Abstract
Glaucoma is a chronic eye disease in which the pressure inside the eye increases and leads to damage to the optic nerve, and eventually causes blindness. In this disease, it is often necessary to use a multi-drug treatment system. There is a fixed combination of timolol maleate and brimonidine tartrate among the combination drugs in glaucoma treatment. Liposomes are one of the most important targeted drug delivery systems to eye tissue, which leads to improved drug permeability and durability in ocular tissue. In this study, thin layer hydration was used to make liposomal formulations containing timolol maleate (TM) and brimonidine tartrate (BT). After the necessary evaluations, one of the eight initial formulations was selected as an optimization formulation. Then, characteristics such as drug loading percentage, particle size, pH, zeta potential, and drug release were performed on the optimized formulation. The study of reducing intraocular pressure was performed on the optimized formulation. This study in total was performed on 18 rabbits in three groups. Hydroxypropyl methylcellulose (HPMC) polymer was injected into the anterior chamber to experimental induce glaucoma. The selected formulation was within the acceptable range of ocular products in terms of physical properties. HPMC polymer injection successfully induced glaucoma in the animal model, resulting in a 79% increase in intraocular pressure. The results showed that the liposomal formulation significantly reduced the intraocular pressure compared to the simple formulation of the aqueous solution, and both formulations were able to significantly reduce the intraocular pressure compared to the control group (P < 0.001). The results also showed that liposomal formulation has a therapeutic effect in reducing intraocular pressure. It seems that the selected liposomal formulation made by thin layer hydration can act as a suitable drug carrier to increase the effectiveness of the fixed combination of timolol maleate and brimonidine tartrate and be proposed as a new drug formulation for targeted and controlled drug delivery in the treatment of glaucoma.
Graphical Abstract

Similar content being viewed by others
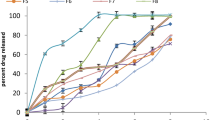
Avoid common mistakes on your manuscript.
Introduction
Glaucoma is a chronic eye disease that is associated with progressive optic neuropathies and degeneration of retina ganglion cells. During this disease, the intraocular pressure (IOP) becomes so high that the optic nerve is damaged and eventually leading to ganglion cells’ death [1]. Pathophysiology of glaucoma involves structures in both the anterior and posterior segments of the eye. The main and important pathophysiological results of glaucoma include the following: loss of neuronal and axonal architecture, activation of glial cells, changes in ocular blood flow, and tissue remodeling. According to global statistics, there are about 37 million blind people globally, 15 to 20% of whom have lost sight due to glaucoma. Studies show that in 2010, nearly 60.5 million people worldwide were diagnosed with glaucoma [2]. It is estimated that by 2040, this number will reach 100 million people [3]. Visual damage caused by glaucoma is not reversible, but with timely diagnosis and effective treatment, the disease’s progressive damage and destruction caused by the disease can be prevented and thus lead to preserving the patient's remaining vision [4]. For ocular diseases, ophthalmic drug delivery by the topical route is considered to be the most appropriate treatment route because the blood–ocular barrier does not allow effective drug delivery to ocular tissue in systemic administration [5]. However, eye drops alone have < 5% corneal bioavailability due to non-productive absorption in the conjunctiva and the nasal cavity, rapid tear turnover, a rapid clearance mechanism, and low permeability of corneal epithelium [6]. An important factor in maintaining adequate IOP control is the patient’s adherence to the prescribed treatment. Low compliance greatly diminishes drug efficacy, increases healthcare costs, and often aggravates health problems [7]. According to studies, the initial monotherapy fails to control IOP within the first 2 years of treatment in about 50% of glaucoma patients. Thus to reach the target IOP, it is often necessary to use multiple drugs [8,9,10]. Studies show that patients using two concomitant drugs often skip one drug in a few days.
Furthermore, patients do not always allow adequate time to absorb their first drop instillation before the second drug is administered [11, 12]. For patients who need multiple drug regimens to control their IOP, using a fixed combination of two medications may improve compliance and better follow the protocols of multiple drug treatment prescribed by reducing the time required to administer drops, the number of drops, and the frequency of drug administration. Additionally, it reduces the concentration of preservatives in the eye by administering eye products [13, 14]. Combigan® is the brand name of a combination of brimonidine/timolol in solution eye drop form in the market [15]. Clinical studies found that brimonidine/timolol fixed combination is more effective in lowering IOP than any of its components [16, 17] and has better therapeutic efficacy than other combinations, such as dorzolamide/timolol [18]. Timolol maleate (TM) is a non-selective β-adrenergic receptor antagonist that inhibits this receptor in the epithelium ciliary body and caused decreased secretion of aqueous humor. Brimonidine tartrate (BT) is an α-agonist that caused decreased aqueous humor production and increases its exiting. Decreasing the secretion of aqueous humor or increasing its exiting for the canal leads to a reduction in IOP [19,20,21]. Topical ophthalmic drug delivery has always been an important challenge in the pharmaceutical industry due to the eye’s physiological barrier (different layers of the cornea, sclera, and retina). In recent years, research has focused on developing new dosage forms to achieve higher drug levels in intraocular tissues. Recent advances in nanotechnology, especially liposomes, offer a great opportunity to increase drug release duration in the eye [22, 23]. Liposomes are vesicles with a phospholipid bilayer membrane that is among the nano-carriers in ocular drug delivery. Due to their phospholipid membrane, liposomes can adhere to the surface of tissue such as the cornea, and gradually release the drug on the eye’s surface, thus increasing the drug’s shelf life in the eye. Other benefits of liposomes include low side effects, biocompatibility, and drug protection against degradation [23]. In the present study, liposomal vesicles were designed to sustain the delivery of a beta-blocker (TM) and an α2-adrenergic agonist (BT) simultaneously and achieve the desired IOP-lowering better effect. Both BT and TM-loaded liposomal vesicles were prepared by the thin-film hydration method. To our knowledge, this is the first study of the simultaneous delivery of BT and TM from liposomal eye drops for the treatment of glaucoma.
