-Arg/glimepiride fabricated employing the supercritical fluid method showed a pure COA mixture with high content homogeneity [70].
Conversely, the l-Arg/glimepiride (LAG) combination developed by physical mixing and solvent evaporation made up of a crystalline eutectic mixture indicated insignificant COAs. The results of Fourier-transform infrared spectroscopy (FTIR), nuclear magnetic resonance (NMR), and Tg showed significant intermolecular interactions between l-Arg and glimepiride (GLB) in the COAs of LAG mixture system. Moreover, the measurement of contact angle, solubility test, and dissolution displayed that the LAG COAs mixture formulation’s improved GLB dissolution rate. Additionally, LAG COAs mixture generated via the supercritical antisolvent technique greatly improved the therapeutic efficacy of GLB through the study of its pharmacodynamic hypoglycemic effect [69].
Mesoporous Particles-Based Amorphization
Mesoporous particles have recently gained considerable attention as s stabilizers for amorphous formulations. Mesoporous particles, featuring pore diameters between 2 and 50 nm, have emerged as a prominent choice for stabilizing amorphous formulations. In contrast to microporous (sub-2 nm pores) and macroporous (above 50 nm pores) particles, their unique size range offers distinct advantages in enhancing formulation stability [58]. Several mesoporous silica nanoparticles available in the market have different properties such as their pore size, pore volume, and surface area. Their large pore volume and surface area allow to incorporate large quantity of therapeutic agent. In fact, their characteristic pore structure has been reported to entrap drug molecules in an amorphous state and prevent the drug from recrystallization, thereby acting as the stabilizer for amorphous formulations. In addition, due to presence of silanol groups on the surface of the mesoporous silica, drug-silica interactions may occur through hydrogen bonding, thereby leading to the AMORP of the drug molecules [58]. The pharmaceutical industry predominantly employs mesoporous materials including MCM-41, Neusilin® UFL2, Fujicalin®, and FujisilTM-F, which are primarily synthesized through electrochemical methods such as stain etching and anodization [71]. In 2001, valet-Ragi anc co-worker suggested the use of mesoporous silica MCM-41 in drug delivery that can carry drug molecules in the pore structure [72]. Whereas in another investigation, liquid carbon dioxide effectively loaded meropenem into surface-modified mesoporous silica MCM-41, achieving an impressive drug loading capacity exceeding 25% [73]. A study designed to load celecoxib mesoporous tablet formulation demonstrated improved loading capacity, 3-fold drug solubility, and dissolution profile; however, both these were significantly affected drug loading method and drug-to-mesoporous ratios [38]. In another investigation fluid bed hot-melt impregnation has proven highly effective for AMORP of drugs within mesoporous particles, offering a solvent-free approach with demonstrated efficiency. This innovative method holds significant promise in pharmaceutical formulation, ensuring both drug efficacy and safety through unique amorphous structures [74].
Hot-Melt Extrusion-Based Amorphization
In recent decades, hot-melt extrusion technology as thermal processing has attracted a great deal of attention from the pharmaceutical industry as promising solvent-free continuous process with a wide range of applications in drug development. In a single continuous process called melt extrusion, materials are melted or softened at high temperatures before being cooled down to produce a hardened phase. The continuous processing and versatility to provide improved process control and promote the scalability of this technology that means it can be used for expanded pharmaceutical development alongside other emerging technologies and applications. Hot-melt extrusion has proven highly efficient in producing an amorphous blend of curcumin and piperine, enhancing their bioavailability and biological efficacy [75]. It was first used in the plastics sector and later introduced to pharmaceuticals. A hot melt extruder consists of a temperature-controlled barrel and spinning screws to mix and feed ingredients through a die, suitable for developing amorphous SDs [76]. Lenz and coworkers studied the viability of hot melt extrusion for producing COAs of IND-AAs in combinations continuously. A melting-solvent extrusion procedure was used to fabricate COAs of IND-Arg with and without polymer. The formulations consisting of IND along with polymer and Arg exhibited a surge in dissolution behavior compared to formulations containing only Arg and polymer [77].
The feasibility of employing hot melt extrusion for the continuous production of co-amorphous drug-amino acid formulations has been thoroughly investigated [77]. Shi and co-worker employed hot melt extrusion technology to enhance the dissolution and solubility of amorphous self-micellizing solid dispersions, featuring BCS II drugs, namely indomethacin and fenofibrate. Their study also investigated the “spring-parachute” processes, illustrating hot melt extrusion possess potential for optimizing drug delivery with improved bioavailability and effectiveness [78].
In Situ Amorphization
The idea of in situ AMORP has attracted significant attention among numerous researchers looking for novel solutions to the problem of physical stability presented by amorphous formulations. It was not initially thought of as a novel and alternative method of fabricating amorphous systems, nonetheless rather as an unwanted abnormality or deviation in the production process [79]. The ability to avoid the issue of physical stability and the simplification of the preparation stage are two obvious advantages of an in situ AMORP strategy. The three methods namely solvent-assisted method, laser irradiation method, and microwave irradiation are employed for in situ AMORP [80]. The in situ AMORP induced by microwave radiation was proposed as a promising solution to amorphous drugs which are thermodynamically unstable. Before oral administration, the originally loaded crystalline drugs are in situ amorphized within the final dose form using a standard microwave [81].
An effort for in situ AMORP by fabricating the tablet was carried out by immersing it in an aqueous buffer solution (pH 6.8) with Eudragit in different ratios was investigated. The state of AMORP with the disappearance of crystalline peaks is confirmed by X-ray powder diffraction (XRPD) and thermal analysis [82]. Similarly, the feasibility of in situ co-amorphization of Arg and IND or furosemide (FUR) along with Eudragit L within the tablet was proved [83]. Another COAs formulation utilizing CRD and aspartic acid was effectively formulated by Petry and colleagues further demonstrating the usefulness of in situ co-amorphization, indicating the development of an in vivo AMORP paradigm where drugs amorphized in the gastrointestinal tract after ingestion [84].
Microwave-induced in situ amorphization is another novel technique for preparing amorphous solid dispersion to address the challenges of its long-term physical stability and downstream processing. Wei and colleague investigated the feasibility and mechanism of utilizing the non-ionic surfactants (kollisolv P124, kolliphor RH140, d-α-tocopheryl polyethylene glycol succinate, tween 60, tween 65, tween 80, and tween 85) as plasticizers to facilitate microwave-induced in situ amorphization. Investigator indicated that due to kolliphor RH40 excellent dielectric heating ability, plasticizing effect, and solubilizing effect facilitated the amorphization at 1.5 (w/w) of the plasticizer/polymer ratio with a fast in vitro dissolution [85].
As the microwave irradiation insisted technique necessitate the involvement of a microwave absorbing plasticizer that carries the risk of causing hydrolysis of the drug, there seemed to be a need for a new method to fabricate amorphous systems in situ. In a recent study by Hempel and co-worker, laser irradiation was selected as an alternative heating source with photothermal plasmonic NPs as the laser absorbing component. The plasmonic NPs are stimuli-responsive materials that can be activated. By laser at their plasmonic resonance frequency and release vibrational energy in the form of heat, compacts containing the physical mixture of celecoxib, PVP, and plasmonic silver nanoaggregates were prepared with different drug loads (30, 50 wt%) and plasmonic concentrations (0.1, 0.25 wt%). These tablets were treated with near-IR laser radiation at varying intensities and exposure time. It was demonstrated that with a higher laser intensity and plasmonic content, the onset and rate of AMORP was faster due to the shorter exposure required to reach the temperature threshold, above which the on-demand AMORP of drugs was shown to take place. Moreover, to achieve full AMORP in the compacts with a higher drug content, a longer duration of laser irradiation was needed, compared to the compacts with lower drug content at the identical tablet thickness [86].
In most scenarios, amorphous SDs are produced at higher temperature or solvent-assisted environment using various process. Hot melt extrusion and subsequent fused deposition modeling-based three-dimensional printing offer great potential opportunities in the formulation development and production of customized oral dosage forms with poorly soluble drugs. However, thermal stress within this process can be challenging for thermo-sensitive drugs. Lena and co-worker investigated the degradation and the solid state of the thermo-sensitive and poorly soluble drug escitalopram oxalate during the two heat-intensive processes during hot-melt extrusion and fused deposition modeling-based three-dimensional printing in presence of hydroxypropyl methyl cellulose and butylated methacrylate copolymer. Thermal and x-ray diffraction analysis indicated that hydroxypropyl methyl cellulose was able to produce amorphous in both hot-melt extrusion and fused deposition modeling process, whereas in the presence of butylated methacrylate during hot-melt extrusion, degradation of the drug was observe due to conversion of active moiety into the R-enantiomer. Moreover, the drug release from extrudates and tablets was slower but still qualified as immediate drug release than those tested from the commercial product Cipralex® [87].
The first amorphization attempt within the tablet was performed through immersion in an aqueous buffer (pH 6.8) [88]. In an attempt to improve solubility using solvent assisted, Doreth and co-worker plasticized Eudragit E at pH 6.8 and dissolved at pH 4.1 as carrier to release amorphous drug indicating that this on-demand amorphization could be done through simply placing the tablet in a glass of water for a few mint [89].
Characterization Techniques for Amorphized Materials
It is of utmost importance that the formulations used for ASDs are characterized in depth due to the nature of the ASDs and the inherent risks of recrystallization. To adhere to the quality by-design principles, it is essential to have a deep understanding of the processes occurring at the molecular level. There is no specific characterization technique that can give all the information needed, and a combination of complementary methods is often needed to get the full picture. However, some specific characterization techniques for COAs formulations such as XRD, DSC, TGA, FTIR, and chromatography analysis followed by dissolution or solubility testing have been briefly discussed (Fig. 6).
Different characterization techniques executed for co-amorphous formulations. Adapted with permission from [9] Copyright 2022, Elsevier
X-ray powder diffraction (XRD) is widely used in material science, chemistry, and geology to study the structure of materials at the atomic level. It is particularly useful for analyzing crystalline solids, including minerals, metals, ceramics, and polymers. XRD is a non-destructive technique used to determine the crystal structure of materials, which involves passing a beam of an x-ray through powdered samples and measuring the diffraction pattern produced as the x-ray interacts with the crystal lattice of the sample. The diffraction pattern is unique to the crystal structure of the material and can be used to identify and quantify the phases present in the samples [90]. As further investigations demonstrate the powder diffraction method’s effectiveness in different fields, it has attracted significant interest. zeolites, tiny chemical molecules, and more recently biological macromolecules have all shown them to be very useful in defining their structures. Due to the utilization for high-resolution synchrotron data and modern analytical techniques, significant improvements have been discovered in XRD effectiveness. This has broadened the number of amino acids that may be structurally examined, making XRD a potent and useful tool in addition to other traditional methods. While dealing with poly-crystalline samples, which have certain disadvantages, such as the possibility for lower or medium-resolution diffraction data (range from 3 to 10), their benefits over single crystals lie in their ease of creation and simplicity. Examining low-quality crystals is made possible by XRD technologies, opening the door to crucial uses including amino acid polymorph screening and time-resolved research. Furthermore, XRD investigations offer important preliminary structural understandings that are essential for further structural characterization. A systematic and well-defined procedure employed to deploy refined structures of a specific amino acid is far more beneficial. However, it is important to acknowledge that peak overlap is a common issue encountered in XRD data. To address this problem, multiple data sets with different cell characteristics or preferred orientations can be combined. This approach allows to draw more accurate identification of individual reflections within overlapping peak clusters. Notably, the PRODD refining software has been enhanced to support a multi-pattern overlay fit, which facilitates a more precise extraction of intensity information. Overall, the ongoing breakthroughs in powder diffraction, together with improvements in XRD methods and data interpretation, have further enhanced its position as an important approach for structural research in a variety of scientific fields [91]. Corbia and coworkers included the PXRD data for a fascinating palladium (II) amino acid complex that showed a distinct PdC12H20N2O4S2 composition. Using cutting-edge PXRD technology, their investigation illuminated the structural properties of this new molecule [92]. Additionally, Vijayan and his coworker used XRD to investigate a single l-alanine crystal for unrevealing the quality using a Rich Seifert PXRD with a scan speed of 1°/min to examine the crystal’s characteristics [93].
