Abstract
Background
It is well established that the activation of the renin–angiotensin system (RAS) and the oxidative stress caused by hyperglycemia are major mediators of the development and progression of diabetic nephropathy (DN). Vitamin D may be important in maintaining podocyte health, preventing epithelial-to-mesenchymal transformation, and suppressing renin gene expression and inflammation, but its mechanism requires clarification. This study evaluated the specific mechanism of vitamin D to DN improvement.
Methods
We induced a rat model of diabetes with an intraperitoneal injection of streptozotocin (60 mg/kg). The streptozotocin-induced diabetic rats were fed normal chow for about 2 months to induce the DN model. The DN rats were then treated with irbesartan and/or calcitriol, administered intragastrically about 1 month.
Results
The rats displayed the early characteristics of DN, including increased microalbuminuria, obvious hypertrophic kidney, and a markedly increased kidney weight/bodyweight ratio. Vitamin D inhibited the compensatory increase in renin expression. Malondialdehyde, which reflects oxidative stress levels, was elevated in the DN group rats and their antioxidant capacity was significantly reduced. The irbesartan and calcitriol interventions markedly improved the renal pathology and pathophysiological changes. Irbesartan combined with vitamin D (calcitriol) more effectively ameliorated DN than irbesartan alone.
Conclusions
Vitamin D combined with angiotensin II type 1 receptor blockers exerts a synergistic effect on the treatment of DN, not only by inhibiting renin but also by reducing oxidative stress and increasing the renal antioxidant capacity.
Similar content being viewed by others
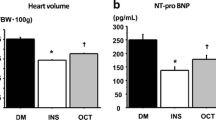
Avoid common mistakes on your manuscript.
Introduction
Diabetic nephropathy (DN) is the most common cause of endstage renal failure in the world [1, 2], and about 25–40 % of type 1 and type 2 diabetic patients develop diabetic nephropathy within 20–25 years of the onset of their diabetes [3]. DN accounts for the high levels of disability and high mortality rates in diabetic patients. Previous studies have suggested that multiple risk factors are involved in the pathogenesis of DN [4–6]. Its morphological characteristics include glomerular hypertrophy, basement membrane thickening, mesangial expansion, tubular atrophy, interstitial fibrosis, and arteriolar thickening. It is well established that the activation of the renin–angiotensin system (RAS) and the oxidative stress caused by hyperglycemia are major mediators of the development and progression of DN. Among patients with DN, microalbuminuria is a principal risk factor for progressive damage to renal function. The incidence of DN in diabetic patients whose urine albumin excretion rate is 20–200 μg/min is 10 to 20-fold higher than that in diabetic patients with normal albuminuria [7]. Therefore, the reduction of albuminuria is a major renoprotective goal in DN patients.
RAS inhibitors, including angiotensin (Ang)-converting enzyme inhibitors (ACEIs) and Ang II type 1 receptor blockers (ARBs), are widely used in current therapies for renal disease, to reduce proteinuria. Many studies have demonstrated that ACEIs and ARBs have an important protective effect against DN [5]. However, the major problem associated with these drugs is the compensatory increase in renin that arises when the feedback inhibition of renin production is disrupted by them. The increase of renin activity stimulates the conversion of Ang I and ultimately Ang II. Ang II activates NADPH oxidase, inducing oxidative stress in the kidney by increasing the generation of free radicals, specifically reactive oxygen species (ROS), which effectively reduces the efficacy of RAS inhibition. Under normal physiological circumstances, there is a balance between the generation of free oxygen radicals and the antioxidant defense mechanisms used to deactivate their toxicity. Impairment of the oxidant/antioxidant equilibrium results in oxidative stress in numerous pathological conditions, including DN, which leads to cellular damage.
1,25-Dihydroxyvitamin D3 (1,25[OH]2D3), the active hormonal metabolite of vitamin D, has been shown to function as a key negative endocrine regulator of the RAS [8]. 1,25(OH)2D3 suppresses renin gene transcription [9], so vitamin D plays an important protective role in the renal and cardiovascular systems by regulating the RAS. Recent experimental data suggest that vitamin D protects podocytes by targeting multiple pathways, including the RAS, the Wnt/β-catenin pathway, and the proapoptotic pathway [10]. Several studies have also demonstrated that vitamin D reduces oxidative stress and inflammation [11]. However, vitamin D deficiency and insufficiency are highly prevalent in individuals with diabetes, and there is an independent association between vitamin D deficiency or insufficiency and the presence of nephropathy [12]. In the kidney, vitamin D may be important for maintaining podocyte health, preventing epithelial-to-mesenchymal transformation, and suppressing renin gene expression and inflammation, but its mechanism requires clarification.