Materials and methods
Materials
The following materials were used in this study: timolol maleate and brimonidine tartrate obtained from Sinadarou pharmaceutical company (Iran). Cholesterol and stearylamine were obtained from Sigma-Aldrich (Germany). Chloroform, methanol, and egg lecithin PC (phospholipid 90G®) were obtained from Merck (Germany). Hydroxypropyl methylcellulose (K 15000) was obtained from the Oxichemistry company (Iran). A dialysis tubing cellulose membrane was obtained from Sigma-Aldrich (Germany). All other chemicals were of the highest grade commercially available.
Method
Preparation of TM/BT-liposomes
In the present study, eight initial formulations of TM and BT were based on three variables (Table 1) prepared by the film hydration method [24]. Variables were chosen based on a preliminary study. All the lipid compounds (including cholesterol and egg lecithin) and stearylamine of the formulation (as per the formula given in Table 2) were taken in a round-bottom flask dissolved in 100 mL chloroform–methanol solution (3:1 v/v). The organic solution was then evaporated in a rotary evaporator (EV311, Lab-Tech, Germany) at 55 °C under reduced pressure at 120 rpm, forming a thin lipid film on the inside of a round bottom evaporator flask. The thin lipid film obtained was maintained in a desiccator for 24 h to ensure the complete removal of solvents. The dried lipid film obtained was hydrated with an aqueous phase of phosphate buffer (pH 6.4) containing the 0.5% (w/v) TM, 0.2% (w/v) BT, and O.25% (w/v) polymer HPMC. The flask was shaken for 5 min to get liposomal formulations. Liposomal formulations were named LF1 to LF8. After that, the stable colloidal suspension was then sonicated at 80% amplitude with a pulse of 0.5 cycles per second for 5 min using a bath sonicator (T710, Elma, Germany) to form smaller vesicles and make the extrusion process that followed more straightforward. The size of the vesicles was analyzed by Nano Zetasizer (Malvern, Nano ZS, UK) after sonication. After reviewing the results, a selected liposomal formulation (LFs) was designed and created.
Chromatographic conditions
Simultaneous analysis of brimonidine tartrate and timolol maleate was performed by HPLC technique connected to UV/Visible detector. Fifty microliters of sample solutions was analyzed at two fixed wavelengths (254 nm for brimonidine tartrate and 300 nm for timolol maleate). Mobile phase is composed of ammonium acetate (pH 5)-methanol (40:60 v/v) with a flow rate of 1.3 ml/min. Cyano column (250 × 4.6 mm, 5 µl) at 25 °C was used for drug separation [25].
Atomic force microscopy (AFM)
First, the sample of selective liposomal formula (LFs) was diluted 1:400 with deionized water, and after being placed on the slide, it was dried for 24 h at room temperature; then, the morphology of LFs was studied using NanoWizard® II AFM (JPK, Germany).
Determination of size and shape
The following parameters were measured: the mean particle size, polydispersity index (PDI), and zeta potential of the liposomes by Nano Zetasizer (Nano ZS, Malvern, UK).
Entrapment efficiency of TM/BT-liposomal
Entrapment efficiency (EE%) of timolol maleate and brimonidine tartrate in the liposomal vesicles was determined as follows: after sonication, 1 mL of the vesicle suspension was taken in a 1-mL microcentrifuge tube, and centrifuged at 18,000 rpm for 30 min at 4 °C in a cold centrifuge (Clements 2000, Germany) to get a yellow sediment deposited. To the sediment, 500 μL of chloroform (the liposomes were broken in organic solvent) was added and vortexed thoroughly for 5 min to ensure that the vesicles were lysed completely. One milliliter phosphate buffer (pH 6.4) was added and vortexed for 5 min (the drugs are highly soluble in water). Afterward, samples were centrifuged at 15,000 rpm for 15 min at 25 °C (to separate the organic and aqueous phases). A clear solution containing the drug is obtained after separating the aqueous phase and passing it through a syringe filter (0.45 µm). Then, 500 μl of clear solution was dilluted (1: 5) with phosphate buffer, and was analyzed for free TM and BT using a UV spectrophotometer (Biochorm, England) Respectively at 297 and 320 nm. EE% was calculated as follows [26]:
\(\mathrm{EE}\ \%= \frac{\begin{array}{c}\mathbf{Entraped\ drug}\\ (\frac{\mathbf{m}\mathbf{g}}{\mathbf{m}\mathbf{l}})\end{array}}{\begin{array}{c}\mathbf{total\ drug\ added}\\ (\frac{\mathbf{m}\mathbf{g}}{\mathbf{m}\mathbf{l}})\end{array}}\times 100\)
In vitro drugs release study
Three microliters of the liposomal suspension was placed on one side of the dialysis membrane in a Franz diffusion cell. The other side of the membrane was in continuous contact with the dissolution medium included 37 mL phosphate buffer (pH 6.4). The entire dissolution assembly was placed on a magnetic stirrer and stirred at 100 rpm at a temperature of 37 °C. Aliquots (2 mL) of dissolution medium were withdrawn at different time intervals (30 min, 60 min, 2 h, 4 h, 6 h, 9 h, 12 h) and replaced with fresh dissolution medium. Whenever the sample was withdrawn, an equal volume of fresh dissolution medium was added to maintain the constant volume. Drug concentration in the dissolution medium was determined by UV spectrophotometric method at 297 nm (for TM) and 320 nm (for BT) [27]. Then to find the mechanism of drugs release from optimal liposomal formulation, the data were evaluated according to zero-order (cumulative amount of drug released vs time), first-order (log cumulative percentage of drug remaining vs time), and Higuchi’s (cumulative percentage of drug released vs square root of time) pattern [28].
Animal studies
A total of 36 adult male rabbits (weight, 2.5–3 kg) were used for the study. All animals were housed at a controlled temperature (22 ± 2 °C) and humidity (55 ± 5%), with a 12/12 h light–dark cycle, and were given access to food and water. The animal experiments were conducted in full compliance with the regulatory principles of the ethics committee of Ahvaz Jundishapur University of Medical Sciences.