Differential scanning calorimetry [94] is a highly sensitive technique that detects small changes in the heat flow of a sample, making it a powerful tool for the analysis of complex materials. DSC is a thermal analysis technique used to measure the heat flow or heat capacity of a sample as a function of temperature or time. DSC measures the difference in heat flow between a sample and a reference material as they are subjected to a controlled temperature program. DSC is used to study an inclusive range of materials including polymers, pharmaceuticals, foods, and metals for characterizing the thermal behavior of tested materials such as changes in their phase transitions or chemical reactions that occur with temperature [95]. Furthermore, the thermal property of materials and chemistry are also analyzed using TGA for an inclusive range of materials, including polymers, ceramics, metals, and pharmaceuticals. Thermal analytical approaches interpret molecular processes that take place in SDs, such as glass transition, crystallization, polymorphic transition, molecular mobility, structural relaxation, and miscibility between drugs and polymers. It is particularly used to analyze thermal stability, thermal decomposition, and the presence of volatile components in materials. TGA is simultaneously used with other techniques such as DSC and DTA to collect compressive results. Moreover, differential mechanical thermal analysis can provide details on the binary or ternary miscibility, relaxation transitions, and viscoelastic properties of polymers [95].
Pfeil investigated a comprehensive analysis of the changes in free energy, enthalpy, and heat capacity associated with protein unfolding. Among the proteins studied were streptomyces subtilisin inhibitor (SSI), known to exist as a dimer at room temperature. DSC analysis revealed that SSI undergoes dissociative unfolding, where the dimeric structure transitions to a monomeric state. The melting temperature (Tm) values obtained from the DSC curves increased with higher protein concentrations, and the curves exhibited asymmetry. Quantitative analysis of the DSC curves confirmed that the denaturation protein suggests that the natural dimeric state of SSI persists up to temperatures of approximately 80°C [96]. Furthermore, Sabulal and his group investigated the impact of pH 2 on the heat denaturation of lysozyme in the presence of aqueous solutions containing different amino acids, such as glycine, alanine, leucine, Ly, diglycine, triglycine, tetraglycine, pentaglycine, glycyl leucine, and glycyl-glycyl leucine. Moreover, the effect of lysozyme at various temperatures and how denaturation underwent in the presence of tested amino acids aqueous solutions were quantified using high-sensitivity DSC. This research illuminated the impact of various amino acids on the denaturation process of lysozyme as well as its thermal stability and behavior in acidic circumstances [97].
Fourier-transform infrared spectroscopy (FTIR) in combination with attenuated total reflectance and/or diffuse reflectance and Raman spectroscopy are two efficient techniques in vibrational spectroscopy. These techniques are used in many pharmaceutical applications, including the identification of polymorphs, phase transitions, recrystallization stability, evaluation of various solid dispersion preparation processes, phase separation, and the nature and extent of drug-polymer interactions. Moreover, these techniques provide information about the structure and molecular conformation of solids through the study of band vibrations [98]. Additionally, Raman spectroscopy is a powerful light-scattering technique used to diagnose the internal structure of molecules and crystals. Insight into crystal packing can be also gained by studying the low-energy lattice vibrations associated with different crystal packing arrangements [99]. Furuyama and co-workers used Raman spectroscopy as a mapping technique to distinguish between crystalline and amorphous forms of troglitazone in SDs [100]. In addition, Sinclair and colleagues adopted FT-Raman spectroscopy to measure the recrystallization kinetics of amorphous SDs of the drug [101].
The information from infrared spectroscopy is well recognized and includes biological systems. Thus, it has developed into a crucial tool for analyzing amino acid structure, comprehending the molecular processes behind amino acid reactions, and investigating amino acid folding, unfolding, and misfolding. Therefore, biological systems that are larger than amino acid can benefit from the wealth of information found in the infrared spectrum. The capacity to distinguish between and categorize various types of microbes based on their infrared spectra is another remarkable use of this. In addition to these skills, infrared spectroscopy has several other benefits including investigating both tiny soluble proteins and big membrane proteins, giving it a broad variety of applications [102].
The polarized Raman and infrared spectra with vibrational properties of crystalline amino acid l-glutamic acid were investigated by Dhamelincourt and coworkers. The investigation resulted in important information on the vibrations of molecules and structural characteristics of l-glutamic acid revealing an extra significance of amino acids and how they behave spectroscopically [103]. Further, to precisely identify 16 distinct free amino acids such as aspartic acid, threonine, serine, glutamic acid, proline, glycine, alanine, valine, methionine, isoleucine, leucine, tyrosine, phenylalanine, Ly, histidine, etc. in Chinese rice wine using near-infrared (NIR) spectroscopy was investigated. The investigation indicated for the first time, NIR spectroscopy in transmission mode was utilized by the researchers to examine different samples of aged rice wines. The results indicated that NIR spectroscopy could be a potential alternative with the capability to replace complicated analytical processes with a quick, simple, and creative method for precisely determining the concentrations of basic amino acids [104].
Nuclear magnetic resonance (NMR) is also another non-destructive technique that provides qualitative and quantitative information about solid amorphous dispersions with detailed 1D and 2D structural information. Additionally, information on the phase composition and molecular mobility of polymers in SDs is obtained from 1H transverse magnetization relaxation T2 measurements. 13C cross-polarization magic-angle spinning NMR experiments were used to study the recrystallization of amorphous troglitazone in SDs prepared by different methods with no difference in temperature and relative humidity [105]. Shi and a coworker analyzed acid-hydrolyzed material to ascertain the amino acid content of Nephila clavipes dragline silk fiber using 1H NMR. Investigation indicated that Nephila clavipes dragline silk mostly includes amino acids such as Gly (43.0 ± 0.6%), Ala (29.3 ± 0.2%), Glx (9.1 ± 0.1%), leucine (Leu) (4.0 ± 0.1%), Tyr (3.3 ± 0.1%), Ser (3.4 ± 0.2%), Pro (2.7 ± 0.1%), Arg (2.1 ± 0.1%), Asx (1.07 ± 0.05%), Val (0.96 ± 0.05%), Thr (0.48 ± 0.03%), Ile (0.28 0.03%), and Phe (0.35 0.03%) with great resolution, compared to conventional chromatography-based amino acid analysis methods indicating potential in quantifying the varied class of amino acids [106]. In another investigation, 1H-NMR spectra were recorded at 600 MHz on trichloroacetic acid extracts of fish flesh stored over 15 days under different storage conditions to differentiate the amino acid concentration. The amino acid trends were related to the different storage temperatures from day 4 onwards [107]. Moreover, the simultaneous estimation of betaine, citric acid, threonine, alanine, and proline in different Lycii Fructus samples using strong cation exchange solid phase extraction (SPE) and 1H-NMR spectroscopy was accomplished. The results revealed a trustworthy method for the analysis of amino acid with the benefit of simultaneous measurement of numerous analytes [108].
Water vapor sorption has been another widely employed technique to study the behavior of amorphous and crystalline materials in the presence of moisture. Moisture sorption data are interpreted using theoretical approaches such as predicting the additivity of the hygroscopic isotherms of individual components or using the FH ternary interaction theory, which supplies insight into drug-polymer-water interactions. In combination with other techniques such as DSC, FTIR, and NMR, molecular level attributes such as surface properties, degree of AMORP, phase transition, glass transition, and critical relative humidity for crystallization provides a variety of information about the physical stability of freshly prepared and aged material. Dynamic vapor sorption coupled with near-infrared spectroscopy presents insight into the desorption behavior of amorphous materials before and during crystallization as a function of solid-state nuclear magnetic resonance (ssNMR).
Analyzing the surface characteristics of amorphous SDs are studied using inverse gas chromatography, which is still a relatively new technology used to research amorphous transition or recrystallization, molecular relaxation, and molecular mobility. This helps in identifying different amorphous SDs, manufactured using the same or different techniques. Investigating the increased molecular mobility on the material's surface compared to its bulk can also shed light on how amorphous pharmaceuticals interact with moisture and recrystallize. Hasegawa and coworkers investigated structural relations at the surface of SDs using inverse gas chromatography and discovered that structural relaxation happens more quickly at the surface than in the bulk due to the increased molecular mobility there. Predictions about the physical stability of amorphous products can be made by studying crystallization kinetics at the surface of SDs [9, 59].
Further, the purity, solubility, and percentage of drug loss during AMORP are characterized by chromatography analytical techniques, which are used to separate and analyze components of a COA mixture. Chromatography analysis works on the principle of differential partitioning of components in a mixture between two phases, one stationary and the other mobile phases, which can be either gas or liquid. Morphological analysis for COAs is investigated to identify the surface modification, especially AMORP performed by spray-drying techniques using scan electron microscopy (SEM). The surface characterization of native and modified drugs with amino acids is studied for the transformation of crystalline form to an amorphous state using SEM or field emission scan electron microscopy (FESEM). Furthermore, the improvement in solubility, dissolution, and stability is also of importance in the characterization of amorphized drugs. For predication of the component’s miscibility, the Flory-Huggins interaction parameter (χ) [109] and the Hansen solubility parameter (δ) [110] have been employed. For thermally stable compounds, miscibility of components in the melted state can be easily determined from a phase diagram. Marsac and colleague used a melting point depression approach, whereby miscible systems showed melting point depression of the drug, while immiscible or partially miscible systems showed little or no depression. More research using larger data sets and advanced statistical tools is necessary in order to develop systematic approaches for the selection of co-formers [111]. Summarized characterization techniques used for AMORP are presented in Fig. 7.
Application of Amorphization in Improving the Bioavailability of Low Soluble Drugs
The COAs consisting of drugs along with AAs are becoming increasingly popular for enhancing the rate of the dissolution of poorly water-miscible drugs. Based on their component combinations, COAs are classified into two primary types such as combination mixtures of drug-drug and drug-excipient. In a binary amorphous system known as a drug-drug COAs system in which drugs that are relevant from a pharmacological perspective are combined and kept stable in the amorphous state for a prolonged time. In combinations of a drug with an excipient, excipients with tiny molecular weights, also known as co-formers in this discipline, function as stabilizers in co-amorphization to increase the dissolution rate and physical stability of drugs [112].