In this study, we investigated whether vitamin D improves DN by inhibiting renin and reducing oxidative stress.
Materials and methods
Animals
Normal male Sprague–Dawley rats weighing 140–180 g and normal chow were obtained from the Experimental Animal Centre of Jiangsu University of China. The rats were placed in special cages (3–4/cage) on a 12-h day/night cycle with access to food and water ad libitum, except when specified by the experimental protocol. The rats were individually housed in special metabolic cages to allow urine collection. The animals were fasted for 12 h before all experiments.
Instruments and biochemical reagents
Streptozotocin (STZ) was purchased from Sigma Company (St. Louis, MO, USA). Pentobarbital sodium was purchased from Baoman Biotechnology Company Limited (Shanghai, China). Assay kits for urine protein, malondialdehyde (MDA), total superoxide dismutase (T-SOD), total antioxidant capacity (T-AOC), catalase (CAT), and glutathione peroxidase (GSH-PX) were purchased from Nanjing Jiancheng Bioengineering Institute (Nanjing, China). TRIzol Reagent and the real-time PCR kit (Platinum® SYBR® Green qPCR SuperMix) were purchased from Invitrogen Company (Carlsbad, CA, USA). MMLV reverse transcriptase, RNase inhibitor, Taq DNA polymerase, and dNTPs were purchased from Fermentas Company (Ottawa, Canada). The RNA primers were synthesized by Shanghai R&S Biotechnology Company Limited (Shanghai, China). Other biochemical reagents were purchased from Shanghai Sangon Biotechnology Company Limited (Shanghai, China). The OneTouch Surestep® Blood Glucose Monitoring System (Johnson & Johnson Medical Company Limited) was used to monitor the blood sugar of the rats. A 722S Visible Spectrophotometer was used (Shanghai Analytical Apparatus Factory) and the DCA2000 Analyzer (Siemens Company). Real-Time PCR realplex 4 was from Eppendorf Co., Germany. Calcitriol (Rocaltrol®) was purchased from Shanghai Roche Medical Co., Ltd, and irbesartan (Aprovel®) was purchased from Hangzhou Sanofi Medical Co., Ltd.
Experimental protocol
Upon arrival in the laboratory, 50 male Sprague–Dawley rats were fed normal chow and allowed to acclimatize for 1 week. After acclimatization, the fasting plasma glucose (FPG) and random plasma glucose (RPG) levels of the rats were measured. In this study, RPG was defined as the plasma glucose measured on a non-empty stomach. The rats were then randomly divided into two groups. Animals in the normal control group (NC, n = 5) and the diabetic model group (DM, n = 45) were fed normal chow ad libitum. The rats in the DM group were administered STZ (60 mg/kg) by intraperitoneal injection, whereas the NC group received an intraperitoneal injection of citrate buffer solution. The RPG of all the rats was measured after 1 week. The standard indicator of diabetes induction is an RPG ≥16.7 mmol/l [13], and the rats that did not attain this level of glucose were excluded. The diabetic rats were then fed normal chow continuously for about 2 months. The rats were individually housed in special metabolic cages to collect 24-h urine samples, which were analyzed with the DCA2000 Analyzer to determine the rates of urine albumin/creatinine (UACR). The standard indicator of DN induction is UACR ≥30 mg/g. The screened DN rats were randomly divided into the DN control group (DN, n = 9), the DN with irbesartan treatment group (DNI, n = 6), the DN with calcitriol treatment group (DNC, n = 6), and the DN with irbesartan and calcitriol treatment group (DNIC, n = 6). Calcitriol (2.5 μg) was dissolved in 10 ml of peanut oil. The rats in the DNC and DNIC group were administered calcitriol (0.2 μg/kg) intragastrically once daily. The rats in the NC and DN group were intragastrically administered only isochoric peanut oil. Irbesartan was dispensed as a suspension (30 mg/ml) in physiological saline and was mixed well before use. The rats in the DNI and DNIC groups were administered irbesartan (50 mg/kg) intragastrically once daily. The rats in the DNIC group were treated with irbesartan and calcitriol. The interval between the intragastric administrations of the two drugs was 2 h. After treatment for 1 month, all the rats were anesthetized with an intraperitoneal injection of 1 % sodium pentobarbital (60 mg/kg bodyweight). Blood samples were collected with heart puncture, and the kidneys were quickly removed. Part of the kidney tissue was fixed in 4 % buffered paraformaldehyde solution and then cut into sections. The remaining kidney samples were immediately frozen in liquid nitrogen and stored at −70 °C until analysis.
