Abstract—
Methods for the preparation of GaAs–CdSe and ZnTe–CdSe solid solutions based on isothermal diffusion of the initial binary compounds (GaAs and CdSe, ZnTe and CdSe) with their main bulk physical and physicochemical properties taken into account are developed. X-ray, microscopic, electron-microscopic, and spectroscopic data enriched the database on multicomponent diamond-like semiconductors. They include the luminescence properties, average sizes, and average numbers of particles. This makes it possible to unambiguously certify solid solutions as of the substitutional type with a cubic sphalerite and wurtzite hexagonal structures depending on the composition. The surface properties (chemical composition and acid-base properties) of the solid solutions and binary components of the systems are studied. When the surfaces are predominantly weakly acidic, there is a relative increase in the basicity with an increase in the amount of CdSe and ZnTe in the GaAs–CdSe and ZnTe–CdSe systems, respectively. The changes in the composition of bulk and surface properties and the correlations between them are found. This simplifies the search for new materials—the primary transducers of sensors.
Similar content being viewed by others
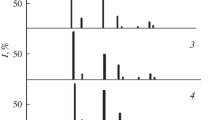
Avoid common mistakes on your manuscript.
INTRODUCTION
Success in the development of one of the most important areas of modern technology, i.e., the sensor technology, depends on sensors, which possess high selective sensitivity, low operating temperatures, simple design, low cost, and other more attractive technical and economic indicators in comparison with widespread oxide sensors [1, 2]. The production of such sensors depends on the materials used, i.e., the primary converters.
Considering that binary diamond-like compounds, especially АIIIBV and АIIBVI one [2], possess unique properties, the search for effective materials based on the preparation and study of multicomponent diamond-like semiconductors seems to be promising. In this case, both the expected smooth and unexpected extreme changes in properties and composition are possible due to complex internal processes to form solid solutions. Both these changes are interesting in scientific and practical aspects when considering the АIIIBV–АIIBVI, АIIBVI–АIIBVI systems, among which GaAs–CdSe and ZnTe–CdSe are studied in this work.
The preparation and certification of GaAs–CdSe and ZnTe–CdSe solid solutions based on X-ray, microscopic, electron microscopic, and spectroscopic studies (the Raman scattering of light (RS), IR spectroscopy, and Auger spectroscopy) are relevant tasks. To get additional information about multicomponent diamond-like semiconductors, the complex study of acid-base properties of the surfaces of system components, the identification of patterns of changes with the composition of bulk and surface properties, determination of the relative influence of GaAs and ZnTe binary components, and assessment of the revealed patterns for the less expensive search for new materials for sensor technology are also important.
EXPERIMENTAL
Fine powders (Ssp = 0.3–0.91 m2/g) of binary compounds GaAs, ZnTe, and CdSe and (GaAs)x(CdSe)1 – x (x = 1.1, 11.5, 97.7, and 98.2 mol %) and (ZnTe)x(CdSe)1 – x (x = 12, 26, 68, and 75 mol %) solid solutions obtained according to the developed techniques [2] were studied. X-ray, microscopic, electron microscopic, Raman, IR, and Auger spectroscopic data were used to study the synthesis of solid solutions and their structure. The molar compositions of the obtained solid solutions were compared with the elemental one found from energy dispersive analysis and Auger spectra.
X-ray diffraction studies were performed on a D8 Advance Powder X-Ray spectrometer (Bruker AXS, Germany) (CuKα radiation, λ = 0.15406 nm, and T = 293 K) at large angles [3, 4] equipped with a Lynxeye position-sensitive detector. The ICDDIPDF-2 powder diffraction database and TOPAS 3.0 software (Bruker) were used to process the diffraction patterns and to refine the lattice parameters, respectively.