In vivo glaucoma induction method
For this purpose, after anesthesia of rabbits by intramuscular ketamine (35 mg/kg) and xylazine (5 mg/kg), and also corneal anesthesia was performed by using topical 0.5% tetracaine eye drops, 0.2 mL of the solution of HPMC 2%(W/V) was injected under sterile conditions into the anterior chamber. Injection in the anterior chamber was performed only in the right eye of every rabbit in this study. A single dose of topical 0.3% ciprofloxacin eye drop was used to prevent eye infection after injection of HPMC 2% in the eye. IOP was measured before HPMC 2% injection and 24 h after injection. A significant increase in IOP after injection was considered high ocular pressure and acute glaucoma [29].
In vivo intraocular pressure lowering activity
Intraocular pressure (IOP) of both eyes was measured using an IOPEN® tonometer every day at 8 am [30]. All IOP measurements were carried out by the ophthalmologist using the same tonometer. Rabbits that showed a consistent difference in IOP between the left and the right eye during baseline measurements, or any sign of eye stimulation, were excluded from the study. Thirty-six rabbits were divided into 6 groups. The samples (TM/BT-loaded liposomes(, TM-loaded liposomes (TM control), BT-loaded liposomes (BT control), aqueous solutions of TM/BT (aqueous control), drug-free liposomal formulation (Blank control), and the group without any treatment (negative control) were instilled into the right eyes (one drop, per turn). Then, the eye was kept closed for 1 min (to prevent wasting medicine). During the study, the left eye did not receive any intervention (considered a negative control). All formulations were used twice a day. We measured IOP before injection in the AC, 24 h after, and 2 h after each intervention (27, 41, 51, 65, 75, 89, 99, 113, 123, and 137 h after starting the injection in the right eye AC). The end of the study in each group was when eye pressure returned to baseline. It should be noted that the interval between pressure measurements in the morning to evening was 10 h and from evening to morning was 16 h.
Data were expressed as mean (mean ± SD) up to two decimal places.
Change in IOP (ΔIOP) is expressed as IOP right eye – IOP left eye.
Ocular tolerability
The Draize test was used to evaluate the potential ocular irritancy of TM/BT-loaded liposomes compared to placebo liposomes using a slit-lamp. Fifty microliters of each formulation was topically administered in the left eye every 30 min for 4 h and the right eyes were treated with sterile water as control. The congestion, redness, and swelling of the conjunctiva were scored and graded from 0 to 4. The grade was performed at 10 min, 4 h, and 12 h after the last treatment [31].
Stability study
In the present work, a stability study was carried out for selected formulations of LFs. Three formulations of LFs were stored at room temperature and under refrigeration (2–8 °C) for 1, 3, and 5 months, and the formulations were then evaluated for the drug content and particle size [32].
Statistical analysis
All the experimental data were subjected to statistical analysis, using the IBM® SPSS® Statistics 26 followed by Turkey’s test. P < 0.001 was considered to be statistically significant. All tests were carried out in triplicate, and data were reported as mean ± SDs.
Results
Characterization of TM/BT-liposomes
The particle size for TM/BT-liposomes was less than 214.53 ± 4.43 nm (Table 2), which is a suitable size considering that the recommended particle size in ophthalmic products is less than 10 μm. The size of a particle, in ophthalmic, plays a vital role in the irritation potential of the formulation and bioavailability. Hence, it is recommended that the particle size of ophthalmic preparation be less than 10 μm to minimize irritation to the eye [33, 34]. A validated HPLC method has been used for liposome characterization that demonstrated valuable retention time for TM (4.3 min) and BT (6.9 min) in chromatograms. The results showed that the EE% of TM and BT in TM/BT-liposomes was less than 34.36 ± 2.04% and 39.36 ± 1.66%, respectively (Table 2). Also, the EE% of TM in TM-liposomes and BT in BT-liposomes was less than 48.33% and 56.8%, respectively. pH and zeta potential values of TM/BT-liposomes were 6.22 ± 0.02 and + 12.3 ± 0.12 mv, respectively (Table 2).
Characterization of selected liposomal formulation for animal studies
The optimal TM/BT-liposome was selected based on the lowest particle size, suitable zeta potential, and highest drug entrapment and contained a lipid molar ratio of 0.75:1 (cholesterol: lecithin), 7.5 mL phosphate buffer, and 0.275 μm stearylamine (Table 3). The median values of variable amounts were selected to prepare an optimized formulation. Regression analysis showed that there is a significant and direct relationship between the variables of X1 (P < 0.045) and X2 (P < 0.012) with particle size. Given that the optimized formulation should have a minimum particle size for better penetration into the cornea and a maximum drug loading, and given that the effect of X2 on the particle size and drug loading is an increasing effect, so the average between the upper and lower volume levels of the aqueous phase volume was selected as the volume of the aqueous phase in the optimized formulation. Also, considering that the molar ratio of X1 on the particle size is an increasing effect and no effect was observed on the drug loading, so the amount of this variable in the optimized formulation was selected as the average between the high and low levels of this variable. Although we could use the low level of this variable in making the optimal formulation, we considered that increasing the ratio of cholesterol to lecithin is effective in the stability of the formulation and drug release, so it was decided to use the average of this variable. The effect of X3 on particle size and drug loading was not significant, but it was important in terms of creating a positive electric charge and interaction with the cornea, so the mean of this variable was used in the selected formulation.
AFM image
The AFM images show that all the vesicles were spherical, multilamellar, and have a distinct spherical shape surrounded by thin, evenly distributed coats (Fig. 1).
In vitro release of TM/BT-liposomes and determination of drug release kinetics
The comparative in vitro drug release profile is summarized in Fig. 2 for TM/BT-liposomes and TM/BT-aqueous solution formulation. It was observed that aqueous solution released > 95% of drugs within 2 h, while LFs showed 70.67 ± 5.87% of TM and 58.52 ± 4.9% of BT release in 12 h. The results of in vitro drug release profile of formulations showed that TM/BT-liposomes provide the prolonged release of drugs compared to aqueous solution formulation (12 h vs 2 h). Results also showed a slow and prolonged release of both drugs from TM/BT-liposomes and for TM followed first-order kinetics and BT followed zero-order kinetics (r2 values were nearer to 1 with the first-order for TM and zero-order for BT compared to another release kinetic). Therefore, our result showed that TM and BT’s release profile was dependent and independent of the concentration of the drug entrapped, respectively (Fig. 2).