General characteristics
The food intake and bodyweight (BW) of the rats in each group were recorded weekly. After the rats were killed, the left kidneys were isolated and weighed. The ratio of the left kidney weight to BW was calculated and expressed as a percentage. Several biochemical parameters, including FPG, RPG, UACR, urine protein, total cholesterol (TC), and triglycerides (TG) were tested. The oxidative stress index (MDA) and antioxidative stress indices, such as T-SOD, T-AOC, CAT, and GSH-PX, of the rats were determined in the sera and kidney tissues. All tests were carried out strictly according to the kit instructions.
Histopathology of the kidney
Formalin-fixed paraffin-embedded kidney cortices were processed routinely for hematoxylin and eosin staining. The size and shape of the glomeruli were observed under a light microscope. The mean values for the long diameters and short diameters of the glomeruli were calculated with the Image-Pro® Insight software. We selected 100 glomeruli from the rats in each group for measurement. The mean glomerular diameter was calculated for each group. The tissues were also processed for electron microscopy with standard techniques. Small fragments of kidney cortex tissue were excised and cut into 1 mm3 pieces, which were immediately fixed in 2.5 % glutaraldehyde and rinsed in 0.1 mol/l phosphate buffer. After fixation in 1 % osmium tetroxide, the samples were rinsed in 0.1 mol/l phosphate buffer, dehydrated in a graded series of alcohol, and embedded in pure epoxy resin. Ultrathin sections were cut with the Leica Ultracut UCT ultramicrotome (Leica Microsystems, Wetzlar, Germany), stained with uranyl acetate and lead citrate, and examined with a Hitachi H-600 transmission electron microscope (Hitachi, Japan).
Real-time RT–PCR
Total cellar RNAs extracted with TRIzol Reagent from the kidney tissues were assayed for gene expression with real-time RT–PCR. First-strand cDNAs were synthesized from 4 μg of total RNA in a 20 μl reaction using MML-V reverse transcriptase, and were used as the templates for PCR. Real-time PCR was performed with the realplex 4 system (Eppendorf), using a SYBR Green PCR reagent kit (Platinum® SYBR® Green qPCR SuperMix), as described previously [14]. β-Actin was used as the internal control. The PCR primers used in this study are listed in Table 1.
Statistical analysis
All results are expressed as mean ± SD and were analyzed with one-way ANOVA. The Student–Newman–Keuls multiple range test was then used to compare the results of two groups. P < 0.05 was considered statistically significant. All statistical analyses were performed with the SAS 8.1 software.
Results
General characteristics of the rats during the experiments
The rats in the DM group were treated with an intraperitoneal injection of STZ (60 mg/kg). Most rats displayed obvious symptoms of DM, such as polydipsia, polyuria, and polyphagia. Seven rats did not attain the standard RPG for DM of ≥16.7 mmol/l. The model of diabetes was successfully established in 84 % of rats. The diabetic rats were fed normal chow for about 2 months to induce the DN model. The bodyweights of the rats in the DM group were clearly lower than those of the rats in the normal control group. In the process of inducing the DN model, the blood sugar of the rats showed no tendency to recover spontaneously. During the DN induction process, no rats used insulin to reduce their blood sugar. The blood sugar of the DM rats remained high at all times (up to 20 mmol/l). Consequently, the rats in the DM group displayed higher mortality than the control rats. After the DM rats were fed for 2 months, all emerged with different degrees of binocular cataract, which could be seen with the naked eye. About 77 % of the surviving rats in the DM group developed DN. At that time (before treatment with calcitriol or irbesartan), the bodyweights of the rats in the NC group differed significantly from those of the rats in the DN, DNC, DNI, and DNIC groups (Table 2).
Comparison of the measured parameters in each group after treatment
The rats in DNC, DNI, and DNIC groups were treated according to the protocol. The blood sugar levels in these groups did not differ from that in the DN group, and all were maintained upon 33.3 mmol/l. However, the vigor of the rats in the DNC, DNI, and DNIC groups was clearly better than that of the rats in the DN group. No rats died in the treated groups, but three rats died in the DN group. There were no significant differences in TC among the NC, DN, DNI, DNC, and DNIC groups, but TG was clearly elevated in the DN, DNI, DNC, and DNIC groups compared with the NC group. In the DN group, the volume and weight of the kidney was clearly increased. The kidney weight/bodyweight ratio of the DN group was markedly higher than that of the NC group. After treatment with calcitriol and irbesartan, the kidney weight/bodyweight ratio decreased significantly (Fig. 1). The UACR and urine protein of rats in the DNI, DNC, and DNIC groups were significantly reduced compared with that of the rats in the DN group. Overall, the UACR and urine protein were most reduced in the DNIC group. The UACR of group DNIC, which was treated with both calcitriol and irbesartan, differed significantly from those of the DNI and DNC groups, which were treated singly with calcitriol or irbesartan (Table 3).