The Arg and P-glycoprotein inhibitors verapamil hydrochloride, piperine, and quercetin were employed as co-formers for COAs combinations of FUR, a BCS class IV medication. The SD technique was used to fabricate 1:1 and 1:2 molar ratios of FUR combinations with verapamil hydrochloride, piperine, and quercetin, while solvent evaporation was used to formulate mixtures with Arg. The results of stability experiments conducted under various storage circumstances showed single glass transition values for the mixtures assessed using XRD, FTIR, and DSC. Moreover, the results indicated the development of intermolecular interactions between the components, particularly salt formation between FUR and Arg. Additionally, diffusion and dissolution studies demonstrated that COAs mixtures of FUR:Arg were capable of enhancing the permeation and dissolution of FUR [113]. Several studies indicated that the BM was used to achieve the co-amorphization of several forms of crystalline Trph [114, 115]. Trph, a chiral AAs, and its enantiomers were used as a model system to develop a novel method for enhancing the aqueous solubility of poorly water-soluble substances. Solid state study revealed significant differences between two Trph enantiomers, individually the Trph racemate, and an equimolar physical mixture of d- and l-Trph in terms of physical stability and AMORP tendency. It was observed that plain d- and l-Trph were amorphous after 6 h of BM; however, the Trph racemate and Trph conglomerate were converted to amorphous within 60 and 90 min of BM, respectively. The physical stability of Trph conglomerate/COAs was observed for one month with a reduction in physical stability in an ambient environment. However, the intrinsic dissolution rate of the amorphous Trph racemate and conglomerate showed insignificant effects on corresponding crystalline forms [114]. Wu and co-worker investigated the co-amorphization of mebendazole (MBD) with different dipeptides including proline-Trph, PHEL-Trph, Trph-PHEL, histidine-glycine, and aspartic acid-tyrosine. After 30 min of BM, different MBD-dipeptide complexes indicated the formation of an amorphous form. However, AMORP did not apply to individual AAs or physical mixtures of the AAs composing the dipeptides. Moreover, the dissolution tests revealed that the rate of dissolution was much higher for most of the COAs, compared to amorphous and crystalline MDB. Additionally, there was no obvious pattern for the drug dissolution enhancement observed between the several COAs drug dipeptide complexes. Besides this, in the stability investigation, COAs dipeptide systems with MBD demonstrated greater physical stability than the amorphous form of MBD [116] (Fig. 8).
Scan electron microscopy images of native micronized IND (a), spray-dried IND-Arg (b), and precipitates obtained after the dissolution of SD IND-Arg physical mixture compacted tablet (c), SD IND-Arg compacted tablet (d), and SD IND-Arg (e). Adopted with permission from [117] under Copyright Clearance Center Rights Link of Elsevier
The study involved how different AAs affected the stability of spray-dried amorphous powders for inhalation, utilizing simvastatin as a model agent. Lenz and colleagues fabricated the SD of IND-Arg and investigated the effect of compression pressure on tablet properties, physical stability, and dissolution profiles under non-sink conditions. A broad range of compaction pressure resulted in different porosities and tensile strengths with supersaturation of in situ amorphized IND in the presence of Arg. Moreover, the SEM images showed a typical prism and plate-like shape of native micronized IND, which was transformed into needle shape crystals for SD of IND-Arg. However, the crystallized IND was finally dispersed after the dissolution of the compact tablet of SD IND-Arg, which was confirmed by thermal analysis and XPRD of precipitates (Fig. 10) [117]. In another study, the stabilizing effect of Leu, Trph, and Ly were investigated using the SD of SIM for nasal drug delivery. The morphological analysis using SEM and AMORP quantification using XRD indicated the transformation of crystalline Leu to amorphous after spray drying with enrichment of SIM surface by Leu. In contrast, an even distribution of Trph over SIM was observed with significant stability for 8 months in the desiccator at 12% RH. Spray-drying of Leu alone resulted in wrinkled and fragmented particles; however, Trph and Ly appeared smooth with spherical [118]. Moreover, SEM images showed irregular shapes that tend to form aggregates (Fig. 9). Spray-drying of SIM with different AAs was aggregated, which become spherical with optimization of spray drying feed rate (Fig. 8) [118]. HDT is a first-line drug in the management of hypertension endured from low oral bioavailability due to poor solubility and permeability. Solid dispersion using kneading techniques of HDT with egg white protein showed significant improvement in the solubility (32-fold) and ex vivo permeability over native HDT. The microphotograph of crystalline HDT demonstrated alteration to small non-uniform, and amorphous particles with porous surfaces. Additionally, the optimized composition showed fused particles with characteristics of large HDT particles suggesting the formation complex of drug and protein [119] (Fig. 10).
Scan electron microscopy images of spray-dried alone simvastatin (a), leucine (b), lysine (c), trypsin (d), spray-dried simvastatin with leucine (e), spray-dried simvastatin with lysine (f), spray dried simvastatin trypsin. Adopted with permission from [118] under Copyright Clearance Center Rights Link of Elsevier
Scan electron microscopy image of pure HDT (a), egg white protein-based solid dispersion (b), and optimized solid dispersion of egg white protein with HDT. Adopted with permission from [119] under Springer Nature Switzerland AG. Part of Springer Nature (2023), respectively
In another study, ASDs prepared by mixing whey protein isolate and whey protein hydro-leucine with poorly soluble drugs FUR, CRD, and IND using the vibrational BM technique demonstrated improvement in solid-state properties, rate of dissolution, and solid dispersion physical stability. Moreover, as compared to the corresponding pure crystalline and amorphous drugs, ASDs exhibit a noticeably higher Tg, quicker rate of dissolution, and superior apparent solubility. The inclusion of whey proteins improved the drug’s saturation solubility and the tested ASDs demonstrated supersaturation by reaching superior drug concentrations than corresponding saturation solubility. In addition, ASDs with IND were physiologically stable for approximately 27 months, while ASDs with FUR or CRD were stable for around 8 months and 17 months, respectively during storage. However, pure amorphous drugs recrystallized in less than a week with instability suggesting that the whey protein isolate and whey protein hydrolysate are promising stabilizers to fabricate ASDs [120].
To gain a deeper understanding of the structure of materials generated from corn flour via thermomechanical processing, the specific hydrolytic activity of proteolytic and amylolytic enzymes was utilized [121]. Adhikari’s co-worker examined the impact of co-amorphization on the aerosolization of kanamycin (KAN), an aminoglycoside antibiotic with various AAs such as Phel, Trph, Met, and Val by employing a co-spray drying technique. The thermal technique authenticated co-amorphization with enhanced fine particle fraction, except for the formulation of KAN-Val. The highest fine particle fraction of 84% was achieved by KAN-Met formulation. The COAs formulations displayed stable aerosolization and were significantly stable for 28 days. The combination of KAN-Met showed excellent aerosolization stability at a relative humidity of 53%, despite Met changing into its crystalline form, without any loss in fine particle fraction [122] implying a high dose of KAN could be formulated in powder formulation via COAs mixtures of KAN with Met, although more research is needed to determine the underlying process. FUR is often prescribed to treat edema and congestive heart failure. Although there are several issues with bioavailability due to poor solubility and erratic GI absorption, the fabrication of FUR/AAs COAs is thought to be a solution. The COAs of FUR with l-Arg, l-cysteine, l-Trph, l-Phel, and l-Val were successfully fabricated employing the BM method. The protonated interaction between the AAs carboxyl group and the secondary amino group in FUR improves compatibility between FUR and AAs. Due to the advantageous aromatic stacking in the FUR-Phel and FUR-Trph systems, the COAs generated between FUR and Phel/Trph have excellent physical stability compared to other FUR-AAs-COAs. The results of powder dissolution for COAs FUR/Phel and FUR/Trp showed that stable COAs had exceptional dissolution advantages, with two times enhancement in the rate of dissolution over pure FUR [123].
Experiments on prodrugs of AAs have shown enhancing many therapeutical parameters such as poor permeability and solubility of low oral bioavailable drugs. By employing AAs prodrugs concept in delivering drugs via the intravenous route, sustained release and enhanced metabolic stability of different pharmaceutical substances have been accomplished [124]. Secondly, only a few drugs for central nervous disease can cross the blood-brain barrier; however, pro-moieties of AAs had been reported as an imperative tool to improve the blood-barrier brain transposition. Moreover, these pro-moieties are safer pharmacological agents that do not augment the toxicity of the compound and conversely reduce the adverse effects of drugs, particularly those employed as antitumor agents. Pharmaceutical industries face a tough task in delivering a drug to different parts of the eyes like the sclera, retina, vitreous humor, and choroid. Regular administration of the drug or surgery is required in such kinds of infections; therefore, it is so crucial to develop strategies to amplify the permeation of l-valine ester into corneal or retinal tissues [125]. Katragadda and colleagues studied the derivatization of acyclovir (ACV) with AAs and exhibited a 2-fold enhancement in ocular bioavailability after topical application of l-valyl and l-serine esters of ACV [126]. Ganciclovir has poor aqueous solubility is another such example it is employed to treat cytomegalovirus retinitis [127]. Furthermore, Ojarinta and co-workers investigated the ability of Arg, Phel, and Trph to prolong the supersaturation of either free or COAs IND with AAs in aqueous and relevant conditions. The dissolution and precipitation test for COAs of AAS with IND in buffer solution and feed and fasted stimulated fluids indicated supersaturation stability and insignificant precipitation of AAs. The solid-state investigation of these precipitates indicated that they consisted of crystalline IND-Arg with residual crystalline materials. Moreover, regardless of processing steps such as drying and medium used, COAs of IND with AAs showed similar FTIR spectra; however, differed from the spectra of crystalline AAs, micronized IND, and COAs of IND (Fig. 11(a and b)). The appearance of peaks ranging in 1500–1750 cm−1 was observed in COAs of IND with AAs than those of free IND suggesting that the COOH-groups of IND ionized [128]. The solid-state characterization, stability, and dissolution efficacy of COAs mixture of drug with AAS (Trph, Arg, Pro) using BM demonstrated significant improvement, compared to native free drug. Pro acted as a stabilizer, while Trph improved the molecular interactions in the form of hydrogen bonds between AAs and drugs. The overall AMORP was quantified by the formation of a halo in the XRD diffractogram as presented in Fig. 11(c and d). The XRD spectra showed that 90 min of BM was able to transform the mixture into amorphous form (Fig. 11(c)); however, milling of drug till 6 h with tryptophan demonstrated significant formation of amorphous mixture (Fig. 11(d)). Additionally, the results indicated that alone Pro was able to transform the mixture into amorphous with solid-state stability. Moreover, the milling of ternary mixture drug-Trph-Pro, resulted in excellent amorphous blend [129]. Furthermore, two COAs drug-AAs systems, such as IND-Trph and FUR-Trph, were analyzed toward their ease of AMORP using BM for drugs with or without AAs. The solid-state NMR (13C) of BM drug with AAs were assessed for line width and shape changes from the spectra obtained. The spectra indicated asymmetrical broadening of the overall spectrum in an amorphous state due to the inhomogeneous distribution of isotropic chemical shifts resulting in a static distribution of bond or torsion angles (Fig. 12). The projection method such as principal component analysis results for varying milling times for IND, FUR, Trp, IND-Trp, and FUR-Trp demonstrated a quick and high degree of AMORP with π-interaction involving the carbonyl of the drug and aromatic IND, FUR, and Trp [110].