Microscopic studies were carried out on KN 8700 (Hilox, Japan) and Micromed “Polar-3” microscopes with a magnification of up to 7000 [5]; electron microscopic data were obtained using a JCM-5700 scanning electron microscope (SEM) equipped with a JED-2300 unit for energy dispersive analysis [6]. The Raman spectroscopic studies were carried out with a ThermoScientific DXR Smart Raman dispersion spectrometer within 39–3411 cm–1 in the backscattering geometry (spectral resolution was 2.4–4.4 cm–1) [7, 8]. The IR spectra were acquired using an FT-02 InfraLYUM Fourier spectrometer equipped with a unit for recording the total internal reflection (TIR) spectra between 400–4000 cm–1 [9, 10], whereas the Auger spectroscopic data were obtained with a Shkhuna-2 instrument. A probe electron beam with a diameter of 1 μm and energy of 3 keV was scanned into a 50 × 50 μm raster to record Auger transitions. The energy resolution of the analyzer, the pressure of residual gases in the analytical chamber, and the sputtering rate of the target material by argon ions were 0.7%, 10–7 Pa, and 1–4 nm/min, respectively [10, 11].
The surface properties of components of the GaAs–CdSe and ZnTe–CdSe systems (chemical composition of the surfaces and acid–base properties) were studied with hydrolytic adsorption (determination of the pH of the isoelectric state, pHiso) [12] and TIR IR spectroscopy [9, 10]. When hydrolytic adsorption was employed, the pH of the environment, in which the ampholytic adsorbents (amphoteric compounds) split off equal (insignificant) amounts of Н+ and ОН– ions, was found. GaAs, ZnTe, CdSe, and (GaAs)x(CdSe)1 – x, (ZnTe)x(CdSe)1 – x solid solutions with characteristic isoelectric points corresponding to the minimum solubility were used as such adsorbents. The average strength and the ratio of acidic and basic centers were found from the pHiso values.
The reproducibility and the accuracy of the experimental data were verified from parallel studies with mathematical statistics and quantitative analysis data. The Stat-2, Microsoft Excel, and Origin computer programs were used for statistical processing of the numerical values, calculation of the measurement errors, and for the construction and processing of graphical dependencies.
RESULTS AND DISCUSSION
The X-ray diffraction data [13, 14] (Tables 1 and 2) showed that substitutional solid solutions with a cubic sphalerite structure (with an excess of GaAs and ZnTe) and a hexagonal wurtzite structure (with an excess of CdSe) are formed in the GaAs–CdSe and ZnTe–CdSe systems at the specified compositions. The shift in the diffraction patterns of lines corresponding to solid solutions relative to those of binary components at a constant number, the relative position and intensity distribution of the main lines [13, 14], a smooth or linear change with the composition (within the same structure) of the lattice parameters (a and c), interplanar spacings dhkl, and X-ray density ρr of components of the GaAs–CdSe and ZnTe–CdSe systems (Tables 1 and 2) indicates this.
The electron microscopic, Raman, Auger, and IR spectroscopic data confirm that substitutional solid solutions are formed in these systems. Indeed, the average numbers of predominant particles (nav) found from SEM images (Fig. 1) vary smoothly with the composition to correlate with the relationship between the X-ray density and the composition (Fig. 2).
The Raman spectra show (Fig. 3) that the substitution of metal atoms at lattice sites leads to a decrease in the vibrational frequency and, as a result, in the intensity of Raman peaks assigned to longitudinal (LO) and transverse (TO) oscillations [7, 8]. Raman spectroscopic studies also show that the components of the ZnTe–CdSe system possess luminescence properties. The luminescence intensity increases in the following series:
When the composition of the systems is changed, the intensity of the main IR absorption bands of coordinated water (3400 and 1640 cm–1) and molecular adsorbed carbon dioxide (2440 cm–1) changes in a certain way (Fig. 4).