Comparison of left kidney weight/bodyweight in each group. NC normal control, DN diabetic nephropathy, DNI DN with irbesartan treament, DNC DN with calcitriol treatment, DNIC DN with irbesartan and calcitriol treatment. All results were expressed as the mean ± SD and analyzed using one-way ANOVA. Student–Newman–Keuls multiple-range test was used to compare results between two groups. Versus group NC, △ P < 0.05, ▲ P < 0.01
Oxidative stress parameters in rat sera
Compared with the NC group, the diabetic rats in the DN, DNI, DNC, and DNIC group displayed significantly increased MDA and decreased anti-oxidant capability parameters such as T-SOD, T-AOC, CAT and GSH-PX. However, after treatment with irbesartan or/and calcitriol in the DNI, DNC and DNIC group, these parameters clearly improved than that of rats in DN group. Especially, the improved parameters were most significant in DNIC group which treated with irbesartan and calcitriol (Table 4).
Oxidative stress parameters in rat kidney tissues
Besides testing the oxidative stress and antioxidant capacity parameters in rat sera, we also tested them in the kidney tissues of the rats. Consistent with the rat sera, the regional kidney tissues of diabetic rats in the DN, DNI, DNC, and DNIC group displayed significantly increased oxidative stress and an impaired antioxidant capacity. After treatment with irbesartan or/and calcitriol, oxidative stress improved in the kidney tissues and their anti-oxidant capacities clearly increased, especially in the groups treated with calcitriol (Table 5).
Changes in kidney histology and ultrastructure
After the kidney sections were stained with hematoxylin and eosin, the mean glomerular diameter was calculated for each group. The kidney glomerular volume in the DN rats was clearly greater than that in the NC group, but recovered after treatment (Fig. 2).
Comparison of glomerulus diameter in each group. NC normal control, DN diabetic nephropathy, DNI DN with irbesartan treament, DNC DN with calcitriol treatment, DNIC DN with irbesartan and calcitriol treatment. All results were expressed as the mean ± SD and analyzed using one-way ANOVA. Student–Newman–Keuls multiple-range test was used to compare results between two groups. Versus group NC, △ P < 0.05, ▲ P < 0.01. Versus group DN, ☆ P < 0.05, ★ P < 0.01
The ultrastructural changes in the kidneys of each group are shown in Fig. 3. Electron microscopy showed normal well-distributed foot processes and normal basilar membranes of the kidney glomeruli in the rats of the NC group. No fusion or false trophonema changes were observed in the foot processes. The basilar membranes of the kidney glomeruli were uniform in the NC group of rats. In the DN group, a greater number of foot processes was observed, which were clearly short and flat, and showed general fusion. Some foot processes had disappeared. The podocytes were swollen and the number of podocytes was significantly reduced. Increased thickness of the basilar membranes of the kidney glomeruli was observed in the DN group rats. The rats in the DNI, DNC, and DNIC groups were treated with calcitriol and/or irbesartan. The fusion of their foot processes was notably improved. The main part of the foot process could be seen clearly. The increased thickness of the basilar membranes was also significantly ameliorated. Overall, the DNIC group showed the most significant improvement in its foot processes and basilar membranes.
The mRNA expression of renin, manganese superoxide dismutase (MnSOD), glutathione peroxidase 1 (GPX1), and cytosolic CuZn-SOD (SOD1) in rat kidneys.
The mRNA expression of renin had no significant differences in the rat kidney tissues of NC, DN and DNC group. The irbesartan treatment clearly elevated the mRNA expression of rennin in the DNI and DNIC group. Compared with DNI group, the mRNA expression of renin was significantly reduced in DNIC group which treated with irbesartan and calcitriol (Fig. 4). Compared with NC group, the mRNA expression of antioxidant enzymes, such as MnSOD, GPX1, and SOD1, was significantly reduced in the DN group. However, the mRNA expression of antioxidant enzymes of rats in the DNI, DNC, and DNIC groups was significantly increased compared with that of the rats in the DN group. Overall, treatment with calcitriol in DNC and DNIC group more clearly increased the mRNA expression of these antioxidant enzymes (Fig. 5).
