Abstract
Purpose
Cervical cancer is one of the most prevalent malignancies in women worldwide. Therefore, the investigation about the molecular pathogenesis and related therapy targets of cervical cancer is an emergency. The objective of the present study is to investigate the effects of valproic acid (VPA), a histone deacetylase inhibitor, on the angiogenesis of cervical cancer.
Methods
The effects and mechanisms of VPA on in vitro angiogenesis and vascular endothelial growth factor (VEGF) expression of human cervical cancer HeLa and SiHa cells were investigated.
Results
Our present study reveals that 1 mM VPA can significantly inhibit the in vitro angiogenic potential and VEGF expression of human cervical cancer HeLa and SiHa cells. Further, the transcription and protein levels of hypoxia inducible factor-1α (HIF-1α), and not HIF-1β, are significantly inhibited in VPA-treated cervical cancer cells. Over expression of HIF-1α can obviously reverse VPA-induced VEGF down regulation. VPA-treatment decreases the activation of Akt and ERK1/2 in both HeLa and SiHa cells in a time-dependent manner. The inhibitor of Akt (LY 294002) or ERK1/2 (PD98059) can inhibit VEGF alone and cooperatively reinforce the suppression effects of VPA on HIF-1α and VEGF expression.
Conclusion
Collectively, our data reveal that the inhibition of PI3K/Akt and ERK1/2 signals are involved in VPA-induced HIF-1α and VEGF suppression of cervical cancer cells.
Similar content being viewed by others
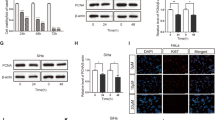
Avoid common mistakes on your manuscript.
Introduction
Cervical cancer is one of the most prevalent malignancies in women worldwide, especially in developing countries. On a global scale, it is estimated that there are 530,000 new cases and 275,000 resultant deaths each year [1]. Human papilloma virus (HPV) infection is the leading cause of cervical cancer. Genetic and epigenetic alternations are also important for the tumorigenesis and development of cervical cancer besides HPV. Surgeries accompanied with radiotherapy and chemotherapy have been considered as the major treatment method for cervical cancer [2]. Generally, chemotherapy is used to treat patients with metastasis or recurrence at times. Although some causes of cervical cancer have been illustrated, the precise mechanisms for the progression, metastasis and angiogenesis are still largely unknown. There is an urgent need to investigate the molecular pathogenesis and related therapy targets of cervical cancer.
Epigenetic modifications have been suggested to act as an important role in cancer cell biology [3, 4]. Histone deacetylases (HDACs), which remove acetyl groups from the N-acetyllysines on histone and non-histone proteins, have garnered great interest as anti-cancer therapeutic targets [5]. Valproic acid (VPA, 2-propylpentanoic acid), which has long been used clinically for treatment of epilepsy and bipolar disorder without significant toxicity [6], is suggested to be a HDAC inhibitor (HDACI) in recent years [7]. Numerous studies show its anti-cancer effects in various cancer models including lung, renal, bladder, and cervical cancer via inhibition of cell proliferation and inducing cell apoptosis [8–11]. However, other roles and mechanisms of VPA on the progression of cervical cancer remain to be further illustrated.
Angiogenesis, which describes the formation of new blood vessels from the existing vasculature, is critical for tumor growth and metastasis [12]. Therefore the anti-angiogenic agents have gathered special attention for the development of anti-cancer drugs. Within tumors, circulating cells such as bone marrow derived endothelial progenitor cells, macrophages and fibroblasts with malignant cells are able to secrete pro-angiogenic factors including vascular endothelial growth factor (VEGF) and then induce tumor blood vessel formation [13]. Recent studies indicated that HDACIs such as FK228 can inhibit the expression of angiogenic factors, including VEGF and basic fibroblast growth factor (bFGF), and therefore suppress the angiogenesis of cancer cells [12, 14, 15]. Although few studies also suggested that VPA can modulate the progression of angiogenesis in cancer cells [16–18], there is insufficient amount of data on its effects in cervical cancer cells. The present study investigates the roles and related mechanisms of VPA on the angiogenic potential of cervical cancer cells and suggests that VPA can down-regulate VEGF via HIF-1α signals.
Materials and methods
Cell culture and transfection
Human cervical cancer cell lines HeLa and SiHa were obtained from the American Type Culture Collection (ATCC) and cultured in DMEM containing 10 % FBS and antibiotics. All cells were grown at 37 °C in a humidified 5 % CO2 incubator. VPA was dissolved in DMSO with the final concentration of DMSO in the medium less than 0.5 %. For transfection, HeLa or SiHa cells were seeded into plates and transfected with siRNA negative control (siRNA-NC: 5′-GGC TAC GTC CAG GAG CGC A-3′), si-HIF-1α (sequence 5′-GAG GAA ACU UCU GGA UGC UGG UGA Utt-3′), pcDNA3.1 (vector control), or pcDNA3.1/HIF-1α by use of Lipofectamine 2000 reagent (Invitrogen) according to the manufacturer’s instructions.