As already noted, the molar compositions of the solid solutions obtained were compared with the elemental one. Table 3 shows the elemental composition of components of the systems found from energy dispersive analysis. It should be noted that there is a satisfactory agreement between the elemental composition and molar composition. These results were significantly enhanced by Auger spectroscopic studies (Fig. 5 and Table 4). The atoms were found, the elements on the surfaces were identified, and the quantitative chemical composition of the surfaces was determined from the energies and the number of Auger electrons. Figure 5, for example, shows that there are clear Auger transitions on the surface of the (ZnTe)0.26(CdSe)0.74 solid solution assigned to its elements (Zn, Te, Cd, and Se). A certain energy value corresponds to each element: 50 and 900–1000, 400–500, 270–400, and 1200–1350 eV are assigned to Zn, Te, Cd, and Se, respectively (in this case, these are transitions between neighboring orbitals).
The IR spectra show the chemical compositions of the real surfaces of the components in the GaAs–CdSe and ZnTe–CdSe systems exposed to air (Fig. 4). They contain bands assigned to coordinated water (3400 and 1640 cm–1), molecular adsorbed carbon dioxide (2440 cm–1), C–H bonds (2900 cm–1), C=O (1750 cm–1), and the HO–CO2 group (1390 cm–1).
The surfaces of components of the GaAs–CdSe and ZnTe–CdSe systems exposed to air are mainly weakly acidic (except ZnTe and solid solutions with its excess): the pHiso values vary between 6.2–6.8 and 6.8–7.87 (Fig. 2). The pHiso values increase in the following series: \({\text{GaAs}} \to {{({\text{GaAs}})}_{x}}{{({\text{CdSe}})}_{{1{\kern 1pt} - {\kern 1pt} x}}} \to {\text{CdSe}}{\text{,}}\) and \({\text{CdSe}} \to {{({\text{ZnTe}})}_{x}}{{({\text{CdSe}})}_{{1{\kern 1pt} - {\kern 1pt} x}}} \to {\text{ZnTe}}\), which correlate with the increased band-gap values (ΔE) from 1.43 to 1.88 and from 1.88 to 2.20 eV.
This relationship between the surface (pHiso) and bulk (ΔE) properties is probably due to active (acid-base) centers: the fraction of ionic bonds increases with an increase in the band gap. As a result, the degree of surface hydration and the concentration of Bronsted centers (mainly OH groups) increase, so that the basicity of the surfaces increases as well (increase in pHiso). It should be noted that the acid-base properties of components of the GaAs–CdSe system correlate with the band gap and with other bulk properties: the average number of particles (nav) and the X‑ray density ρr (Fig. 2).
When the surfaces are predominantly weakly acidic, components of the systems (except ZnTe surfaces and solid solutions with its excess) can be used (especially (GaAs)x(CdSe)1 – x) to produce sensors for detecting trace impurities of basic gases (NH3 type). One can facilitate significantly the search for such materials based on correlations between the relationships pHiso = f(XCdSe), nav = f(XCdSe), and ρr = f(XCdSe) and the resulting final one pHiso = f(nav) and pHiso = f(ρr).
The influence of GaAs and ZnTe binary components in GaAs–CdSe and ZnTe–CdSe systems (in particular, on the surface acid–base properties) appeared to be opposite. The pHiso decreases with an increase in the amount of GaAs in the GaAs–CdSe system, and it increases with an increase in the amount of ZnTe in the ZnTe–CdSe system. The reason for the increase in pHiso is explained above.
CONCLUSIONS
The GaAs–CdSe and ZnTe–CdSe solid solutions obtained via special isothermal diffusion of the initial binary compounds (GaAs and CdSe, ZnTe and CdSe) and their bulk, physical, and physicochemical properties were studied with X-ray, microscopic, electron-microscopic, Raman, IR, and Auger spectroscopies.
The results of this work were used to enrich the data bank on multicomponent diamond-like semiconductors, including the luminescence and structural properties, and to certify the solid solutions obtained. They appeared to be substitutional solid solutions with a cubic sphalerite structure with an excess in the GaAs and ZnTe systems and a hexagonal wurtzite one with an excess of CdSe.