Renin mRNA expression of rat kidney in each group. NC normal control, DN diabetic nephropathy, DNI DN with irbesartan treament, DNC DN with calcitriol treatment, DNIC DN with irbesartan and calcitriol treatment. All results were expressed as the mean ± SD and analyzed using one-way ANOVA. Student–Newman–Keuls multiple-range test was used to compare results between two groups. Versus group NC, △ P < 0.05, ▲ P < 0.01. Versus group DN, ☆ P < 0.05, ★ P < 0.01. Versus group DNI, ◇ P < 0.05, ◆ P < 0.01. n = 3
SOD1, MnSOD, GPX1 mRNA expression of rat kidney in each group. NC normal control, DN diabetic nephropathy, DNI DN with irbesartan treament, DNC DN with calcitriol treatment, DNIC DN with irbesartan and calcitriol treatment, SOD1 cytosolic CuZn-SOD, MnSOD manganese superoxide dismutase, GPX1 glutathione peroxidase 1. All results were expressed as the mean ± SD and analyzed using one-way ANOVA. Student–Newman–Keuls multiple-range test was used to compare results between two groups. Versus group NC, △ P < 0.05, ▲ P < 0.01. Versus group DN, ☆ P < 0.05, ★ P < 0.01. Versus group DNI, ◇ P < 0.05, ◆ P < 0.01. n = 3
Discussion
Diabetic nephropathy is the main microvascular complication of diabetes. ACEIs and ARBs are widely used to treat DN because they exert renoprotective effects. However, not all DN patients benefit from ACEI or ARB therapy. One reason is the compensatory increase in renin that occurs after the ACEI- or ARB-mediated inhibition of renin production. Another reason may be that the increase in renin activity stimulates the conversion of Ang II, escalating oxidative stress in these DN patients. However, vitamin D, a renin biosynthesis inhibitor, is thought to assist ARBs to improve DN.
To clarify the protective effects of vitamin D on DN, we prepared a diabetic rat model using intraperitoneal injections of STZ. The diabetic rats were then fed normal chow to maintain their hyperglycemic state for about 2 months to induce DN. STZ can specifically damage the beta cells of islets, thus inducing hyperglycemia, although the specific mechanism of STZ action is unclear. The main mechanisms include DNA fragmentation, the induction of ROS and thus oxidative stress, and so on, which impair islet beta cells [15, 16]. In our study, the random blood sugar of rats injected intraperitoneally with 60 mg/kg STZ increased significantly. After they were fed normal chow for about 3 months, the glycemia of the rats was maintained at 33.3 mmol/l and showed no tendency to recover spontaneously. In the induced DN model, the rats displayed the early characteristics of DN, including increased microalbuminuria, obvious hypertrophic kidney, and a markedly increased kidney weight/bodyweight ratio. After treatment with irbesartan for 1 month, the microalbuminuria of the rats in the DNI group was significantly reduced and the renin mRNA expression in the kidney tissues was clearly elevated, which suggests that irbesartan dramatically improves DN. At the same time, the rats displayed the compensatory increase in renin attributable to the inhibition of renin by irbesartan. When the rats in the DNIC group were treated with irbesartan and calcitriol, the increase in renin mRNA expression was markedly reduced. As a result, the UACR was lower than that of the DNI group. The kidney glomerular diameters and ultrastructures in the DNI, DNC, and DNIC groups were improved relative to those in the DN group. These data suggest that the ARB irbesartan combined with vitamin D (calcitriol) more effectively ameliorates DN than irbesartan alone. The synergetic effects of ARBs and vitamin D are associated with the inhibition of renin by vitamin D, a biosynthesis inhibitor. These results are consistent with those of other studies [17–19]. However, the UACR and glomerular ultrastructure also improved significantly in the rats in the DNC group, treated with calcitriol only, but the expression renin was only slightly reduced in this group. This demonstrates that the inhibition of renin expression by vitamin D is not the only way it ameliorates DN. Some other mechanisms may account for the synergetic effects of vitamin D.