Infrared spectra of amorphous IND, Arg-IND salt, a co-amorphous mixture of Arg and IND, physical mixture of Arg and IND, crystalline Arg, micronized IND, and either dried or undried Arg-IND precipitates, from two different media (a and b). Adopted with permission from [130] under Copyright Clearance Center Rights Link of Elsevier. X-ray diffractogram of crystalline NAP, BM NAP, BM NAP-Trp, BM NAP-Pro, co-amorphous TRP-Pro, and co-amorphous NAP-Trp-Pro (c and d). Adopted with permission from [129] under open access Creative Commons CC BY 4.0 license
NMR (C13) spectra of alone IND, FUR, and tryptophan BM for different time intervals (a). NMR (C13) spectra of IND-tryptophan and FUR-tryptophan mixture BM for different time intervals (b). NMR (C13) spectrum of amorphous IND, tryptophan, co-amorphous IND-tryptophan, amorphous IND and tryptophan, and amorphous drug-tryptophan subtracted from co-amorphous IND-tryptophan (c). The left side figure stands for IND and the right side represents FUR. Adopted with permission from [110] under Copyright Clearance Center Rights Link of American Chemical Society
In another study, the molecular interaction of IND and FUR with different AAs using the BM process. Interaction reactions were studied using XRD, DSC, FTIR, and NMR. The FTIR interaction study indicated the formation of heterodimers via intermolecular hydrogen bonding in COAs mixture proving high stability for samples with equal molar ratio (Fig. 13(a)). Moreover, NMR spectra of COAs IND-Trp and FUR-Trp showed interaction via deviation from Gordon-Taylor equation at 50% molar ratio (Fig. 13(b)) with a large part of resonances that relates to the aromatic resonances in the spectral region 100–150 ppm. Likewise, salt formation during AMORP is another predicted process demonstrating the resonance of sensitive carboxylate of ionized and non-ionized states at different ppm [130]. Ordoubadi and co-workers developed carrier-free spray-dried dispersion of leucine for pulmonary delivery, with the stability of more than 8 months. Generally, alone Leu through stability develops a small fibre due to its presence in higher energy states during crystalline polymorphs [131]. Similarly, zein-coated polycaprolactone nanofibers were investigated for antimicrobial properties, which showed enhanced mechanical strength, reduced diameter (Fig. 14(a)), and water stability with sustained release of tetracycline hydrochloride. Furthermore, the in vitro cell proliferation assay demonstrated adhesion of fibroblast cells (Fig. 14(b)) over nanofibers with rapid closures of the physical scratch (Fig. 13(c)), compared with pristine polycaprolactone nanofibers [132]. Yannick and co-worker studied the assessment of most suitable low-molecular weight excipients for stabilization of amorphous drug. The co-amorphous blends were prepared by cryo-milling with l-leucine, l-norieucine, l-tert-leucine, and l-arginine to understand the stabilization mechanism. Among tested amino acids, l-arginine demonstrated possible stiffening of the H-bond that drastically improved the stability of indomethacin [133]. In another study, co-amorphization of flubendazole with l-phenylalanine and l-trytophan in 1:1 ratio demonstrated significant improvement of physical stability and dissolution might be due to suppression of crystallization tendency [134]. Hoda EI and May fabricated the spray-dried mixture of tadalfil with histidine, valine, alanine, and arginine. The spray dried mixture showed improvement in solubility (15.12 ± 0.13 μg/mL) and dissolution in 6 h with amendment pharmacokinetic parameter when tested on animals, compared with pure crystalline drug [135]. The summary of recently used amino acid in AMORP is presented in Table I.
Infrared spectra of IND-Arg co-amorphous mixture FUR-Arg mixture with a different molar ratio of Arg (a). ssNMR spectra of BM mixtures at varying molar ratios and the PCA loadings (b). The left side image represents IND-Arg, and the right side of the image represents FUR-Arg of different purity. Adopted with permission from [130] under Copyright Clearance Center Rights Link of Elsevier
Scan electron microscopy of nanofiber indicating surface morphology and diameter of zein-coated PCL at different ratios prepared using Electrospun (a), adhesion of fibroblast cells on zein-coated PCL nanofibers (b), and fibroblast cell migration in presence of zein coated PCL nanofibers (c). Adopted with permission from [132] under Copyright Clearance Center Rights Link of Elsevier
After administration, absorption and permeability of the drug depend on the solubility and dissolution rate, as well as the gastrointestinal permeability of the drug. In the case of poorly soluble drugs, a low intraluminal concentration result in inadequate absorption. Amorphization is one of the common approaches to improve the solubility and dissolution of BCS class II and IV drugs. Several polymers have been reported that act as stabilizers for the amorphous form of the drugs by diminishing the molecular mobility of this drug, therefore, inhibiting the nucleation and crystal growth. A higher concentration of dissolved drug can be obtained via several approaches including developing a supersaturation of a drug in its amorphous form. Lenz and coworkers performed an in vitro dissolution experiment under non-sink conditions (phosphate buffer of pH 4.5) to investigate the concentration-time profile, Cmax, and AUC. The mechanism of in situ AMORP was related to the pH-dependent dissolution behavior of the drug and polymer. At a pH of 6.8, IND showed a high solubility; however, swollen polymers restricted the process of drug release and ultimately solubility. Although improvement in solubility resulted at pH 6.8, no significant improvement was indicated at pH 4.5 attributed to the precipitation of amorphous salt during dissolution testing in aqueous media, pH, and the co-former present in the medium [145]. In another investigation, Huang and co-workers investigated COAs formation for challenging the poor solubility of valsartan using vibrational BM techniques with amino acids such as l-histidine, l-lysine, and l-arginine. The results for equilibrium solubility of amorphous VAL at pH 4.6, pH 6.8, and in water were 0.83 mg/mL, 5.42 mg/mL, and 0.19 mg/mL, respectively. However, the COAs mixtures, binary COAs mixtures, and ternary mixtures exhibited higher equilibrium solubility at pH 4.6, pH 6.8, and water. Besides this, the intrinsic dissolution rate of COAs formulations also indicated a similar trend at the tested pH. These results were attributed to amino acid elements in COAs mixtures that improved 1000-fold solubility over amorphous drugs due to ionization between the drug and the amino acids to enhance solvation, which facilitates dissociations into ions facilities with the formation of ion-dipole interactions with water molecules that are more energetically favorable than the hydrogen bond interactions between water and the non-ionized drug. On the other side, for the COAs system, no energy is required to break up the crystal lattice during the dissolution process. Therefore, the higher solvation energies resulting from ionization typically offset the fewer energy cost of breaking up the crystal lattice, leading to a significant increase in solubility for COAs than those free drugs [138]. Furthermore, Sormunen and colleagues investigated the effect of the co-amorphization of glibenclamide on its dissolution properties and permeability through cell layers and indicated that COAs formulation exhibited a 9-fold increase in cell permeation with solubility, compared to the alone physical mixture [140].
Co-amorphous systems are a potential approach to address the poor water solubility challenge of many drugs. Wu and colleagues established a different ratio between amino acids and their corresponding dipeptides to alter the solubility and dissolution of MBD. The results from the investigation indicated time-dependent BM techniques with the ratio of amino acids significantly improved the dissolution performance and stability [142]. Quercetin has been significantly explored in various investigations; however, its solubility reflects problems at several quantifications. Thus, an attempt to improve its solubility was studied by Minode and co-workers using mechanochemical techniques with amino acids such as beta-alanine and l-arginine. The results demonstrated that the number of radicals and the solubility of quercetin complexed with Arg was much higher than those of quercetin complexed with Ala. Interestingly, the rotational speed of grinding altered the number of radicals with improvement in the solubility of quercetin [143]. The influence of processing parameters and external conditions on the stability of amino acids with their influence on formulation stability is presented in Table II.
Challenges and Limitation
The amorphous solid state offers an improved apparent solubility and dissolution rate. However, due to thermodynamic instability and recrystallization tendencies during processing, storage, and dissolution, their potential application is limited. For this reason, the production of amorphous drug with adequate stability remains a major challenge and formulation strategies based on solid molecular dispersions are being exploited. Moreover, it is important to note that a COAs drug-drug formulation has the potential to achieve combination therapy. However, for this kind of therapy, the dose requirements of drug candidates are fairly dissimilar from that for maintaining physical stability. From a pharmaceutical standpoint, the COAs formulation’s fixed stoichiometric ratio of drug components present a particular set of difficulties. To design a commercially viable COAs formulation, the simultaneous realization of physical stability and combination therapy should be given careful consideration. For a thorough investigation of the pharmacological effects and potential functions of co-formers in biological contexts, numerous in vivo research is also necessary. Certain COAs systems may not be suitable for the industrial production of polymer-based amorphous SDs. The requirement that the API and co-former both be adequately soluble in the same solvent or stable at high temperatures may severely restrict the design space.
Conclusions and Future Perspectives
Polymeric amorphous and COAs both hold a significant degree of supersaturation and offer improved drug permeation. The main target of both systems is to prevent drugs from recrystallization. However, in vivo, pharmacodynamic and pharmacokinetic investigation along with in-depth stability studies according to regulatory protocols can give significant knowledge to fabricate amorphous systems loaded with drug and amino acid. Due to the inherent metastability driving recrystallization during preparation and storage, stability has been the key challenge for the commercial product development of the ASDs. Novel ways have therefore developed, which are being investigated with various issues as comprehensively covered in this review, to partially address such limitations. Co-amorphous systems, mesoporous particle-based amorphous systems, and in-situ AMORP are among the emerging AMORP processes that are thought to be promising PASD substitutes; nevertheless, additional research is still required for large-scale industrial applications. Multivariate visualization is a powerful method for identifying variations in amorphous materials, especially when used in conjunction with complementary spectroscopic characterization. The scale-up of prepared formulation and downstream processing of COA formulations into final dose forms will remain a challenging issue for the researchers. Certain COA systems may not be suitable for the industrial production of PASD. The requirement that the API and co-former both be adequately soluble in the same solvent or stable at high temperatures may severely restrict the design space. The addition of further excipients such as filler, binder/carriers, glidant, lubricant, or disintegrants to COA formulations may aid in enhancing the downstream processing conditions for conversion into final solid dosage forms as well as the physicochemical characteristics of COA solids. So far, only few studies have reported in vivo evaluation of drug-drug COA. High curcumin concentration administered as COA with artemisin was found in the plasma of male rats compared to administration alone, although it was not clear whether the increase was due to AMORP curcumin or co-AMORP. Additionally, studies in mice showed great improvement in the bioavailability of HDT COA mixture with atenolol. Therefore, extended clinical studies are further required to study the effect of co-administration of either active drug or AMORP active moiety with another drug.
Data Availability
The data used for the review can be availed on request by email to the corresponding authors.
