Abstract
In this paper, we study nonlinear effects in the optical communication fibers such as four wave mixing (FWM) in an integration of dense wavelength division multiplexing (DWDM) with radio over fiber (ROF). We used an optical double side band (ODSB) configuration with direct and external modulation using dual drive Mach–Zehnder modulator. A 16-DWDM-ROF link at different data rates is used for information transmission in single mode fiber. Also, the performance analysis of the proposed system under FWM effect with different parameters such as data rate, channel spacing, input power, fiber length and optical amplifier gain is presented. The results of the proposed system are evaluated based on bit error rate (BER) and maximum quality factor (Q). Results show that the external DSB modulation format has better performance rather than the direct modulation. Generally, for 10 dB input power, 100 GHz channel spacing, 10 Gbps bit rate and 20 km link, the Q is about 20 and BER is less than 10−84 for external ODSB modulation format. Also it has been shown that with increasing data transfer rates, output results such as optical signal-to-noise ratio, BER and Q are deteriorated under influence of FWM-effect.
Similar content being viewed by others
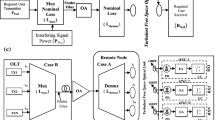
Avoid common mistakes on your manuscript.
Introduction
The demand for high bandwidth with high data rates have increased due to the progress in communication networks. So, the bandwidth extension and elevating the spectral efficiency of the transmission networks are necessary. The wavelength division multiplexing (WDM) systems capable to expand the bandwidth by transmitted several wavelength into single mode fiber (SMF) without other optical fibers [1]. However the WDM systems increase the performance and bandwidth of transmission links with high cost [2]. Therefore advanced optical modulation could increase the spectral efficiency of optical transmission systems [3]. Optical double side band (ODSB) transmission is one of the advanced modulations that can increase the spectral efficiency of optical networks [4]. In an optical transmission system with long distance, four wave mixing (FWM) and chromatic dispersion (CD) are two main issues to design of WDM system. The CD happens in single mode fiber and several methods are used to reduce the CD effect [5]. FWM is one of the nonlinear effects. In FWM three wavelengths mixed and produce fourth wavelength. This effect can cause several problems such as additional noise and loss in the optical power signals [6]. To transmit the signal with ODSB modulation, the modulator configuration in [7] for Radio over Fiber (ROF) transmission is used. It should be noted that the proposed configuration in [7] has better performance such as less CD and high sensitivity in comparison with the optical single sideband (OSSB) signals. To reduce the role of nonlinear effect such as CD and polarization mode dispersion (PMD) in WDM system, two modulations consist of OSSB and optical vestigial sideband (OVSB) was presented in [8]. In [9], direct and external modulation in ROF system was presented. In external modulation, the LiNb Mach–Zehnder (MZM) is used, and in direct modulation format, the vertical-cavity surface-emitting laser (VCSEL) is used. The results demonstrated that external modulation has better performance than direct modulation. Operation of delay interferometer in 5 Gb/s ODSB ROF by RF signals was introduced in [10].
We organized our paper as follows: in the second part, we introduce the modulation techniques and analysis manner. The proposed ROF-DWDM system and simulation results are presented in the third part.
Modulation techniques
The aim of incorporation of fiber optical communication with radio frequency (RF) technology is the ability of integration with other links and reduction of dispersion [11]. Conventional intensity modulation is phase insensitive that allows to RF signals to transmit over an optical carrier. In radio communication systems, the sideband is symmetrically spaced in the frequency domain, below and up of the carrier, that sometimes is known as double sideband amplitude modulation. In direct modulation, electrical information changes the input laser current to produce different optical power. Therefore the direct modulation through generation of 0 and 1 bits, and by turning on and off the laser, is not a suitable manner for high data rates transmission systems [12, 13]. Figure 1a shows the configuration of direct ODSB modulation. In this configuration, the non-return to zero (NRZ) pulse generator multiplexed with Sine generator at 10 GHz. Output of electrical multiplier excited the VCSEL laser, which is known as optical carrier. The output of laser is output of direct ODSB modulation. Figure 1b shows the optical spectra of this configuration at 193.1 THz. In external modulation, laser source current has constant amplitude that enters to a MZM. This modulation is reduced dispersion and increased the data rates. Figure 2 demonstrates the configuration of external ODSB modulation and its optical spectra at 193.1 THz. In this configuration, after multiplexing of the NRZ pulse generator with Sine generator, the output of electrical multiplier excited the LiNb MZM. A continuous waveform (CW) laser used as the input of the LiNb MZM and optical carrier.