In DN, chronic hyperglycemia is the principal factor inducing metabolic, biochemical, and vascular abnormalities. Hyperglycemia generates further free radicals by the oxidation of glucose itself. The increased ROS induced by oxidative stress impairs the function of the vascular endothelium. It thus plays a key role in the onset and development of diabetic macrovascular and microvascular complications [20, 21]. Clinical observations have shown that the incidence of macroalbuminuric and coronary artery diseases in diabetic patients with Gilbert syndrome (with hyperbilirubinemia) is about 20 % of that in diabetic patients without Gilbert syndrome. Therefore, it is clear that bilirubin has an obvious antioxidative effect. The proteinuria and nephridial tissue pathology of db/db DN mice improved significantly when they were treated with biliverdin, a precursor of bilirubin [22]. In transgenic diabetic mice, the overexpression of SOD can prohibit the development of DN [23]. Telmisartan alleviates early-phase DN by upregulating the expression of SOD to relieve the oxidative stress induced by the hyperglycemia of DN [24]. These studies demonstrate the importance of alleviating oxidative stress in the treatment of DN. Accordingly, inhibiting the overproduction of ROS induced by hyperglycemia may be an effective method of preventing the onset of diabetic complications. However, strategies that reduce oxidative stress are so far very limited. Maintaining satisfactory blood glucose, blood pressure, blood fat, and lifestyle factors still constitute the accepted treatment options. The developmental and metabolic disorders of glucose and lipid metabolism in diabetes can induce the overproduction of mitochondrial superoxide in vascular endothelial cells, which will activate many signaling pathways, and increase ROS in cells. This induces dysfunction of the vascular endothelium, stimulating the expression of various kinds of proinflammatory genes. Although blood glucose can be controlled well, the chronic secondary effects persist for a long time with the persistent expression of various proinflammatory genes. This is called the “hyperglycemia memory effect” [23, 25]. Therefore, it is very important for the prevention and treatment of DN to find a suitable treatment of oxidative stress.
Vitamin D is the major regulatory factor of calcium and phosphorus metabolism. However, the wide distribution vitamin D receptors throughout the body suggest that vitamin D functions beyond the regulation of calcium and phosphorus metabolism. It plays important multisystemic roles, including in the immune system, cardiovascular system, genital system, glycolipid metabolism, etc. Several studies have shown that vitamin D can protect islet beta cells by inducing anti-inflammatory factors interleukin 1β and interferon γ. Vitamin D also increases the levels of the SOD, CAT and GPX enzymes and reduces lipid peroxidation [26, 27]. Treatment with vitamin D alone or combined with other immunosuppressive agents can prevent the onset of type 1 diabetes [28, 29]. Many studies have shown that vitamin D actively affects DN through multiple pathways. It is easier to cause renal impairment by inducing hyperglycemia in mice with a defect in the vitamin D receptor than in those with no such defect. Diabetic mice in which the vitamin D receptor gene is knocked out develop more serious albuminuria and glomerulosclerosis than do mice with a normal receptor, which suggests that vitamin D exerts protective effects against DN [14, 30]. Unfortunately, many people, especially diabetic patients, show varying degrees of vitamin D deficiency [31–33]. Therefore, vitamin D supplementation is an appropriate therapy for diabetic and DN patients. Our study indicates that vitamin D plays an important role in relieving oxidative stress in DN. Previous research has indicated that diabetes induced with STZ is associated with chronic endogenous oxidative stress. In the first four weeks of STZ-induced diabetes, hyperoxide is significantly elevated [16]. In our study, RPG and MDA, which reflect the levels of oxidative stress, were increased in the DN group and antioxidant levels were significantly reduced, which suggests that oxidative stress and a reduced antioxidant capacity are important factors in the onset of DN. Although the DN rats in group DNC were treated with calcitriol only, the total antioxidant capacity of these rats was markedly increased and their MDA levels were significantly reduced. As a result, the UACR and glomerular ultrastructure of these rats improved markedly. However, the blood glucose and blood lipids of the rats in the DNC group did not differ from those of the group DN rats. This suggests that the renal protective effects of vitamin D are attributable to improvements in oxidative stress, and not to reductions in blood glucose or blood lipids. Further molecular analyses showed that calcitriol strikingly increased the mRNA expression of some important antioxidants in the kidney, including MnSOD, GPX1, and SOD1. This demonstrates that vitamin D mainly exerts a protective effect against DN by increasing the renal antioxidant capacity, thus reducing oxidative stress levels. Therefore, when the DN rats in the DNIC group were treated with irbesartan combined with calcitriol, the mRNA expression of antioxidants in the kidney increased and the antioxidant levels in the blood and kidney were clearly elevated. However, the expression of renin mRNA clearly decreased relative to that in the DNI group. Finally, among the DNI, DNC, and DNIC groups, the rats in the DNIC group showed the greatest reduction in urinary protein. These data demonstrate that vitamin D combined with ARBs exerts a synergistic effect in the treatment of DN, not only by inhibiting renin expression but also by relieving oxidative stress and increasing the renal antioxidant capacity.