Cell proliferation assays
For the cell proliferation assays, HeLa or SiHa cells (7 × 103 cells/well) were separately seeded in 96-well plates and then treated with various concentrations of VPA for the indicated times. Then cell viability was assessed using the MTT [3-(4,5-dimethylthiazol-2-yl)-2,5-diphenyltetrazolium bromide] assay as previously described [19].
Tube formation assays
Human umbilical vein endothelial cells (HUVECs) were obtained from Pricells (Wuhan, Hubei, China), suspended in conditioned media, and seeded on growth factor-reduced Matrigel (BD Biosciences, Franklin Lakes, NJ, USA) in a 12-well plate. After incubation with different condition medium, the number of mature, well-connected capillary tubes were manually counted under an inverted Nikon Eclipse Ti microscope (Nikon USA, Melville, NY, USA) and compared among different groups.
Quantitative real-time PCR analysis (qRT-PCR)
Total RNA samples were extracted from cells using Trizol reagent (Invitrogen, Carlsbad, CA, USA). The RNA was converted into cDNAs using the RevertAid First Strand cDNA Synthesis Kit (Fermentas, K1621). The cycle number when the fluorescence first reached a preset cycle threshold (Ct) was used to quantify the initial concentration of individual templates for expression of mRNA of genes of interest. Transcripts of the housekeeping gene GAPDH in the same incubations were used for internal normalization. Primer sequences were as follows: VEGF, 5′-TCG AGT ACA TCT TCA AGC CAT CCT G-3′ and 5′-TCC TAT GTG CTG GCC TTG GTG AG-3′; GAPDH, 5′-TGA ACG GGA AGC TCA CTG G-3′ and 5′-TCC ACC ACC CTG TTG CTG T-3′. After normalization to GAPDH gene, expression levels for each target gene were calculated using the comparative CT method. The Dct values were calculated according to the formula Δct = ct (gene of interest) − ct (GAPDH) in correlation analysis, and the 2−ΔΔct was calculated according to the formula ΔΔct = Δct (control group) − Δct (experimental group) for determination of relative.
Quantification of VEGF protein
VEGF protein was measured using the Quantikine human VEGF ELISA kit from R&D Systems (Minneapolis, MN, USA) according to previous study [20]. Briefly, cells were seeded in 12-well plates and cultured to 90–100 % confluence. Cells were switched to fresh medium in the presence or absence of 1 mM VPA. After treatment, the supernatants were collected and cell numbers of each well were counted. VEGF in the supernatant (100 μL) was determined and normalized to the remaining cell numbers. A serial dilution of human recombinant VEGF was included in each assay to obtain a standard curve.
Western blot analysis
Cells were grown in 6-cm dishes, exposed to VPA for the indicated times, and then lysed in 500 μL of SDS buffer (0.125 M Tris–HCl, 10 % 2-mercaptoethanol, 4 % SDS, and 10 % sucrose) and sonicated on ice for 30 s. The protein concentrations were determined with the bicinchoninic acid method. Equal quantities of proteins were loaded and separated by electrophoresis in SDS-PAGE and then transferred onto polyvinylidene fluoride membranes. The membranes were incubated with primary antibodies at 4 °C overnight. Blots were stained with horseradish peroxidase-linked goat anti-rabbit Ig secondary antibodies and developed with a chemiluminescence detection system (Fujifilm).
Statistical analyses
Statistical analyses were performed using SPSS 17.0 (SPSS, Inc., Chicago, IL, USA). All the data presented are based on an average of at least three independent experiments. The values are presented as the mean ± standard deviation. Student’s t test’s were used to compare the differences between groups. P < 0.05 was used to indicate a statistically significant difference.
Results
The effects of VPA on the in vitro proliferation of cervical cancer cells
To explore whether VPA might be a potential therapeutic agent against cervical cancer, we investigated its effects of growth inhibition in human cervical cancer HeLa and SiHa cells. Our results indicated that VPA can inhibit the proliferation of HeLa (Fig. 1a) and SiHa (Fig. 1b) cells via both time and concentration dependent manners. The IC50 of VPA in HeLa and SiHa cells at 24 h was 5.6 and 6.3 mM, respectively, and at 48 h was 2.8 and 3.4 mM, respectively. Therefore, 1 mM VPA was chosen for further studies due to having no significant effect on the proliferation of cervical cancer cells and on the basis of other previous studies [21, 22].