In vivo glaucoma induction
The mean IOP of the right eye in a total of 36 rabbits studied before injection of HPMC polymer into the anterior chamber (AC) was 16.8 ± 0.6 mmHg, and 24 h after polymer injection was equal to 21.8 ± 0.53 mmHg (P < 0.001). Therefore, the intervention started 24 h after polymer injection when the intraocular pressure was significantly increased. In the negative control group, before the injection of HPMC polymer into the AC space, the mean right eye pressure was 16.73 ± 0.75 mmHg and at its highest value (79 h after polymer injection) was 29.9 ± 0.48 mmHg arrived (Fig. 3). Therefore, it can be concluded that 0.2 mL injection of HPMC 2% (w/v) in AC space due to a 79% increase in IOP for 4.5–5 days is a suitable method for glaucoma induction (Fig. 3).
Magnitude and duration of IOP reduction by TM/BT-liposomes
The results of this study showed that in the group receiving TM/BT-liposomes compared with the control group, 2 h after the first intervention (27 h after injection of polymer in right eye) to 2 h after the seventh intervention (105 h after injection of polymer in right eye), the IOP of right eye decreased significantly (P < 0.001). The highest reduction in IOP in the group receiving TM/BT-liposomes compared to the control group was 2 h after the seventh intervention (− 8.4 ± 0.35 mmHg). In the group receiving the aqueous solution formulation of TM/BT compared to the control group, from 2 h after the first intervention (27 h after polymer injection in right eye) to 2 h after the eighth intervention (121 h after polymer injection in right eye), the IOP of right eye decreased significantly (P < 0.001). The highest reduction in right eye IOP between these two groups was 2 h after the eighth intervention (− 5.3 ± 0.28 mmHg). In the group receiving TM/BT-liposomes compared to the group receiving an aqueous solution of drugs, 2 h after the first intervention, the difference in IOP in both groups was meaningful (P < 0.05). Groups TM-liposomes and BT-liposomes indicated similar patterns such as TM/BT-liposomes but with a lower decrease in IOP significantly (P < 0.05). Groups receiving TM-liposomes and BT-liposomes decreased IOP more than the group receiving the aqueous solution formulation of TM/BT from 2 h after the first intervention (27 h after polymer injection in right eye) to 2 h after the eighth intervention (121 h after polymer injection in right eye). However, after the third intervention and until the IOP returned to baseline in the TM/BT-liposomes group, this group’s IOP reduction rate was significantly more significant than the group receiving the aqueous solution formulation (P < 0.001). Finally, by comparing the blank and control groups, it was found that there was no significant difference between the two groups during the study (P > 0.05). This indicates that the components of the selected liposomal formulation (except for the active ingredient) do not independently play a role in reducing IOP (Tables 4 and 5) (Figs. 3 and 4).
Eye irritancy evaluation
Eye examination revealed mild redness of the eyes in one of the rabbits treated with TM/BT-liposomes, and one of them in the group received the sterile water 24 h after application. Nevertheless, this condition was transient. Mild redness of the eyes disappeared at the end of the day. Other local adverse effects, including corneal erosion, corneal edema, and inflammatory signs in the anterior chamber, were not observed throughout the experiment. The results showed no significant difference between right and left eye scores. All scores for both eyes were between 0 and 0.5 at 10 min, 4 h, and 12 h.
Stability
The reduction rate of EE% at 4–8 °C after 5 months was 7.78% for TM and 3.79% for BT (Tab 5). Also, the reduction of EE% after 5 months of storage at 25 °C was 28.37% for TM and 17.16% for BT. Therefore, the stability of liposomal formulation in terms of drug leakage at 4–8 °C is better than 25 °C (P < 0.001). The particle size of liposome vesicles (LV) also increased during 5 months of storage at 4–8 °C but was still within acceptable limits for ocular topical formulation [35]. This increase in particle size was meaningless compared to the increase in particle size following the formulations’ storage at 25 °C (P < 0.05) (Table 6).
Discussion
This study aimed to develop long-acting and sustained release of combination eye drops for the prolonged management of glaucoma. This was accomplished by preparing liposomal vesicles (LV) including 0.5% (w/v) TM, 0.2% (w/v) BT, and bioadhesive and biodegradable polymer (HPMC). Prepared liposomal formulation provided an extended-release of timolol/brimonidine and an extended IOP-lowering effect compared to aqueous solution formulation of timolol/brimonidine eye drops and TM and BT each alone loaded in liposomal formulations. Optimal liposomal formulation possessed ideal pH values that can be easily tolerated by the eye. The pH of tears is 7.4, and can be tolerated due to natural buffering capacity of the eyes. Due to insufficient buffering, pH values outside this range can irritate, and increased blinking, and the sum of these factors leading to reduce the bioavailability of the drug in the eye [36].
Atomic force microscopy images showed that LV has a distinct shape and multilamellar structure. LVs were consistently small and about ranged 214.5 ± 19.43 nm. Although there was an increase in the mean particle size of TM/BT-liposomes after 5 months of storage at 2–8 °C, it still was in the reasonable range. Previous studies showed that even particles smaller than 200 nm are considered acceptable for passive drug targeting [37, 38].
Particle size demonstrated a key role in drug release and permeability through biological membranes in this manner that with lower particle size and higher surface area, higher release and permeability rates were provided. This is due to the drug’s increased contact surface and its better permeability to the target tissue [39].