In conclusion, vitamin D significantly reduced the albuminuria in DN rats. The effect of vitamin D in relieving oxidative stress is an important mechanism. Vitamin D combined with ARBs improves DN in rats by inhibiting renin expression and relieving oxidative stress. In this study, to avoid the interference of fluctuations in blood glucose, we used no treatment to reduce the hyperglycemia in the rats. Throughout the whole experiment, the DN rats were always maintained in a state of high oxidative stress induced by persistent hyperglycemia. Therefore, we predict that treatment with vitamin D combined with ARBs would have had an even stronger renoprotective effect on DN if we had used hypoglycemic agents to reduce the blood glucose levels of the rats, which would have further reduced the oxygen radicals produced.
References
Ritz E, Rychlik I, Locatelli F, Halimi S (1999) End-stage renal failure in type 2 diabetes: a medical catastrophe of worldwide dimensions. Am J Kidney Dis 34:795–808
Gross JL, de Azevedo MJ, Silveiro SP, Canani LH, Caramori ML et al (2005) Diabetic nephropathy: diagnosis, prevention, and treatment. Diabetes Care 28:164–176
Remuzzi G, Schieppati A, Ruggenenti P (2002) Clinical practice. Nephropathy in patients with type 2 diabetes. N Engl J Med 346:1145–1151
Andersen S, Tarnow L, Rossing P, Hansen BV, Parving HH (2000) Renoprotective effects of angiotensin II receptor blockade in type 1 diabetic patients with diabetic nephropathy. Kidney Int 57:601–606
Jennings DL, Kalus JS, Coleman CI, Manierski C, Yee J (2007) Combination therapy with an ACE inhibitor and an angiotensin receptor blocker for diabetic nephropathy: a meta-analysis. Diabet Med 24:486–493
Forbes JM, Coughlan MT, Cooper ME (2008) Oxidative stress as a major culprit in kidney disease in diabetes. Diabetes 57:1446–1454
Parving HH, Lehnert H, Bröchner-Mortensen J, Gomis R, Andersen S et al (2001) The effect of irbesartan on the development of diabetic nephropathy in patients with type 2 diabetes. N Engl J Med 345:870–878
Li YC, Kong J, Wei M, Chen ZF, Liu SQ et al (2002) 1,25-Dihydroxyvitamin D3 is a negative endocrine regulator of the renin-angiotensin system. J Clin Invest 110:229–238
Yuan W, Pan W, Kong J, Zheng W, Szeto FL et al (2007) 1,25-Dihydroxyvitamin D3 Suppresses renin gene transcription by blocking the activity of the cyclic AMP response element in the renin gene promoter. J Biol Chem 282:29821–29830
Li YC (2011) Podocytes as target of vitamin D. Curr Diabetes Rev 7:35–40
Izquierdo MJ, Cavia M, Muñiz P, de Francisco AL, Arias M et al (2012) Paricalcitol reduces oxidative stress and inflammation in hemodialysis patients. BMC Nephrol 13:159
Diaz VA, Mainous AG, Carek PJ, Wessell AM, Everett CJ (2009) The association of vitamin D deficiency and insufficiency with diabetic nephropathy: implications for health disparities. J Am Board Fam Med 22:521–527
Zhou QX, Liu F, Zhang JS, Lu JG, Gu ZL et al (2013) Effects of triterpenic acid from Prunella vulgaris L. on glycemia and pancreas in rat model of streptozotozin diabetes. Chin Med J (Engl) 126:1647–1653
Zhang Z, Sun L, Wang Y, Ning G, Minto AW et al (2008) Renoprotective role of the vitamin D receptor in diabetic nephropathy. Kidney Int 73:163–171
Montilla P, Barcos M, Munoz MC, Bujalance I, Munoz-Castaneda JR et al (2005) Red wine prevents brain oxidative stress and nephropathy in streptozotocin-induced diabetic rats. J Biochem Mol Biol 38:539–544
Raza H, Prabu SK, John A, Avadhani NG (2011) Impaired mitochondrial respiratory functions and oxidative stress in streptozotocin-induced diabetic rats. Int J Mol Sci 12:3133–3147
Zhang Z, Zhang Y, Ning G, Deb DK, Kong J et al (2008) Combination therapy with AT1 blocker and vitamin D analog markedly ameliorates diabetic nephropathy: blockade of compensatory renin increase. Proc Natl Acad Sci USA 105:15896–15901
Zhang Y, Deb DK, Kong J, Ning G, Wang Y et al (2009) Long-term therapeutic effect of vitamin D analog doxercalciferol on diabetic nephropathy: strong synergism with AT1 receptor antagonist. Am J Physiol Renal Physiol 297:F791–F801