Abbreviations
- Arg:
-
Aarginine
- AAs:
-
Amino acids
- AMORP:
-
Amorphization
- ASDs:
-
Amorphous solid dispersion
- ATS:
-
Atorvastatin
- BM:
-
Ball-milling
- BCS:
-
Biopharmaceutical classification system
- CBZ:
-
Carbamazepine
- CRD:
-
Carvedilol
- CEFT:
-
Ceftazidime
- COA:
-
Co-amorphous
- COAs:
-
Co-amorphous systems
- CM:
-
Cryo-milling
- DSC:
-
Differential scanning calorimetry
- FTIR:
-
Fourier-transform infrared spectroscopy
- FUR:
-
Furosemide
- Tg:
-
Glass transition temperature
- GLB:
-
Glimepiride
- GLP:
-
Glipizide
- HDT:
-
Hydrochlorothiazide
- Iso:
-
Isoleucine
- KAN:
-
Kanamycin
- LAG:
-
l-Arg/glimepiride
- Leu:
-
Leucine
- Ly:
-
Lysine
- MBD:
-
Mebendazole
- Met:
-
Methionine
- NCEs:
-
New chemical entities
- NMR:
-
Nuclear magnetic resonance
- Phel:
-
Phenylalanine
- PASDs:
-
Polymeric amorphous solid dispersions
- Pro:
-
Proline
- SEM:
-
Scan electron microscopy
- SIM:
-
Simvastatin
- ssNMR:
-
Solid-state nuclear magnetic resonance
- SCF:
-
Solution-enhanced dispersion
- SDs:
-
Solid dispersions
- Trph:
-
Tryptophan
- Tyr:
-
Tyrosine
- Val:
-
Valine
- VT:
-
Variable temperature
- XRPD:
-
X-ray powder diffraction
References
Warnken Z, Smyth HDC, Williams RO. Route-specific challenges in the delivery of poorly water-soluble drugs. In: Williams Iii RO, Davis DA, Miller DA, editors. Formulating Poorly Water Soluble Drugs. Cham: Springer International Publishing; 2022. p. 1–31.
Bhalani DV, Nutan B, Kumar A, Singh Chandel AK. Bioavailability enhancement techniques for poorly aqueous soluble drugs and therapeutics. Biomedicines. 2022;10(9). https://doi.org/10.3390/biomedicines10092055.
Batrisyia RN, Janakiraman AK, Ming LC, Helal Uddin ABM, Sarker ZI, Bin LK. A review on the solubility enhancement technique for pharmaceutical formulations. Natural Volatile and Essential Oil. 2021;8(4):3976–89.
Mendonsa N, Almutairy B, Kallakunta VR, Sarabu S, Thipsay P, Bandari S, et al. Manufacturing strategies to develop amorphous solid dispersions: an overview. J Drug Deliv Sci Technol. 2020;55: 101459. https://doi.org/10.1016/j.jddst.2019.101459.
Shi Q, Li F, Yeh S, Wang Y, Xin J. Physical stability of amorphous pharmaceutical solids: nucleation, crystal growth, phase separation and effects of the polymers. Int J Pharm. 2020;590: 119925. https://doi.org/10.1016/j.ijpharm.2020.119925.
Sihorkar V, Dürig T. The role of polymers and excipients in developing amorphous solid dispersions: An industrial perspective. In: Shegokar R, editor. In Drug Delivery Aspects. Elsevier; 2020. p. 79–113.
Liu J, Grohganz H, Löbmann K, Rades T, Hempel N-J. Co-amorphous drug formulations in numbers: recent advances in co-amorphous drug formulations with focus on co-formability, molar ratio, preparation methods, physical stability, In Vitro and In Vivo Performance, and New Formulation Strategies. Pharmaceutics. 2021. https://doi.org/10.3390/pharmaceutics13030389.
Zhu S, Gao H, Babu S, Garad S. Co-amorphous formation of high-dose zwitterionic compounds with amino acids to improve solubility and enable parenteral delivery. Mol Pharm. 2018;15(1):97–107.
Shi Q, Moinuddin SM, Cai T. Advances in coamorphous drug delivery systems. Acta Pharmaceutica Sinica B. 2019;9(1):19–35. https://doi.org/10.1016/j.apsb.2018.08.002.
Karagianni A, Kachrimanis K, Nikolakakis I. Co-amorphous solid dispersions for solubility and absorption improvement of drugs: composition, preparation, characterization and formulations for oral delivery. Pharmaceutics. 2018;10(3):98.
Löbmann K, Grohganz H, Laitinen R, Strachan C, Rades T. Amino acids as co-amorphous stabilizers for poorly water soluble drugs–part 1: preparation, stability and dissolution enhancement. Eur J Pharm Biopharm. 2013;85(3):873–81.
Löbmann K, Laitinen R, Strachan C, Rades T, Grohganz H. Amino acids as co-amorphous stabilizers for poorly water-soluble drugs–Part 2: molecular interactions. Eur J Pharm Biopharm. 2013;85(3):882–8.
Löbmann K, Strachan C, Grohganz H, Rades T, Korhonen O, Laitinen R. Co-amorphous simvastatin and glipizide combinations show improved physical stability without evidence of intermolecular interactions. Eur J Pharm Biopharm. 2012;81(1):159–69.
Kissi EO, Khorami K, Rades T. Determination of stable co-amorphous drug–drug ratios from the eutectic behavior of crystalline physical mixtures. Pharmaceutics. 2019. https://doi.org/10.3390/pharmaceutics11120628.
Aikawa S, Tanaka H, Ueda H, Maruyama M, Higaki K. Formation of a stable co-amorphous system for a brick dust molecule by utilizing sodium taurocholate with high glass transition temperature. Pharmaceutics. 2023. https://doi.org/10.3390/pharmaceutics15010084.
Berardi A, Bisharat L, AlKhatib HS, Cespi M. Zein as a Pharmaceutical excipient in oral solid dosage forms: state of the art and future perspectives. AAPS PharmSciTech. 2018;19(5):2009–22. https://doi.org/10.1208/s12249-018-1035-y.
Khan NA, Khan SU, Ahmed S, Farooqi IH, Dhingra A, Hussain A, et al. Applications of nanotechnology in water and wastewater treatment: a review. Asian J Water Environ Pollut. 2019;16(4):81–6.
Aati S, Aneja S, Kassar M, Leung R, Nguyen A, Tran S, et al. Silver-loaded mesoporous silica nanoparticles enhanced the mechanical and antimicrobial properties of 3D printed denture base resin. J Mech Behav Biomed Mater. 2022;134: 105421.
Kasten G, Grohganz H, Rades T, Löbmann K. Development of a screening method for co-amorphous formulations of drugs and amino acids. Eur J Pharm Sci. 2016;95:28–35. https://doi.org/10.1016/j.ejps.2016.08.022.
Pešić N, Dapčević A, Ivković B, Kachrimanis K, Mitrić M, Ibrić S, et al. Potential application of low molecular weight excipients for amorphization and dissolution enhancement of carvedilol. Int J Pharm. 2021;608: 121033. https://doi.org/10.1016/j.ijpharm.2021.121033.
Zhao L, Mustapha O, Shafique S, Jamshaid T, Din FU, Mehmood Y, et al. Electrospun gelatin nanocontainers for enhanced biopharmaceutical performance of Piroxicam: In vivo and in vitro investigations. Int J Nanomed. 2020;15:8819–28. https://doi.org/10.2147/ijn.S271938.
Flanagan T. Potential for pharmaceutical excipients to impact absorption: a mechanistic review for BCS Class 1 and 3 drugs. Eur J Pharm Biopharm. 2019;141:130–8. https://doi.org/10.1016/j.ejpb.2019.05.020.
Charalabidis A, Sfouni M, Bergström C, Macheras P. The biopharmaceutics classification system (BCS) and the biopharmaceutics drug disposition classification system (BDDCS): beyond guidelines. Int J Pharm. 2019;566:264–81. https://doi.org/10.1016/j.ijpharm.2019.05.041.
Kataoka M, Ikkou S, Kato Y, Minami K, Higashino H, Takagi T, et al. Effects of ingested water volume on oral absorption of fenofibrate, a BCS class II drug, from micronized and amorphous solid dispersion formulations in rats. Biol Pharm Bull. 2022;45(10):1452–7. https://doi.org/10.1248/bpb.b22-00219.
Bhatt-Mehta V, Hammoud H, Amidon GL. A proposed pediatric biopharmaceutical classification system for medications for chronic diseases in children. Eur J Pharm Sci. 2020;152: 105437. https://doi.org/10.1016/j.ejps.2020.105437.
Ting JM, Porter WW III, Mecca JM, Bates FS, Reineke TM. Advances in polymer design for enhancing oral drug solubility and delivery. Bioconjugate Chem. 2018;29(4):939–52.
delMoral-Sanchez J-M, Gonzalez-Alvarez I, Gonzalez-Alvarez M, Navarro A, Bermejo M. Classification of WHO essential oral medicines for children applying a provisional pediatric biopharmaceutics classification system. Pharmaceutics. 2019. https://doi.org/10.3390/pharmaceutics11110567.
Chaturvedi S, Mishra R. Insight into delivery approaches for biopharmaceutics classification system class II and IV drugs. Drug Deliv Lett. 2020;10(4):255–77. https://doi.org/10.2174/2210303110999200712185109.
Singh S, Shyale S, Sandip H. Improved dissolution properties of ketoconazole through application of liquisolid techniques. Int J Pharm Sci Nanotechnol (IJPSN). 2015;8(4):3053–9.
Kendre PN, Lokwani D, Pote A, Singh S, Jayeoye TJ, Prajapati BG. Potential technologies to develop cellulose beads and microspheres. Regenerated cellulose and composites: morphology-property relationship. Springer; 2023. p. 145–76.
Arslan A, Yet B, Nemutlu E, Akdağ Çaylı Y, Eroğlu H, Öner L. Celecoxib nanoformulations with enhanced solubility, dissolution rate, and oral bioavailability: Experimental approaches over in vitro/in vivo Evaluation. Pharmaceutics. 2023;15(2):363.
Kapoor DU, Gaur M, Parihar A, Prajapati BG, Singh S, Patel RJ. Phosphatidylcholine (PCL) fortified nano-phytopharmaceuticals for improvement of therapeutic efficacy. EXCLI J. 2023;22:880–903.
Vasuki A, Christy HJ, Renugadevi K, Dammalli M. Structure-based pharmacophore modeling and DFT studies of Indian Ocean-derived red algal compounds as PI3Kα inhibitors. Mol Divers. 2023:1–19.
Markovic M, Zur M, Ragatsky I, Cvijić S, Dahan A. BCS class IV oral drugs and absorption windows: regional-dependent intestinal permeability of furosemide. Pharmaceutics. 2020;12(12). https://doi.org/10.3390/pharmaceutics12121175.
Laitinen R, Löbmann K, Grohganz H, Priemel P, Strachan CJ, Rades T. Supersaturating drug delivery systems: the potential of co-amorphous drug formulations. Int J Pharm. 2017;532(1):1–12. https://doi.org/10.1016/j.ijpharm.2017.08.123.