TM and BT’s release profiles from optimal formulation confirmed that it was possible to prepare sustained-release combination eye drops containing LV. TM/BT-liposomes possessed a sustained drug release rate free of any burst release that may cause a toxic effect. This topic may be due to two factors. The first is that the drugs are trapped in an aqueous core because of the hydrophilic nature of both drugs. The second factor is the structure of the multilamellar membrane of LV which was confirmed by AFM data. These layers around the liposomal vesicles prevent the burst release. Many studies show that coating around the nanoparticles using various materials effectively prevents the burst release of the drug [38, 40]. Many bioadhesive polymers have drug-release retarding properties and are being used in ophthalmic preparations [4142]. HPMC was chosen as a bioadhesive polymer in our study. HPMC is GRAS (generally recognized as a safe) listed ingredient and is used to manufacture various dosage forms available commercially [43]. This polymer is a hydrophilic polymer with many polar functional groups. Upon hydration, the polymeric chains of HPMC are entangled with glycoprotein chains of target tissue resulting in bioadhesion [44]. Different opinions have been proposed for polymers’ adhesion behavior, such as hydrogen bonding, electronic interaction, electronic theory, wettability theory, adsorption theory, and diffusion and interlocking theory [45].
In our study, the EE% of TM and BT in the optimized formulation was 41.36% and 22.36%, respectively, which are relatively desirable rates. In a previous study, the EE% of BT in the optimal liposomal formulation, which was made by the thin layer hydration method, was equal to 42.43% [46]. These results were consistent with our findings. Also, in another study that focused on the production of TM liposomal hydrogels, it was found that the EE% of TM in LV is a function of the pH of the environment. They reported that the maximum EE% of TM was provided at pH 9.2 [47]. Therefore, according to the pH equal to 6.22 in our liposomal formulation, one of the important factors in the low loading of TM in our study can be considered the liposomal formulation’s acidic pH.
As expected, the zeta potential, which represents the surface charge of LV, was positive and equal to + 12.3. The positive surface charge was provided by using a positive agent called stearylamine in the formulation. The purpose of using stearylamine was to induce a positive surface charge in the LV and to increase the electrostatic interaction between the negative charge at the corneal epithelial level and the positive charge at the surface of the LV. In another study, a niosomal formulation was prepared by stearylamine, and they found that the niosomal formulation of acetazolamide reduced the IOP more than the suspension formulation of acetazolamide and niosomal formulation without a positive agent [41]. Besides, another study has shown that liposomes with a positive surface charge have the greatest ability to penetrate the cornea [48]. Although various methods have been used to establish animal models of glaucoma, due to elevated IOP being well recognized as the sole modifiable risk factor for the development of glaucoma in the majority of cases, the establishment of animal models with chronic and acute elevated IOP is favorable for simulating the pathogenesis of glaucoma and evaluation of the effectiveness of drugs [49,50,51,52,53]. One of these methods is the polymer’s injection into the anterior chamber, which depends on the amount, viscosity, and structure of the polymer, leading to the induction of acute or chronic glaucoma [29, 50,51,52,53]. One of our study goals is to investigate the effectiveness of HPMC injections in an animal glaucoma model. In our method, only one injection was performed per sample to reduce the risk of intraocular tissue damage and variation between tests that repeated injections might cause. HPMC increases aqueous humor outflow resistance by blocking the trabecular network; therefore, IOP increases. Our results showed HPMC significantly increased IOP. The elevated IOP levels were maintained for 4.5–5 days. Twenty-four hours after polymer injection, the mean IOP increased by 29.76%. In the control group before injection of HPMC, the mean right eye pressure was 16.73 ± 0.75 mmHg, and at its highest value (79 h after polymer injection) was 29.9 ± 0.48 mmHg. In another study, injection of HPMC + microbead in an anterior chamber significantly increased IOP compared with the microbead receiving and control groups in mice [53]. Researchers in another study found that HPMC in an anterior chamber could increase IOP for 4 days in rabbits [29]. Our evaluation showed the difference between the duration of high IOP in our study compared with the studies mentioned in the injection method (no removal of aqueous humor before injection HPMC vs the removal of aqueous humor before injection HPMC), which was injection volume (0.2 mL vs 0.25 mL) and the number of samples (17 rabbits vs 3 rabbits). Our method kept the high IOP longer. The rate of reduction of IOP in liposomal, aqueous solution, and control groups in the first 24 h after HPMC injection in the anterior chamber was 21.65 + 0.75, 21.97 + 0.22, and 21.8 + 0.66 mmHg, respectively. Also, at the IOP peak, 79 h after HPMC injection, the IOP was 22.07 + 0.63, 26 + 0.18, and 29.9 + 0.48 mmHg, respectively. According to the mentioned results, the formulation of the aqueous solution of timolol/brimonidine, despite the appropriate IOP reduction, did not prevent its increase. Therefore, it can be said that this formulation has not been effective in preventing increased IOP (IOP at peak time was 26 ± 0.18 mmHg vs before the first intervention was 21.97 + 0.22 mmHg, P < 0.001). However, TM/BT-liposomes, TM-liposomes, and BT-liposomes despite being more effective in reducing IOP compared to aqueous solution formulation were able to prevent the process of increasing intraocular pressure (IOP at peak time was 22.07 ± 0.63 mmHg vs before the first intervention was 21.65 + 0.75 mmHg, P > 0.05). In other words, our results showed TM/BT-liposomes have been much more effective than aqueous solution formulation in both controlling and preventing increased IOP. One study found that single-dose intraocular injection of latanoprost nanoliposomes in patients with glaucoma could significantly reduce IOP within the first hour after injection and up to 3 months later. This conjunctival injection was well tolerated in all patients [54]. In our study, in contrast to this study, a non-invasive method was used for drug delivery. From both studies, it can be concluded that using liposomes as a drug carrier, by an invasive or non-invasive method, can significantly improve the effectiveness of ophthalmic drugs with minimal side effects. In another study, the liposomal formulation of brimonidine reduced IOP by 39% and the simple formulation of brimonidine by 59%, but this effect was more stable in the liposomal group with a significant difference [46]. In the study mentioned above, contrary to our study, no positive agent or polymer was used. Also, in our study, a liposomal formulation containing a fixed combination of brimonidine and timolol was used, and the treatment period and number of times of intervention were longer. The sum of the mentioned factors can be considered the factor of more stable and longer control of IOP in our study. According to studies, the greatest reduction in IOP in topical anti-glaucoma drugs dosage from aqueous solution is approximately when the most drug release is done [30, 55,56,57]. Our method was very appropriate for IOP measurement time because pressure measurement was performed when drug release from aqueous solution formulation was at its highest (2 h after taking the drug).