Ohara I, Tanimoto M, Gohda T, Yamazaki T, Hagiwara S et al (2011) Effect of combination therapy with angiotensin receptor blocker and 1,25-dihydroxyvitamin D(3) in type 2 diabetic nephropathy in KK-A(y)/Ta mice. Nephron Exp Nephrol 117:e124–e132
Cetinkalp S, Delen Y, Karadeniz M, Yüce G, Yilmaz C (2009) The effect of 1alpha, 25(OH)2D3 vitamin over oxidative stress and biochemical parameters in rats where Type 1 diabetes is formed by streptozotocin. J Diabetes Complications 23:401–408
Singh DK, Winocour P, Farrington K (2011) Oxidative stress in early diabetic nephropathy: fueling the fire. Nat Rev Endocrinol 7:176–184
Takayanagi R, Inoguchi T, Ohnaka K (2011) Clinical and experimental evidence for oxidative stress as an exacerbating factor of diabetes mellitus. J Clin Biochem Nutr 48:72–77
Giacco F, Brownlee M (2010) Oxidative stress and diabetic complications. Circ Res 107:1058–1070
Fujita H, Fujishima H, Morii T, Sakamoto T, Komatsu K et al (2012) Modulation of renal superoxide dismutase by telmisartan therapy in C57BL/6-Ins2(Akita) diabetic mice. Hypertens Res 35:213–220
Lee WC, Chen HC, Wang CY, Lin PY, Ou TT et al (2010) Cilostazol ameliorates nephropathy in type 1 diabetic rats involving improvement in oxidative stress and regulation of TGF-Beta and NF-kappaB. Biosci Biotechnol Biochem 74:1355–1361
Hamden K, Carreau S, Jamoussi K, Miladi S, Lajmi S et al (2009) 1Alpha,25 dihydroxyvitamin D3: therapeutic and preventive effects against oxidative stress, hepatic, pancreatic and renal injury in alloxan-induced diabetes in rats. J Nutr Sci Vitaminol (Tokyo) 55:215–222
Del Pino-Montes J, Benito GE, Fernández-Salazar MP, Coveñas R, Calvo JJ et al (2004) Calcitriol improves streptozotocin-induced diabetes and recovers bone mineral density in diabetic rats. Calcif Tissue Int 75:526–532
Baeke F, Van Belle TL, Takiishi T, Ding L, Korf H et al (2012) Low doses of anti-CD3, ciclosporin A and the vitamin D analogue, TX527, synergise to delay recurrence of autoimmune diabetes in an islet-transplanted NOD mouse model of diabetes. Diabetologia 55:2723–2732
Skyler JS (2013) Primary and secondary prevention of Type 1 diabetes. Diabet Med 30:161–169
Deb DK, Sun T, Wong KE, Zhang Z, Ning G et al (2010) Combined vitamin D analog and AT1 receptor antagonist synergistically block the development of kidney disease in a model of type 2 diabetes. Kidney Int 77:1000–1009
Esteghamati A, Aryan Z, Esteghamati A, Nakhjavani M (2014) Differences in vitamin D concentration between metabolically healthy and unhealthy obese adults: Associations with inflammatory and cardiometabolic markers in 4391 subjects. Diabetes Metab 40:347–355
Raab J, Giannopoulou EZ, Schneider S, Warncke K, Krasmann M et al (2014) Prevalence of vitamin D deficiency in pre-type 1 diabetes and its association with disease progression. Diabetologia 57:902–908
Al-Daghri NM, Al-Attas OS, Alokail MS, Alkharfy KM, Yakout SM et al (2014) Lower vitamin D status is more common among Saudi adults with diabetes mellitus type 1 than in non-diabetics. BMC Public Health 14:153
Acknowledgments
This study was supported by the Social and Science Foundation of Changzhou, Jiangsu Province, China (CS20092021).
Author information
Authors and Affiliations
Corresponding author
Ethics declarations
Conflict of interest
There is no conflict of interest to be reported for this manuscript.
Ethical approval
All procedures performed in studies involving animals were approved by the Animal Ethics Committee of Changzhou Second People’s Hospital affiliated to Nanjing Medical University and carried out in accordance with the Guideline on the Humane Treatment of Laboratory Animals (China).
Informed consent
No informed consent.
Additional information
X. Deng and J. Cheng contributed equally to this work.
Rights and permissions
About this article
Cite this article
Deng, X., Cheng, J. & Shen, M. Vitamin D improves diabetic nephropathy in rats by inhibiting renin and relieving oxidative stress. J Endocrinol Invest 39, 657–666 (2016). https://doi.org/10.1007/s40618-015-0414-4
Received:
Accepted:
Published:
Issue Date:
DOI: https://doi.org/10.1007/s40618-015-0414-4