The chemical composition of the initial surfaces of the system components, which did not fundamentally differ from that of the initial surfaces of other previous diamond-like semiconductors, was found.
The surfaces of the system components (pHiso values) are weakly acidic except ZnTe surfaces and solid solutions with its excess. The binary components of the GaAs and ZnTe systems have the opposite effect on the acid-base state to decrease the pHiso in the first case and to increase it in the second. As a result, the surfaces of solid solutions of the GaAs–CdSe system possess more acidic properties compared to those of the ZnTe–CdSe solid solutions. The is probably due to active acid-base centers.
Changes in the properties with the composition and correlations between them were found. The relationships between the surface (pHiso) and bulk (nav, ρr, and ΔE) properties and the resulting direct dependences pHiso = f(nav) and pHiso = f(ρr) facilitate significantly the search for new effective materials for semiconductor gas analysis (sensor technology). System components with pHiso < 7 and especially (GaAs)x(CdSe)1 – x solid solutions are recommended to produce sensors for trace impurities of basic gases.
REFERENCES
I. A. Kirovskaya, Surface Phenomena (Omsk. Gos. Univ., Omsk, 2001) [in Russian].
I. A. Kirovskaya, Physicochemical Properties of Binary and Multicomponent Diamond-Like Semiconductors (Sib. Otd. Ross. Akad. Mauk, Novosibirsk, 2015) [in Russian].
E. F. Smyslov, Zavod. Lab., Diagn. Mater. 72 (5), 33 (2006).
S. S. Gorelik, L. N. Rastorguev, Yu. A. Skakov, X-Ray and Electron-Optical Analysis (Metallurgiya, Moscow, 1970) [in Russian].
A. Clarke and C. Eberhardt, Microscopy Techniques for Materials Science (CRC, Boca Raton, 2002; Tekhnosfera, Moscow 2007).
J. I. Goldstein, D. E. Newbury, P. Echlin, D. C. Joy, C. Fiori, and E. Lifshin, Scanning Electron Microscopy and X-Ray Microanalysis (Plenum, New York, 1981; Mir, Moscow, 1984).
M. M. Sushchinskii, Sov. Phys. Usp. 31, 181 (1988).
M. M. Sushchinskii, Raman Scattering of Light. Structure of Matter (Nauka, Moscow, 1981) [in Russian].
L. H. Little, Infrared Spectra of Adsorbed Species (London: Academic, 1966; Mir, Moscow, 1969).
I. A. Kirovskaya, Adsorption Processes (Irkutsk. Gos. Univ., Irkutsk, 1995) [in Russian].
T. A. Carlson, Photoelectron and Auger Spectroscopy (Plenum New York, 1975; Mashinostroenie, Leningrad, 1981).
L. G. Maidanovskaya, Catalytic Reactions in the Liquid Phase (Akad. Nauk Kaz. SSR, Alma-Ata, 1963) [in Russian].
I. A. Kirovskaya and M. V. Vasina, Procedia Eng. 113, 456 (2015). https://doi.org/10.1016/j.proeng.2015.07.335
I. A. Kirovskaya, E. V. Mironova, M. V. Vasina, et al., J. Phys.: Conf. Ser. 1210, 012064 (2019). https://doi.org/10.1088/1742-6596/1210/1/012064
Author information
Authors and Affiliations
Corresponding author
Additional information
Translated by A. Tulyabaev
Rights and permissions
About this article
Cite this article
Kirovskaya, I.A., Vasina, M.V., Mironova, E.V. et al. Relative Influence of Binary Components on the Bulk and Surface Properties of GaAs–CdSe and ZnTe–CdSe Solid Solutions. J. Surf. Investig. 15, 321–326 (2021). https://doi.org/10.1134/S1027451021020233
Received:
Revised:
Accepted:
Published:
Issue Date:
DOI: https://doi.org/10.1134/S1027451021020233