A single drive LiNb MZM for external ODSB has only one single driving voltage applied to either arm of MZM. The transmitted optical field Eo (t) at output of a single drive MZM is a function of v(t) and bias DC voltage, Vbias. The expression of Eo (t) is given by [14]:
Figure 3a, shows the structure of LiNb MZM that used in external ODSB modulation. Figure 3b, demonstrates the transmitted optical filed according to (v(t) + Vbias) for different Vπ. As can be seen by increasing the Vπ, the minimum modulation voltage is shifted to higher value.
Simulation results and discussion
Figure 4a shows the schematic diagram of the proposed 16-channel DWDM-ROF link without using any optical amplifier and dispersion compensation in transmission block. In the proposed system a 16 DWDM-ROF link has been modeled for 100 km distance by using direct modulation and external modulation. Our proposed system consists of several components such as: subsystems from #1 to #16, ideal multiplexer and demultiplexer, transmission link and receiver units. In subsystems we used direct and external modulation. Transmission link consists of a SMF with 20 km length, 0.2 dB/km attenuation and 16.75 ps/nm/km dispersion. In the proposed system, we used of a loop control to increase the distance of transmission link. In fiber communication systems chromatic dispersion can be calculated as [15]:
where D is the dispersion, L is the distance, dτg/dλ is the differential of delay time per wavelength to propagate in the L, vg is the group velocity, c is the speed of light in vacuum and β2 is the derivative of group velocity. As shown in Fig. 3b, to recover the signals we used in several devices, these are known the receiver units. At the beginning of the receiver units a Bessel optical filter with 0.3 nm bandwidth and an optical amplifier with 15 dB gain are used to increase the power of the input optical signal. We employed an APD photodetector after optical amplifier to convert the optical signals to electrical signals. Also, for demodulation of the electrical signal, an amplitude demodulator with 1 GHz bandwidth is used.
Figure 4a shows the eye diagram of the proposed system at 1 Gbps with direct ODSB modulation by using VCSEL laser. Results demonstrate that direct ODSB modulation has Max quality factor (Q) equal to 3.53 and Min bit error rate (BER) equal to 1.68 × 10−4. So, this method is not suitable for proposed system. Figure 5b shows the eye diagram of the proposed system with external ODSB modulation. For external ODSB modulation with CW laser array, the system has 19.52 Max Q and 3.89 × 10−85 Min BER which shows good performance of the system.
According to the results, the direct modulation is not suitable for our proposed system. Therefore in this section we try to optimize the nonlinear effects such as FWM and chromatic dispersion in external ODSB modulation. FWM is considered as a scattering process and the power generated due to the interaction of signals is given by [16]:
where K is the nonlinear interaction constant, α is the attenuation constant, L is the fiber length, η is the quantum efficiency and D is the degeneration factor. It is obvious that increasing of the fiber length or input power of the system, leads to enhancement of the FWM effect. It should be noted that the nonlinear effects influence the maximum quality factor and the minimum BER. Figure 6 shows the Pfwm versus input power for different fiber link lengths. The parameters which we have changed to improve the nonlinear effects, consist of bit rate, channel spacing, input power and length of the fiber. Also, for performance evaluation of the proposed system, we analyzed the output optical signal-to-noise ratio (OSNR), a parameter that assesses the quality factor of the received signals. It is defined as the ratio of the average optical signal power to the optical noise power, and is given by:
where NF is the noise figure, G is the amplifier gain, hf is the photon energy and Δf is the optical bandwidth [16].