Shi X, Fan B, Gu C, Zhou X, Wang C, Ding Z. Ibrutinib and carboxylic acid coamorphous system with increased solubility and dissolution: a potential interaction mechanism. J Drug Deliv Sci Technol. 2020;59: 101875. https://doi.org/10.1016/j.jddst.2020.101875.
Li B, Wang Y, Feng Y, Yuan D, Xu R, Jiang C, et al. Design and molecular insights of drug-active metabolite based co-amorphous formulation: a case study of toltrazuril-ponazuril co-amorphous. Int J Pharm. 2022;615: 121475. https://doi.org/10.1016/j.ijpharm.2022.121475.
Deng Y, Deng W, Huang W, Zheng Z, Zhang R, Liu S, et al. Norfloxacin co-amorphous salt systems: effects of molecular descriptors on the formation and physical stability of co-amorphous systems. Chemi Eng Sci. 2022;253: 117549. https://doi.org/10.1016/j.ces.2022.117549.
Liu J, Grohganz H, Rades T. Influence of polymer addition on the amorphization, dissolution and physical stability of co-amorphous systems. Int J Pharm. 2020;588: 119768. https://doi.org/10.1016/j.ijpharm.2020.119768.
Suresh K, Mannava MKC, Nangia A. A novel curcumin–artemisinin coamorphous solid: physical properties and pharmacokinetic profile. RSC Adv. 2014;4(102):58357–61. https://doi.org/10.1039/C4RA11935E.
Löbmann K, Laitinen R, Grohganz H, Gordon KC, Strachan C, Rades T. Coamorphous drug systems: enhanced physical stability and dissolution rate of indomethacin and Naproxen. Mol Pharm. 2011;8(5):1919–28. https://doi.org/10.1021/mp2002973.
Wong MK, Brooks DJ, Ikejezie J, Gacic-Dobo M, Dumolard L, Nedelec Y, et al. COVID-19 mortality and progress toward vaccinating older adults—World Health Organization, worldwide, 2020–2022. Morb Mortal Wkly Rep. 2023;72(5):113.
Han J, Wei Y, Lu Y, Wang R, Zhang J, Gao Y, et al. Co-amorphous systems for the delivery of poorly water-soluble drugs: recent advances and an update. Expert Opin Drug Deliv. 2020;17(10):1411–35. https://doi.org/10.1080/17425247.2020.1796631.
Huang R, Han J, Wang R, Zhao X, Qiao H, Chen L, et al. Surfactant-free solid dispersion of BCS class IV drug in an amorphous chitosan oligosaccharide matrix for concomitant dissolution in vitro—permeability increase. Eur J Pharm Sci. 2019;130:147–55. https://doi.org/10.1016/j.ejps.2019.01.031.
Sai Krishna Anand V, Sakhare SD, Navya Sree KS, Nair AR, Raghava Varma K, Gourishetti K, et al. The relevance of co-amorphous formulations to develop supersaturated dosage forms: In-vitro, and ex-vivo investigation of Ritonavir-Lopinavir co-amorphous materials. Eur J Pharm Sci. 2018;123:124-34. https://doi.org/10.1016/j.ejps.2018.07.046.
da Costa NF, Pinto JF, Fernandes AI. Co-amorphization of olanzapine for solubility enhancement. Ann Med. 2019;51(sup1):87-. https://doi.org/10.1080/07853890.2018.1561801.
Phan AD, Thu Thuy TT, Kim An NT, Knapik-Kowalczuk J, Paluch M, Wakabayashi K. Molecular relaxations in supercooled liquid and glassy states of amorphous gambogic acid: Dielectric spectroscopy, calorimetry, and theoretical approach. AIP Adv. 2020;10(2): 025128. https://doi.org/10.1063/1.5139101.
Mishra DK, Dhote V, Bhargava A, Jain DK, Mishra PK. Amorphous solid dispersion technique for improved drug delivery: basics to clinical applications. Drug Deliv Transl Res. 2015;5(6):552–65. https://doi.org/10.1007/s13346-015-0256-9.
Fael H, Demirel AL. Tannic acid as a co-former in co-amorphous systems: Enhancing their physical stability, solubility and dissolution behavior. Int J Pharm. 2020;581: 119284. https://doi.org/10.1016/j.ijpharm.2020.119284.
Kanaujia P, Poovizhi P, Ng WK, Tan RBH. Amorphous formulations for dissolution and bioavailability enhancement of poorly soluble APIs. Powder Technol. 2015;285:2–15. https://doi.org/10.1016/j.powtec.2015.05.012.
Mamidi HK, Rohera BD. Application of thermodynamic phase diagrams and gibbs free energy of mixing for screening of polymers for their use in amorphous solid dispersion formulation of a non-glass-forming drug. J Pharm Sci. 2021;110(7):2703–17. https://doi.org/10.1016/j.xphs.2021.01.036.
Deng Y, Liu S, Jiang Y, Martins ICB, Rades T. Recent advances in co-former screening and formation prediction of multicomponent solid forms of low molecular weight drugs. Pharmaceutics. 2023;15(9):2174.
Hetal K, Shreel B, Singh S. Development of colon delivery tablets of albendazole using pH-dependent polymers. Int J Pharm Sci Nanotechnol (IJPSN). 2013;6(4):2219–24.
Nadaf S, Jadhav A, Killedar S. Mung bean (Vigna radiata) porous starch for solubility and dissolution enhancement of poorly soluble drug by solid dispersion. Int J Biol Macromol. 2021;167:345–57. https://doi.org/10.1016/j.ijbiomac.2020.11.172.
Wei W, Lu M, Xu W, Polyakov NE, Dushkin AV, Su WK. Preparation of protamine-hyaluronic acid coated core-shell nanoparticles for enhanced solubility, permeability, and oral bioavailability of decoquinate. Int J Biol Macromol. 2022;218:346–55. https://doi.org/10.1016/j.ijbiomac.2022.07.152.
Martínez LM, Videa M, López Silva T, Castro S, Caballero A, Lara-Díaz VJ, et al. Two-phase amorphous-amorphous solid drug dispersion with enhanced stability, solubility and bioavailability resulting from ultrasonic dispersion of an immiscible system. Eur J Pharm Biopharm. 2017;119:243–52. https://doi.org/10.1016/j.ejpb.2017.06.021.
Karmwar P, Graeser K, Gordon KC, Strachan CJ, Rades T. Investigation of properties and recrystallisation behaviour of amorphous indomethacin samples prepared by different methods. Int J Pharm. 2011;417(1–2):94–100. https://doi.org/10.1016/j.ijpharm.2010.12.019.
Kim DH, Kim YW, Tin YY, Soe MT, Ko BH, Park SJ, et al. Recent technologies for amorphization of poorly water-soluble drugs. Pharmaceutics. 2021;13(8). https://doi.org/10.3390/pharmaceutics13081318.
Baghel S, Cathcart H, O’Reilly NJ. Polymeric amorphous solid dispersions: a review of amorphization, crystallization, stabilization, solid-state characterization, and aqueous solubilization of biopharmaceutical classification system class II drugs. J Pharm Sci. 2016;105(9):2527–44. https://doi.org/10.1016/j.xphs.2015.10.008.
Gröls JR, Castro-Dominguez B. Intelligent mechanochemical design of co-amorphous mixtures. Cryst Growth Des. 2022;22(5):2989–96. https://doi.org/10.1021/acs.cgd.1c01442.
Dengale SJ, Grohganz H, Rades T, Löbmann K. Recent advances in co-amorphous drug formulations. Adv Drug Deliv Rev. 2016;100:116–25. https://doi.org/10.1016/j.addr.2015.12.009.
Yu L, Reutzel-Edens SM, Mitchell CA. Crystallization and polymorphism of conformationally flexible molecules: problems, patterns, and strategies. Org Process Res Dev. 2000;4(5):396–402. https://doi.org/10.1021/op000028v.
Moinuddin SM, Ruan S, Huang Y, Gao Q, Shi Q, Cai B, et al. Facile formation of co-amorphous atenolol and hydrochlorothiazide mixtures via cryogenic-milling: Enhanced physical stability, dissolution and pharmacokinetic profile. Int J Pharm. 2017;532(1):393–400. https://doi.org/10.1016/j.ijpharm.2017.09.020.
Löbmann K, Grohganz H, Laitinen R, Strachan C, Rades T. Amino acids as co-amorphous stabilizers for poorly water soluble drugs—Part 1: Preparation, stability and dissolution enhancement. European Journal of Pharmaceutics and Biopharmaceutics. 2013;85(3, Part B):873-81. https://doi.org/10.1016/j.ejpb.2013.03.014.
Renuka, Singh SK, Gulati M, Narang R. Stable amorphous binary systems of glipizide and atorvastatin powders with enhanced dissolution profiles: formulation and characterization. Pharm Dev Technol. 2017;22(1):13-25. https://doi.org/10.3109/10837450.2015.1125921.
Kondo K, Rades T. Solventless amorphization and pelletization using a high shear granulator. Part I; feasibility study using indomethacin. Eur J Pharm Biopharm. 2022;181:147–58. https://doi.org/10.1016/j.ejpb.2022.11.010.
Silva JFC, Pereira Silva PS, Ramos Silva M, Fantechi E, Chelazzi L, Ciattini S, et al. Amorphous solid forms of ranolazine and tryptophan and their relaxation to metastable polymorphs. Cryst Growth Des. 2023;23(9):6679–91. https://doi.org/10.1021/acs.cgd.3c00565.
Furuishi T, Sato-Hata N, Fukuzawa K, Yonemochi E. Characterization of co-amorphous carvedilol–maleic acid system prepared by solvent evaporation. Pharm Dev Technol. 2023;28(3–4):309–17. https://doi.org/10.1080/10837450.2023.2194406.
Park H, Jin Seo H, Hong S-h, Ha E-S, Lee S, Kim J-S, et al. Characterization and therapeutic efficacy evaluation of glimepiride and L-arginine co-amorphous formulation prepared by supercritical antisolvent process: Influence of molar ratio and preparation methods. International Journal of Pharmaceutics. 2020;581:119232. https://doi.org/10.1016/j.ijpharm.2020.119232.
Ningaraju S, Munawer U, Raghavendra VB, Balaji KS, Melappa G, Brindhadevi K, et al. Chaetomium globosum extract mediated gold nanoparticle synthesis and potent anti-inflammatory activity. Anal Biochem. 2021;612: 113970. https://doi.org/10.1016/j.ab.2020.113970.
Li Z, Zhang Y, Feng N. Mesoporous silica nanoparticles: synthesis, classification, drug loading, pharmacokinetics, biocompatibility, and application in drug delivery. Exp Opin Drug Deliv. 2019;16(3):219–37. https://doi.org/10.1080/17425247.2019.1575806.
Vallet-Regí M, Balas F, Arcos D. Mesoporous Materials for Drug Delivery. Angew Chem Int Ed. 2007;46(40):7548–58. https://doi.org/10.1002/anie.200604488.
Raza A, Sime FB, Cabot PJ, Roberts JA, Falconer JR, Kumeria T, et al. Liquid CO2 formulated mesoporous silica nanoparticles for pH-responsive oral delivery of meropenem. ACS Biomater Sci Eng. 2021;7(5):1836–53. https://doi.org/10.1021/acsbiomaterials.0c01284.