Therefore, in our study, more accurate comparison of IOP reduction between liposomal formulation and the aqueous solution was obtained. The result of the in vitro drug release profile of formulations showed that TM/BT-liposomes provide the prolonged release of drugs compared to aqueous solution formulation (12 h vs 2 h). Our results showed a slow prolonged release of both drugs from TM/BT-LIPOSOMESs, and TM followed first-order kinetics, and BT followed zero-order kinetics. Therefore, TM and BT’s drug release was dependent and independent of the concentration of the drug entrapped, respectively. This would mean that TM/BT-LIPOSOMESs can release TM/BT drug content in a sustained-release system. Therefore, it has expected to keep the drug concentration in the eye constant for a longer period, which is consistent with the results of other studies [46]. In a study aimed at achieving a suitable liposomal formulation of the fixed timolol/latanoprost combination, it was found that the selected liposomal formulation was able to release 72% of timolol and 55% of latanoprost within 6 h. It was also found that the release kinetics for both drugs follow a zero-order model. Animal studies also showed that the liposomal formulation of timolol/latanoprost compared with the marketing formulation of timolol/latanoprost reduces IOP equally after 4 days, and before that, the pressure reduction slope was in favor of the commercial formulation [58]. Our study had a longer release (12 h VS 6 h), lower EE%, and different release kinetics for timolol (first-order vs zero-order) compared to this study. Another important difference between the two studies is that the difference between the amount of IOP between the liposomal formulation and the aqueous solution formulation was clear from the first intervention (from the first intervention onwards P < 0.05 and the fourth intervention onwards P < 0.001). This difference between the two studies could be due to the positive surface charge (+ 12.3mv vs − 17.3mv) and the longer release profile in our study, which leads to better penetration and longer shelf life of the drug in the eye. In two other studies that aimed at achieving the timolol/brimonidine hydrogel formulation to increase the shelf life of the drugs in the eye, the researchers found that the hydrogel formulation released the drugs almost completely after 8 h [59, 60]. In both studies, the release profile showed a burst release; for example, in one of these studies, more than 60% of the timolol and brimonidine were released in the first 1 h [60]. Our study lacked a burst release and a longer release for TM and BT than these two studies. Also, in our study, unlike the two mentioned studies, the release kinetics of drugs were determined. Other research to improve the retention time of TM/BT combination in the eye is confined to the development of an intraocular implant with significant IOP-lowering efficacy over 90 days in vivo [15], in light of what has been said and due to patients’ better compliance and ease of administration of eye drops than marketed ophthalmic formulations [61]. It seems that the use of liposomal eye drops compared to hydrogels is an efficient method for increasing the retention time of combination drugs in the eye, and it can be a bright future in the development of ocular drug delivery methods, especially for the treatment of glaucoma.
Conclusion
The development of TM/BT eye drop liposomal formulation containing stearylamine and HPMC as a positive agent and bio-adhesive polymer, respectively, resulted in successful TM and BT delivery to eye tissue and significant therapeutic effectiveness in terms of IOP reduction. In vitro release study showed that the TM/BT-LIPOSOMESs had no burst release and could provide sustained release for 12 h. Also, the small particle size (nm) of the LV leads to better permeability of the drug to the epithelial tissue of the cornea. It seems that optimized formulation has the potential of being developed into an eye drop formulation of TM/BT leading to better control IOP.
Data availability
The datasets generated during and/or analyzed during the current study are available from the corresponding author on reasonable request.
References
Weinreb RN, Aung T, Medeiros FA. The pathophysiology and treatment of glaucoma. JAMA. 2014;311(18):1901–1911.
Quigley HA, Broman AT. The number of people with glaucoma worldwide in 2010 and 2020. Br J Omlthalmol. 2006;90:262–7.
Tham YC, Li X, Wong TY, Quigley HA, Aung T, Cheng CY. Global prevalence of glaucoma and projections of glaucoma burden through 2040: a systematic review and meta-analysis. Ophthalmology. 2014;121:2081–90.
Gan L, Wang J, Jiang M, Bartlett H, Ouyang D, Eperjesi F, et al. Recent advance in topical ophthalmic drug delivery with lipid-based Nano carriers. Drug Discov Today. 2013;18(5–6):290–7.
Del Amo EM, Urtti A. Current and future ophthalmic drug delivery systems. A shift to the posterior segment. Drug Discov Today. 2008;13:135–143.
Xu J, Xue Y, Hu G, Lin T, Gou J, Yin T, He H, Zhang Y, Tang X. A comprehensive review on contact lens for ophthalmic drug delivery. J Control Release. 2018;281:97–118.
Patel SC, Spaeth GL. Compliance in patients prescribed eyedrops for glaucoma. Ophthalmic Surg Lasers Imaging Retina. 1995;26(3):233–6.
Kobelt-Nguyen G, Gerdtham UG, Alm A. Costs of treating primary open-angle glaucoma and ocular hypertension: a retrospective, observational two-year chart review of newly diagnosed patients in Sweden and the United States. J Glaucoma. 1998;7(2):95–104.
Weinreb RN. Compliance with medical treatment of glaucoma. J Glaucoma. 1992;1(2):134–6.
Lichter PR, et al. Interim clinical outcomes in the Collaborative Initial Glaucoma Treatment Study comparing initial treatment randomized to medications or surgery. Ophthalmology. 2001;108(11):1943–53.
Katz LJ. Modern alchemy: fixed combinations of glaucoma drugs. Am j ophthalmol. 2005;1(140):125–26.
Robin AL, Covert D. Does adjunctive glaucoma therapy affect adherence to the initial primary therapy? Ophthalmology. 2005;112(5):863–8.
Holló G, et al. Long-term outcomes of prostaglandin analog versus timolol maleate in ocular hypertensive or primary open-angle glaucoma patients in Europe. J Ocul Pharmacol Ther. 2011;27(5):493–98.