Input power effects
In optical transmission system, reduction of dispersion is obtained by increasing the input signal power. However, enhancement of the signal power increases the nonlinear effects of the system and leads to signal distortion [14]. Figure 6 shows the optical spectra and eye diagram of the output signals from the ideal multiplexer for − 10, 0, 10 dB optical power. Figure 7 shows the OSNR for − 10, 0, 10 dB input power with 100 GHz channel spacing and 193.1 THz center frequency. From the Fig. 7, it is obvious that by decreasing the input power, the nonlinear effects are decreased. Despite of the reduction of the nonlinear effects in − 10 dB input power, the eye diagram does not give good performance. Therefore, the system with 0 dB has less FWM and good eye diagram. Output OSNR versus channel number at different amount of input power (IP) is shown in Fig. 8.
Channel spacing effects
The channel spacing describes the frequency spacing between two different channels [17]. Figure 9, shows the optical spectra versus wavelength at the point after the demultiplexer and eye diagram of the proposed system at 1 Gbps with several channel spacings, respectively. The channel spacings consist of 100, 110 and 120 GHz. By increasing the channel spacing, BER and Max quality factor give better performance for proposed system. OSNR Output versus the number of channels for different channel spacings (CS) is shown in Fig. 10. It is obvious that by increasing the channel spacing, the OSNR increases. Therefore, by increasing the value of channel spacing from 100 to 120 GHz, the nonlinear effect decreases.
Bit rate effects
To improve the capacity of a communication systems, one method is enhancement of the data rate value. But data rate enhancement leads to undesirable effects on the performance of the system [18]. So increasing the bit rate in the proposed system is not suitable. Figure 11, shows the optical spectra and eye diagram of the system at the point after the demultiplexer at different bit rates, 1, 2 and 3 Gbps, respectively. From this figure, it is obvious that by increasing the bit rate, Max quality factor and BER were deteriorated. So that, at bit rates higher than 2 Gbps, the received signals are destroyed. Figure 12 demonstrate the OSNRs of the proposed system at different bit rates. As can be seen by increasing bit rates, the value of OSNR decreases. Therefore, the optimization value of the bit rate is about 1 Gbps.
Fiber length effects
In this section, the nonlinear effects such as chromatic dispersion which are dependent on the fiber length is discussed. Figure 10 shows the optical spectra at the point after the demultiplexer and eye diagram of the proposed system at different lengths of fiber, respectively. From this figure, it is obvious that by increasing the fiber lengths, Max quality factor, BER and optical power from – 20 to − 50 dB that express increasing the FWM, are decreased. Figure 13 shows the optical power and eye diagram of the proposed system for different fiber lengths from 20 to 30 km. Also, In Fig. 14 the OSNR output of the system for mentioned fiber lengths are depicted. It is clear that by increasing the length of fiber from 20 km to 35 m the OSNR of the proposed system is decreased.
Conclusion
In this paper, a DWDM-ROF optical transmission system is designed and simulated in direct and external ODSB modulation. Based on the results obtained it has been founder the direct modulation unlike the external modulation is not appropriate for the proposed system. For external modulation format, we tried to optimize the nonlinear effects by changing the parameters of the system such as input power, bit rate, channel spacing and fiber length. Increasing the magnitude of the input power, enhances the Max quality factor and BER, but the nonlinear effects is increased too. Higher bit rate and/or longer fiber length, are caused to deterioration of the performance of the system but more channel spacings of the transmitter, lead to better performance of the system parameters such as OSNR, Max quality factor and BER. The proposed system with the mentioned characteristics is appropriate for modern wireless communication.