Mužík J, Lizoňová D, Zadražil A, Štěpánek F. Drug amorphisation by fluid bed hot-melt impregnation of mesoporous silica carriers. Chem Eng J. 2020;392: 123754. https://doi.org/10.1016/j.cej.2019.123754.
Wdowiak K, Pietrzak R, Tykarska E, Cielecka-Piontek J. Hot-melt extrusion as an effective technique for obtaining an amorphous system of curcumin and piperine with improved properties essential for their better biological activities. Molecules. 2023;28(9):3848.
Shah S, Maddineni S, Lu J, Repka MA. Melt extrusion with poorly soluble drugs. Int J Pharm. 2013;453(1):233–52. https://doi.org/10.1016/j.ijpharm.2012.11.001.
Lenz E, Löbmann K, Rades T, Knop K, Kleinebudde P. Hot melt extrusion and spray drying of co-amorphous indomethacin-arginine with polymers. J Pharm Sci. 2017;106(1):302–12. https://doi.org/10.1016/j.xphs.2016.09.027.
Shi N-Q, Wang S-R, Zhang Y, Huo J-S, Wang L-N, Cai J-H, et al. Hot melt extrusion technology for improved dissolution, solubility and “spring-parachute” processes of amorphous self-micellizing solid dispersions containing BCS II drugs indomethacin and fenofibrate: Profiles and mechanisms. Eur J Pharm Sci. 2019;130:78–90. https://doi.org/10.1016/j.ejps.2019.01.019.
Hempel N-J, Knopp MM, Berthelsen R, Zeitler JA, Löbmann K. The influence of drug and polymer particle size on the in situ amorphization using microwave irradiation. Eur J Pharm Biopharm. 2020;149:77–84. https://doi.org/10.1016/j.ejpb.2020.01.019.
Feng L, Qian C, Zhao Y. Recent advances in covalent organic framework-based nanosystems for bioimaging and therapeutic applications. ACS Mater Lett. 2020;2(9):1074–92. https://doi.org/10.1021/acsmaterialslett.0c00260.
Qiang W, Löbmann K, McCoy CP, Andrews GP, Zhao M. Microwave-induced In Situ Amorphization: a new strategy for tackling the stability issue of amorphous solid dispersions. Pharmaceutics. 2020;12(7):655.
Priemel PA, Laitinen R, Grohganz H, Rades T, Strachan CJ. In situ amorphisation of indomethacin with Eudragit® E during dissolution. European Journal of Pharmaceutics and Biopharmaceutics. 2013;85(3, Part B):1259-65. https://doi.org/10.1016/j.ejpb.2013.09.010.
Petry I, Löbmann K, Grohganz H, Rades T, Leopold CS. In situ co-amorphisation of arginine with indomethacin or furosemide during immersion in an acidic medium—a proof of concept study. Eur J Pharm Biopharm. 2018;133:151–60. https://doi.org/10.1016/j.ejpb.2018.10.011.
Petry I, Löbmann K, Grohganz H, Rades T, Leopold CS. In situ co-amorphisation in coated tablets—the combination of carvedilol with aspartic acid during immersion in an acidic medium. Int J Pharm. 2019;558:357–66. https://doi.org/10.1016/j.ijpharm.2018.12.091.
Qiang W, Löbmann K, McCoy CP, Andrews GP, Zhao M. The effects of surfactants on the performance of polymer-based microwave-induced in situ amorphization. Int J Pharm. 2023;630: 122426. https://doi.org/10.1016/j.ijpharm.2022.122426.
Hempel N-J, Merkl P, Asad S, Knopp MM, Berthelsen R, Bergstrom CA, et al. Utilizing laser activation of photothermal plasmonic nanoparticles to induce on-demand drug amorphization inside a tablet. Mol Pharm. 2021;18(6):2254–62.
Hoffmann L, Breitkreutz J, Quodbach J. Investigation of the degradation and in-situ amorphization of the enantiomeric drug escitalopram oxalate during fused deposition modeling (FDM) 3D printing. Eur J Pharm Sci. 2023;185: 106423. https://doi.org/10.1016/j.ejps.2023.106423.
Priemel PA, Laitinen R, Grohganz H, Rades T, Strachan CJ. In situ amorphisation of indomethacin with Eudragit® E during dissolution. Eur J Pharm Biopharm. 2013;85(3):1259–65.
Doreth M, Löbmann K, Grohganz H, Holm R, de Diego HL, Rades T, et al. Glass solution formation in water-In situ amorphization of naproxen and ibuprofen with Eudragit® E PO. J Drug Deliv Sci Technol. 2016;34:32–40.
Ontong JC, Singh S, Nwabor OF, Chusri S, Voravuthikunchai SP. Potential of antimicrobial topical gel with synthesized biogenic silver nanoparticle using Rhodomyrtus tomentosa leaf extract and silk sericin. Biotechnol Lett. 2020;42(12):2653–64. https://doi.org/10.1007/s10529-020-02971-5.
Spiliopoulou M, Valmas A, Triandafillidis D-P, Kosinas C, Fitch A, Karavassili F, et al. Applications of X-ray Powder diffraction in protein crystallography and drug screening. Crystals. 2020. https://doi.org/10.3390/cryst10020054.
Corbi PP, Massabni AC, Costa-Neto CM. X-ray powder diffraction analysis of a new palladium(II) amino acid complex. Powder Diffr. 2004;19(3):270–1. https://doi.org/10.1154/1.1648317.
Vijayan N, Rajasekaran S, Bhagavannarayana G, Ramesh Babu R, Gopalakrishnan R, Palanichamy M, et al. Growth and characterization of nonlinear optical amino acid single crystal: l-alanine. Cryst Growth Des. 2006;6(11):2441–5. https://doi.org/10.1021/cg049594y.
Bundschuh M, Filser J, Lüderwald S, McKee MS, Metreveli G, Schaumann GE, et al. Nanoparticles in the environment: where do we come from, where do we go to? Environ Sci Eur. 2018;30(1):1–17.
Sudarshan Singh WC. Thermo-mechanical properties of bio-based polymers, biopolymers towards green and sustainable development. In: Sudarshan Singh WC, editor. Biopolymers Towards Green and Sustainable Development: Bentham Science Publisher; 2022. p. 1: 90.
Sturtevant JM. Biochemical applications of differential scanning calorimetry. Ann Rev Phys Chem. 1987;38(1):463–88.
Sabulal B, Kishore N. Differential scanning calorimetric study of the interactions of some stabilizing amino acids and oligopeptides with hen egg white lysozyme. J Chem Soc Faraday Trans. 1995;91(14):2101–6. https://doi.org/10.1039/FT9959102101.
Sudarshan Singh WC. Conformational, morphological, and physical characterization of bio-based polymers, biopolymers towards green and sustainable development. In: Sudarshan Singh WC, editor. Biopolymers Towards Green and Sustainable Development: Bentham Science Publisher; 2022. p. 1:73.
Singh S, Nwabor OF, Sukri DM, Wunnoo S, Dumjun K, Lethongkam S, et al. Poly (vinyl alcohol) copolymerized with xanthan gum/hypromellose/sodium carboxymethyl cellulose dermal dressings functionalized with biogenic nanostructured materials for antibacterial and wound healing application. Int J Biol Macromol. 2022;216:235–50. https://doi.org/10.1016/j.ijbiomac.2022.06.172.
Furuyama N, Hasegawa S, Hamaura T, Yada S, Nakagami H, Yonemochi E, et al. Evaluation of solid dispersions on a molecular level by the Raman mapping technique. Int J Pharm. 2008;361(1):12–8. https://doi.org/10.1016/j.ijpharm.2008.05.009.
Sinclair W, Leane M, Clarke G, Dennis A, Tobyn M, Timmins P. Physical stability and recrystallization kinetics of amorphous ibipinabant drug product by fourier transform raman spectroscopy. J Pharm Sci. 2011;100:4687–99. https://doi.org/10.1002/jps.22658.
Tiernan H, Byrne B, Kazarian SG. ATR-FTIR spectroscopy and spectroscopic imaging for the analysis of biopharmaceuticals. Spectrochim Acta A Mol Biomol Spectrosc. 2020;241: 118636. https://doi.org/10.1016/j.saa.2020.118636.
Dhamelincourt P, Ramirez FJ. Polarized micro-raman and FT-IR spectra of L-glutamine. Appl Spectrosc. 1993;47(4):446–51. https://doi.org/10.1366/0003702934335083.
Shen F, Niu X, Yang D, Ying Y, Li B, Zhu G, et al. Determination of amino acids in Chinese rice wine by fourier transform near-infrared spectroscopy. J Agric Food Chem. 2010;58(17):9809–16. https://doi.org/10.1021/jf1017912.
Ito A, Watanabe T, Yada S, Hamaura T, Nakagami H, Higashi K, et al. Prediction of recrystallization behavior of troglitazone/polyvinylpyrrolidone solid dispersion by solid-state NMR. Int J Pharm. 2010;383(1):18–23. https://doi.org/10.1016/j.ijpharm.2009.08.037.
Shi X, Holland GP, Yarger JL. Amino acid analysis of spider dragline silk using 1H NMR. Anal Biochem. 2013;440(2):150–7. https://doi.org/10.1016/j.ab.2013.05.006.
Ciampa A, Picone G, Laghi L, Nikzad H, Capozzi F. Changes in the amino acid composition of Bogue (Boops boops) fish during storage at different temperatures by 1H-NMR spectroscopy. Nutrients. 2012;4(6):542–53.
Hsieh LY, Chan HH, Kuo PC, Hung HY, Li YC, Kuo CL, et al. A feasible and practical (1)H NMR analytical method for the quality control and quantification of bioactive principles in Lycii Fructus. J Food Drug Anal. 2018;26(3):1105–12. https://doi.org/10.1016/j.jfda.2018.01.001.
Pajula K, Wittoek L, Lehto V-P, Ketolainen J, Korhonen O. Phase separation in coamorphous systems: in silico prediction and the experimental challenge of detection. Mol Pharm. 2014;11(7):2271–9.
Jensen KT, Larsen FH, Cornett C, Löbmann K, Grohganz H, Rades T. Formation mechanism of coamorphous drug–amino acid mixtures. Mol Pharm. 2015;12(7):2484–92.
Korhonen O, Pajula K, Laitinen R. Rational excipient selection for co-amorphous formulations. Exp Opin Drug Deliv. 2017;14(4):551–69.
Chavan RB, Thipparaboina R, Kumar D, Shastri NR. Co amorphous systems: a product development perspective. Int J Pharm. 2016;515(1):403–15. https://doi.org/10.1016/j.ijpharm.2016.10.043.
Ruponen M, Kettunen K, Santiago Pires M, Laitinen R. Co-amorphous formulations of furosemide with arginine and P-glycoprotein inhibitor drugs. Pharmaceutics. 2021. https://doi.org/10.3390/pharmaceutics13020171.
Holzapfel K, Rades T, Leopold CS. Co-amorphous systems consisting of indomethacin and the chiral co-former tryptophan: solid-state properties and molecular mobilities. Int J Pharm. 2023:122840.