Fechtner RD, Realini T. Fixed combinations of topical glaucoma medications. Curr Opin Ophthalmol. 2004;15(2):132–5.
Samy KE, Cao Y, Kim J, Konichi da Silva NR, Phone A, Bloomer MM, Bhisitkul RB, Desai TA. Co-delivery of timolol and brimonidine with a polymer thin-film intraocular device. J Ocul Pharmacol Ther. 2019;35:124–131.
Frampton JE. Topical brimonidine 0.2%/timolol 0.5% ophthalmic solution. Drugs Aging. 2006;23(9):753–61.
Goni FJ, Brimonidine/Timolol Fixed Combination Study Group. 12-week study comparing the fixed combination of brimonidine and timolol with concomitant use of the individual components in patients with glaucoma and ocular hypertension. Eur J Ophthalmol. 2005;15(5):581–90.
Arcieri ES, Arcieri RS, Pereira ACA, Andreo EGV, Finotti IGA, SáFilho WF. Comparing the fixed combination brimonidine-timolol versus fixed combination dorzolamide-timolol in patients with elevated intraocular pressure. Curr Med Res Opin. 2007;23:683–9.
Schield's. Text book of glaucoma. 5th ed. Chapter 1 Cellular and molecular biology of aqueous humor dynamics. 15–17.
Katzung BG. Basic & clinical pharmacology. section II; chapter 6. Introduction to autonomic pharmacology. Pharmacology of Eye. 91.
Henderer GD, Rapuano CJ. Goodman & Gilmans. Pharmacol Basis Ther. 1722-23.
Yellepeddi VK, Palakurthi S. Recent advances in topical ocular drug delivery. J Ocul Pharmacol Ther. 2016;32(2):67-82.
Honda M, Asai T, Oku N, Araki Y, Tanaka M, Ebihara N. Liposomes and nanotechnology in drug development: focus on ocular targets. Int J Nanomed. 2013;8:495.
Bangham AD, Standish MM, Watkins JC. Diffusion of univalent ions across the lamellae of swollen phospholipids. J Mol Biol. 1965;13:238–52.
Elshanawane AA, Abdolaziz LM, Mohram MS, Hafez HM. Development and validation of HPLC method for simultaneous estimation of Brimonidine tartrate and timolol maleate in bulk and pharmaceutical dosage forms. J Chromatograph SeparatTechniq. 2014;5:3.
Aggarwal D, Garg A, Kaur IP. Development of a topical niosomal preparation of acetazolamide: preparation and evaluation. J Pharm Pharmacol. 2004;56(12):1509–17.
Maiti S, Paul S, Mondol R, Ray S, Sa B. Nanovesicular formulation of brimonidine tartrate for the management of glaucoma: in vitro and in vivo evaluation. AapsPharmscitech. 2011;12(2):755–63.
Higuchi T. Mechanism of sustained action medication. Theoretical analysis of rate release of solid drugs dispersed in solid matrices. J Pharm Sci. 1963;52:1145-49.
Zhu MD, Cai FY. Development of experimental chronic intraocular hypertension in the rabbit. Aust N Z J Ophthalmol. 1992;20(3):225–34.
Kouchak M, Bahmandar R, Bavarsad N, Farrahi F. Ocular DorzolamideNanoliposomes for Prolonged IOP Reduction: in-vitro and in-vivo Evaluation in Rabbits. Iranian J Pharm Res: IJPR. 2016;15(1):205.
Pokharkar V, Patil V, Mandpe L. Engineering of polymer-surfactant nanoparticles of doxycycline hydrochloride for ocular drug delivery. Drug Deliv. 2015;92(7):955–68.
Morand K, Bartoletti AC, Bochot A, Barratt G, Brandely ML, Chast F. Liposomal amphotericin B eye drops to treat fungal keratitis: physico-chemical and formulation stability. Int J Pharm. 2007;344(1–2):150–3.
Hecht G. Ophthalmic preparations. 4th ed. New York: University of Sciences in Philadelphia. 2001.
Kaur IP, Singh M, Kanwar M. Formulation and evaluation of ophthalmic preparations of acetazolamide. Int J Pharm. 2000;199(2):119–27.
USP29-NF24. Pharmaceutical dosage forms, ophthalmic preparation and uniformity of dosage units chapters. In: The United States Pharmacopeia 29th, The National Formulary 24th. Rockville, MD: United States Pharmacopeial Convention, Inc. 2005.
Scholes PD, Coombes AG, Illum L, Daviz SS, Vert M, Davies MC. The preparation of sub-200 nm poly (lactide-co-glycolide) microspheres for site-specific drug delivery. J Control Release. 1993;25(1–2):145–53.
Ibrahim MM, Abd-Elgawad AE, Soliman OA, Jablonski MM. Natural bioadhesive biodegradable nanoparticle-based topical ophthalmic formulations for management of glaucoma. Translational vision science & technology. 2015;4(3):12–9.
Leroux JC, Allémann E, De Jaeghere F, Doelker E, Gurny R. Biodegradable nanoparticles—from sustained release formulations to improved site specific drug delivery. J Control Release. 1996;39(2–3):339–50.
Garcia-Fuentes M, Torres D, Alonso MJ. New surface-modified lipid nanoparticles as delivery vehicles for salmon calcitonin. Int J Pharm. 2005;296(1–2):122–32.
Kaur IP, Smitha R, Aggarwal D, Kapil M. Acetazolamide: future perspective in topical glaucoma therapeutics. Int J Pharm. 2002;248(1–2):1–4.
Aggarwal D, Garg A, Kaur IP. Development of a topical niosomal preparation of acetazolamide: preparation and evaluation. J Pharm Pharmacol. 2004;56(12):1509-17.
Kashiwagi K, Ito K, Haniuda H, Ohtsubo S, Takeoka S. Development of latanoprost-loaded biodegradable nanosheet as a new drug delivery system for glaucoma. Invest Ophthalmol Vis Sci. 2013;54(8):5629–37.
Nafee NA, Ismail FA, Boraie NA, Mortada LM. Mucoadhesive delivery systems. I. Evaluation of mucoadhesive polymers for buccal tablet formulation. Drug Develop Ind Pharm. 2004;30(9):985–93.