References
M. Marciniak, 100 Gb Ethernet over fibre networks-reality and challenges, in ICTON Mediterranean Winter Conference, ICTON-MW (2007), p. 1–6
J.M. Kahn, K.P. Ho, Spectral efficiency limits and modulation/detection techniques for DWDM systems. IEEE J. Sel. Top. Quantum Electron. 10, 259–272 (2004)
P.J. Winzer, R.J. Essiambre, Advanced optical modulation. Proc. IEEE 94, 952–985 (2006)
C. Lim, M. Attygalle, A. Nirmalathas, D. Novak, Analysis of optical carrier-to-sideband ratio for improving transmission performance in fiber-radio links. IEEE Trans. Microw. Theory Tech. 10, 2181–2187 (2006)
G.P. Agrawal, Fiber-Optic Communication Systems (Wiley, Hoboken, 2010)
G. Deshmukh, S. Jagtap, Four wave mixing in DWDM optical system. Int. J. Comput. Eng. Res. (IJCER) 03, 7–11 (2013)
T.E. Dimmick, G. Rossi, D.J. Blumenthal, Optical dispersion monitoring technique using double sideband subcarriers. IEEE Photonics Technol. Lett. 10, 900–902 (2000)
Y. Kim, S. Kim, I. Lee, J. Jeong, Optimization of transmission performance of 10-Gb/s optical vestigial sideband signals using electrical dispersion compensation by numerical simulation. IEEE J. Sel. Top. Quantum Electron. 12, 371–375 (2004)
S.A. Khwandah, J.P. Cosmas, I.A. Glover, P.I. Lazaridis, N.R. Prasad, Z.Z.D. Zaharis, Direct and external intensity modulation in OFDM RoF links. IEEE Photonics J. 7, 7902710 (2015)
H. Singh, A. Sheetal, A. Kumar, Impact of interferometer delay time on the performance of ODSB-SC RoF system with wavelength interleaving. Opt. Int. J. Light Electron Opt. 125, 2057–2061 (2013)
R. Montgomery, R. DeSalvo, A novel technique for double sideband suppressed carrier modulation of optical fields. IEEE Photonics Technol. Lett. 7, 434–436 (1995)
T. Heil, J. Mulet, I. Fischer, C.R. Mirasso, M. Peil, P. Colet, W. Elsasser, ON/OFF phase shift keying for chaos-encrypted communication using external-cavity semiconductor lasers. IEEE J. Quantum Electron. 38, 1162–1170 (2002)
A. Bahrami, T. Kanesan, W.P. Ng, Z. Ghassemlooy, A.A. Aziz, S. Rajabhandari, Performance evaluation of radio-over-fibre (RoF) system using Mach–Zehnder modulator (MZM) and on-off keying (OOK) modulation schemes, in The 11th Annual Post Graduate Symposium on the Convergence of Telecommunications, Networking and Broadcasting (2010)
L.N. Binh, Optical Fiber Communication Systems with Matlab and Simulink Models (CRC Press, Boca Raton, 2014)
G. Keise, Optical Fiber Communications (Wiley Online Library, New York, 2003)
S.E. Miller, A.G. Chynoweth, Optical Fiber Telecommunications (Academic Press, New York, 1979)
T. Jiang, Y. Wu, An overview: peak-to-average power ratio reduction techniques for OFDM signals. IEEE Trans. Broadcast. 54, 257–268 (2008)
H. Yang, W.K.S. Tang, G. Chen, G. Jiang, System design and performance analysis of orthogonal multi-level differential chaos shift keying modulation scheme. IEEE Trans. Circuits Syst. I 63, 146–156 (2016)
Author information
Authors and Affiliations
Corresponding author
Rights and permissions
About this article
Cite this article
Alipoor, A., Mir, A. & Sheikhi, A. Study of DWDM-ROF link nonlinear effects using direct and external ODSB modulation formats. J Opt 47, 263–271 (2018). https://doi.org/10.1007/s12596-018-0466-x
Received:
Accepted:
Published:
Issue Date:
DOI: https://doi.org/10.1007/s12596-018-0466-x