Wang Y, Rades T, Grohganz H. Considerations on the kinetic processes in the preparation of ternary co-amorphous systems by milling. Pharmaceutics. 2023;15(1):172. https://doi.org/10.3390/pharmaceutics15010172
Wu W, Löbmann K, Schnitzkewitz J, Knuhtsen A, Pedersen DS, Rades T, et al. Dipeptides as co-formers in co-amorphous systems. Eur J Pharm Biopharm. 2019;134:68–76. https://doi.org/10.1016/j.ejpb.2018.11.016.
Lenz E, Jensen KT, Blaabjerg LI, Knop K, Grohganz H, Löbmann K, et al. Solid-state properties and dissolution behaviour of tablets containing co-amorphous indomethacin–arginine. Eur J Pharm Biopharm. 2015;96:44–52. https://doi.org/10.1016/j.ejpb.2015.07.011.
Lu W, Rades T, Rantanen J, Chan H-K, Yang M. Amino acids as stabilizers for spray-dried simvastatin powder for inhalation. Int J Pharm. 2019;572: 118724. https://doi.org/10.1016/j.ijpharm.2019.118724.
Telange DR, Jain SP, Pethe AM, Kharkar PS. Egg white protein carrier-assisted development of solid dispersion for improved aqueous solubility and permeability of poorly water soluble hydrochlorothiazide. AAPS PharmSciTech. 2021;22(3):94. https://doi.org/10.1208/s12249-021-01967-2.
Mishra J, Bohr A, Rades T, Grohganz H, Löbmann K. Whey proteins as stabilizers in amorphous solid dispersions. Eur J Pharm Sci. 2019;128:144–51. https://doi.org/10.1016/j.ejps.2018.12.002.
Jbilou F, Ayadi F, Galland S, Joly C, Dole P, Belard L, et al. Effect of shear stress extrusion intensity on plasticized corn flour structure: proteins role and distribution. J Appl Polymer Sci. 2012;123(4):2177–86. https://doi.org/10.1002/app.34737.
Adhikari BR, Bērziņš K, Fraser-Miller SJ, Gordon KC, Das SC. Co-amorphization of kanamycin with amino acids improves aerosolization. Pharmaceutics. 2020;12(8). https://doi.org/10.3390/pharmaceutics12080715.
Li M, Wang M, Liu Y, Ouyang R, Liu M, Han D, et al. Co-amorphization story of furosemide-amino acid systems: protonation and aromatic stacking insights for promoting compatibility and stability. Cryst Growth Des. 2021;21(6):3280–9. https://doi.org/10.1021/acs.cgd.1c00015.
Dahan A, Zimmermann EM, Ben-Shabat S. Modern prodrug design for targeted oral drug delivery. Molecules. 2014;19(10):16489–505.
Vale N, Ferreira A, Matos J, Fresco P, Gouveia MJ. Amino acids in the development of prodrugs. Molecules. 2018. https://doi.org/10.3390/molecules23092318.
Katragadda S, Gunda S, Hariharan S, Mitra AK. Ocular pharmacokinetics of acyclovir amino acid ester prodrugs in the anterior chamber: evaluation of their utility in treating ocular HSV infections. Int J Pharm. 2008;359(1–2):15–24.
Patel K, Trivedi S, Luo S, Zhu X, Pal D, Kern ER, et al. Synthesis, physicochemical properties and antiviral activities of ester prodrugs of ganciclovir. Int J Pharm. 2005;305(1–2):75–89.
Ojarinta R, Heikkinen AT, Sievänen E, Laitinen R. Dissolution behavior of co-amorphous amino acid-indomethacin mixtures: the ability of amino acids to stabilize the supersaturated state of indomethacin. Eur J Pharm Biopharm. 2017;112:85–95. https://doi.org/10.1016/j.ejpb.2016.11.023.
Jensen KT, Löbmann K, Rades T, Grohganz H. Improving co-amorphous drug formulations by the addition of the highly water soluble amino acid, proline. Pharmaceutics. 2014;6(3):416–35.
Jensen KT, Larsen FH, Löbmann K, Rades T, Grohganz H. Influence of variation in molar ratio on co-amorphous drug-amino acid systems. Eur J Pharm Biopharm. 2016;107:32–9. https://doi.org/10.1016/j.ejpb.2016.06.020.
Ordoubadi M, Shepard KB, Wang H, Wang Z, Pluntze AM, Churchman JP, et al. On the physical stability of leucine-containing spray-dried powders for respiratory drug delivery. Pharmaceutics. 2023;15(2):435.
Martin A, Cai J, Schaedel A-L, van der Plas M, Malmsten M, Rades T, et al. Zein-polycaprolactone core–shell nanofibers for wound healing. Int J Pharm. 2022;621: 121809. https://doi.org/10.1016/j.ijpharm.2022.121809.
Guinet Y, Paccou L, Hédoux A. Mechanism for stabilizing an amorphous drug using amino acids within co-amorphous blends. Pharmaceutics. 2023;15(2):337.
Vasilev NA, Voronin AP, Surov AO, Perlovich GL. Influence of co-amorphization on the physical stability and dissolution performance of an anthelmintic drug flubendazole. Mol Pharm. 2023;20(3):1657–69. https://doi.org/10.1021/acs.molpharmaceut.2c00873.
El-Maradny H, Saab M. Spray-dried co-amorphous Tadalafil ternary mixtures: a promising platform towards the enhancement of solubility and bioavailability. Braz J Pharm Sci. 2023;58:1–14. https://doi.org/10.1590/s2175-97902022e20622.
Raj Adhikari B, Sinha S, Lyons N, Pletzer D, Lamont I, Gordon KC, et al. Inhalable ceftazidime-roflumilast powder targeting infection and inflammation: influence of incorporating roflumilast into ceftazidime-leucine co-amorphous formulation. Eur J Pharm Biopharm. 2022;180:260–8. https://doi.org/10.1016/j.ejpb.2022.10.005.
Laitinen R, Löbmann K, Grohganz H, Strachan C, Rades T. Amino acids as co-amorphous excipients for simvastatin and glibenclamide: physical properties and stability. Mol Pharm. 2014;11(7):2381–9. https://doi.org/10.1021/mp500107s.
Huang Y, Zhang Q, Wang J-R, Lin K-L, Mei X. Amino acids as co-amorphous excipients for tackling the poor aqueous solubility of valsartan. Pharm Dev Technol. 2017;22(1):69–76. https://doi.org/10.3109/10837450.2016.1163390.
Adhikari BR, Gordon KC, Das SC. Co-amorphization: a formulation strategy for amorphous high dose dry powder to treat lung infections. Drug Delivery to the lungs. 2021;32:1–3.
Sormunen H, Ruponen M, Laitinen R. The effect of co-amorphization of glibenclamide on its dissolution properties and permeability through an MDCKII-MDR1 cell layer. Int J Pharm. 2019;570: 118653. https://doi.org/10.1016/j.ijpharm.2019.118653.
Mishra J, Löbmann K, Grohganz H, Rades T. Influence of preparation technique on co-amorphization of carvedilol with acidic amino acids. Int J Pharm. 2018;552(1):407–13. https://doi.org/10.1016/j.ijpharm.2018.09.070.
Wu W, Grohganz H, Rades T, Löbmann K. Comparison of co-former performance in co-amorphous formulations: single amino acids, amino acid physical mixtures, amino acid salts and dipeptides as co-formers. Eur J Pharm Sci. 2021;156: 105582. https://doi.org/10.1016/j.ejps.2020.105582.
Minode M, Kadota K, Kawabata D, Yoshida M, Shirakawa Y. Enhancement in dissolution behavior and antioxidant capacity of quercetin with amino acids following radical formation via mechanochemical technique. Adv Powder Technol. 2022;33(5): 103582. https://doi.org/10.1016/j.apt.2022.103582.
Liu J, Rades T, Grohganz H. Determination of the optimal molar ratio in amino acid-based coamorphous systems. Mol Pharm. 2020;17(4):1335–42. https://doi.org/10.1021/acs.molpharmaceut.0c00042.
Lenz E, Jensen KT, Blaabjerg LI, Knop K, Grohganz H, Löbmann K, et al. Solid-state properties and dissolution behaviour of tablets containing co-amorphous indomethacin-arginine. Eur J Pharm Biophar. 2015;96:44–52. https://doi.org/10.1016/j.ejpb.2015.07.011.
Zou Z, Huang Q, Li X, Liu X, Yin L, Zhao Y, et al. Dissolution changes in drug-amino acid/biotin co-amorphous systems: decreased/increased dissolution during storage without recrystallization. Eur J Pharm Sci. 2023;188: 106526. https://doi.org/10.1016/j.ejps.2023.106526.
Li M, Jin Z, Han D, Wu S, Gong J. Co-former selection for coamorphous amino acid/spironolactone formulations and exploration of the amorphization kinetics of systems. Cryst Growth Des. 2023;23(3):1511–21. https://doi.org/10.1021/acs.cgd.2c01092.
Garbiec E, Rosiak N, Tykarska E, Zalewski P, Cielecka-Piontek J. Sinapic acid co-amorphous systems with amino acids for improved solubility and antioxidant activity. Int J Mol Sci. 2023;24(6):5533.
Alsenz J, Haenel E. Precellys® Evolution Homogenizer—a versatile instrument for milling, mixing, and amorphization of drugs in preformulation. Eur J Pharm Biopharm. 2023;189:1–14. https://doi.org/10.1016/j.ejpb.2023.05.002.
Patil U, Rawal S, Pantwalawalkar J, Nangare S, Dagade D, Patil P, et al. Development of amino acid salt-based curcumin@ lysine acetate co-amorphous system using liquid-assisted grinding for improved solubility and dissolution. Thai J Pharm Sci. 2023;46(6):711–9.
Acknowledgement
This work was partially supported by CMU Proactive Researcher Scheme (2023), Chiang Mai University “Contract No.933/2566”.
Funding
This work was partially supported by CMU Proactive Researcher Scheme (2023), Chiang Mai University “Contract No.933/2566”.
Author information
Authors and Affiliations
Contributions
Sudarshan Singh: conceptualization, supervision, writing, editing, and reviewing; Bhupendra G Prajapati: supervision, editing, and reviewing; Devesh U Kapoor and Pratishtha Sharma: writing original draft.
Corresponding authors
Ethics declarations
Competing Interests
The authors declare no competing interests.
Additional information
Publisher's Note
Springer Nature remains neutral with regard to jurisdictional claims in published maps and institutional affiliations.
Rights and permissions
Springer Nature or its licensor (e.g. a society or other partner) holds exclusive rights to this article under a publishing agreement with the author(s) or other rightsholder(s); author self-archiving of the accepted manuscript version of this article is solely governed by the terms of such publishing agreement and applicable law.
About this article
Cite this article
Kapoor, D.U., Singh, S., Sharma, P. et al. Amorphization of Low Soluble Drug with Amino Acids to Improve Its Therapeutic Efficacy: a State-of-Art-Review. AAPS PharmSciTech 24, 253 (2023). https://doi.org/10.1208/s12249-023-02709-2
Received:
Accepted:
Published:
DOI: https://doi.org/10.1208/s12249-023-02709-2