Andrews GP, Laverty TP, Jones DS. Mucoadhesive polymeric platforms for controlled drug delivery. Eur J Pharm Biopharm. 2009;71(3):505–18.
Smart JD. The basics and underlying mechanisms of mucoadhesion. Adv Drug Deliv Rev. 2005;57(11):1556–68.
Prabhu P, Kumar RN, Koland M, Harish NM, Vijayanarayan K, Dhondge G, et al. Preparation and evaluation of nano-vesicles of brimonidine tartrate as an ocular drug delivery system. J Young Pharm. 2010;2(4):356–61.
Hui-Hui Z, Qiu-Hua L, Zhi-Jun Y, Wei-San P, Shu-Fang N. Novel ophthalmic timolol meleate liposomal-hydrogel and its improved local glaucomatous therapeutic effect in vivo. Drug Del. 2011;18(7):502–10.
Li N, Zhuang C, Wang M, Sun X, Nie S, Pan W. Liposome coated with low molecular weight chitosan and its potential use in ocular drug delivery. Int J Pharm. 2009;379(1):131–8.
Ishikawa M, Yoshitomi T, Zorumski CF, Izumi Y. Experimentally induced mammalian models of glaucoma. BioMed Res Int. 2015;2015.
Lu DW, Chen YH, Chang CJ, Chiang CH, Yao HY. Nitric oxide levels in the aqueous humor vary in different ocular hypertension experimental models. Kaohsiung J Med Sci. 2014;30(12):593–8.
Xu Y, Chen Z, Song J. A study of experimental carbomer glaucoma and other experimental glaucoma in rabbits. [Zhonghuayankezazhi] Chin J Ophthalmol. 2002;38(3):172–5.
Kim HG, Park JW, Park SW. Experimental chronic ocular hypertension by anterior chamber injection of 0.3% carbomer solution in the rat. Clin Exp Ophthalmol. 2013;41(4):404–12.
Liu H, Ding C. Establishment of an experimental glaucoma animal model: a comparison of microbead injection with or without hydroxypropyl methylcellulose. Exp Ther Med. 2017;14(3):1953–60.
Wong TT, Novack GD, Natarajan JV, Ho CL, Htoon HM, Venkatraman SS. Nanomedicine for glaucoma: sustained release latanoprost offers a new therapeutic option with substantial benefits over eyedrops. Drug Deliv Transl Res. 2014;4(4):303–9.
Loftsson T, Thorisdottir S, Fridriksdottir H, Stefansson E. Enalaprilat and enalapril maleate eyedrops lower intraocular pressure in rabbits. Acta Ophthalmol. 2010;88(3):337–41.
Mohsen AM, Salama A, Kassem AA. Development of acetazolamide loaded bilosomes for improved ocular delivery: preparation, characterization and in vivo evaluation. J Drug Del Sci Technol. 2020;59:101910
Manchanda S, Sahoo PK. Topical delivery of acetazolamide by encapsulating in mucoadhesive nanoparticles. Asian J Pharm Sci. 2017;12(6):550–7.
Dubey A, Prabhu P, Beladiya K, Nair N, Ghate V. Development and investigation of timolol maleate and latanoprost combination liposomes for the treatment of glaucoma. Int Res J Pharm. 2015;6(4):256–64.
Dubey A, Prabhu P. Formulation and evaluation of stimuli-sensitive hydrogels of timolol maleate and brimonidine tartrate for the treatment of glaucoma. Int J Pharm Investig. 2014;4(3):112.
Taka E, Karavasili C, Bouropoulos N, Moschakis T, Andreadis DD, Zacharis CK, Fatouros DG. Ocular co-delivery of timolol and brimonidine from a self-assembling peptide hydrogel for the treatment of glaucoma: in vitro and ex vivo evaluation. Pharmaceuticals. 2020;13(6):126.
Peng CC, Burke MT, Carbia BE, Plummer C, Chauhan A. Extended drug delivery by contact lenses for glaucoma therapy. J Control Release. 2012;162(1):152–8.
Acknowledgements
This work is issued from Pharm D thesis of Ali Bigdeli and financial support was provided by a grant from Ahvaz Jundishapur University of Medical Sciences, Ahvaz, Iran.
Funding
This work was supported by Ahvaz Jundishapur University of Medical Sciences (Grant number [N-9917].
Author information
Authors and Affiliations
Contributions
All authors contributed to the study conception and design. Material preparation, data collection, and analysis were performed by Behzad Sharif Makhmal Zaddeh, Ali Bigdeli, Mostafa Feghhi, and Emad SoleimaniBiatiani. The first draft of the manuscript was written by Behzad Sharif Makhmal Zadeh and all authors commented on previous versions of the manuscript. All authors read and approved the final manuscript.
Corresponding author
Ethics declarations
Ethics approval
This study was performed in line with the principles of the Declaration of Helsinki. Approval was granted by the Ethics Committee of Ahvaz Jundishapur University of Medical Sciences (2020/02/04./ IR.AJUMS.ABHC.REC.1399.070.).”
Consent for publication
All authors are happy with this publication.
Competing interests
The authors declare no competing interests.
Additional information
Publisher's Note
Springer Nature remains neutral with regard to jurisdictional claims in published maps and institutional affiliations.
Rights and permissions
Springer Nature or its licensor (e.g. a society or other partner) holds exclusive rights to this article under a publishing agreement with the author(s) or other rightsholder(s); author self-archiving of the accepted manuscript version of this article is solely governed by the terms of such publishing agreement and applicable law.
About this article
Cite this article
bigdeli, A., Makhmalzadeh, B.S., Feghhi, M. et al. Cationic liposomes as promising vehicles for timolol/brimonidine combination ocular delivery in glaucoma: formulation development and in vitro/in vivo evaluation. Drug Deliv. and Transl. Res. 13, 1035–1047 (2023). https://doi.org/10.1007/s13346-022-01266-8
Accepted:
Published:
Issue Date:
DOI: https://doi.org/10.1007/s13346-022-01266-8