VPA inhibits the angiogenic potential of cervical cancer cells
Recent studies indicated that HDACIs including VPA can modulate the progression of angiogenesis in cancer cells [16–18]. Then we tested the effects of VPA on the angiogenic potential of cervical cancer cells by use of in vitro tube formation assay. Our results showed that tube formation of HUVEC cells was significantly (p < 0.01) inhibited by medium prepared from 1 mM VPA-treated HeLa and SiHa cells (Fig. 2a, b). These results suggested that VPA can inhibit the angiogenic potential of cervical cancer cells.
VPA inhibits the angiogenic potential of cervical cancer cells. a HeLa or SiHa cells were treated with 1 mM VPA for 24 h, then the medium of HeLa or SiHa cells was used for conditioned media to test the tube formation for HUVEC cells. b Quantitative analysis of tube formation assays. Data represented the average of three independent experiments. *p < 0.05 compared with control
VPA inhibits VEGF expression of cervical cancer cells
Then we determined the effects of VPA on VEGF mRNA levels in cervical cancer cells. As shown in Fig. 3a, VPA can inhibit the expression of VEGF mRNA in a time-dependent manner in both HeLa and SiHa cells. Then the protein expression of VEGF was measured in VPA treated HeLa or SiHa cells by use of ELISA. The results confirmed that VPA can significantly decrease the production of VEGF protein in both cell lines (Fig. 3b). Collectively, our results suggested that VPA can inhibit the expression of VEGF in cervical cancer cells.
VPA inhibits the expression of VEGF in cervical cancer cells. a HeLa or SiHa cells were treated with 1 mM VPA for the indicated times, and then the mRNA levels of VEGF were measured by qRT-PCR; b HeLa or SiHa cells were treated with 1 mM VPA for the indicated times, the protein expression of VEGF was measured by ELISA. Data represent the average of three independent experiments. *p < 0.05 compared with control
HIF-1α mediates the suppression effects of VPA on VEGF expression of cervical cancer cells
Hypoxia inducible factor 1 (HIF-1) can activate the expression of the VEGF gene by binding to the hypoxia response element (HRE) in the VEGF promoter [23]. HIF-1 is composed of HIF-1α and HIF-1β subunits [24]. To determine whether HIF-1 is involved in VPA-induced VEGF suppression, we examined the effects of VPA on HIF-1α and HIF-1β protein levels. The results showed that VPA can obviously decrease the expression of HIF-1α in both HeLa and SiHa cells, however, VPA had no significant effect on HIF-1β protein levels (Fig. 4a). This was further confirmed by the results that VPA can significantly inhibit the mRNA levels of HIF-1α, but not HIF-1β, in both HeLa and SiHa cells (Fig. 4b).
HIF-1α mediates the suppression effects of VPA on VEGF expression of cervical cancer cells. a HeLa or SiHa cells were treated with 1 mM VPA for the indicated times, and then protein levels of HIF-1α or HIF-1β were measured by use of Western blot analysis; b HeLa or SiHa cells were treated with 1 mM VPA for 12 h, the mRNA of HIF-1α or HIF-1β was measured by qRT-PCR; c HeLa or SiHa cells were transfected with pcDNA3.1 (vector) or pcDNA/HIF-1α for 24 h, the expression of HIF-1αwas measured by use of Western blot analysis; d HeLa or SiHa cells were transfected with pcDNA3.1 (vector) or pcDNA/HIF-1α for 24 h and then treated with 1 mM VPA for another 24 h, the protein expression of VEGF was measured by ELISA. Data represented the average of three independent experiments. **p < 0.01 compared with control
To verify the roles of HIF-1α in VPA-induced VEGF suppression, HeLa or SiHa cells were transfected with pcDNA3.1 (vector) or pcDNA/HIF-1α for 24 h, then further treated with VPA for 24 h. The over expression of HIF-1α was confirmed by the results of Western blot analysis (Fig. 4c). Our results showed that over expression of HIF-1α significantly attenuated VPA-induced down-regulation of VEGF in both HeLa and SiHa cells (Fig. 4d). Collectively, our results revealed that HIF-1α mediates the suppression effects of VPA on VEGF expression of cervical cancer cells.
VPA suppresses the activities of PI3K/Akt and ERK1/2 in cervical cancer cells
Several upstream signals including PI3K/Akt and ERK1/2 can regulate the expression of HIF-1α and VEGF [25, 26]. To identify which signal is responsible for the inhibition effects of VPA on expression of HIF-1α, we determined the effects of VPA on activation of Akt and ERK1/2 by Western blot analysis. The results showed that 1 mM VPA can obviously inhibit Akt phosphorylation in both HeLa (Fig. 5a) and SiHa (Fig. 5b) cells. Further, incubation of cells with VPA also resulted in significant decrease of phospho-ERK1/2 levels. These results suggested that both PI3K/Akt and ERK1/2 might be involved in the effects of VPA on HIF-1α and VEGF down-regulation.
Inhibition of PI3K/Akt and ERK1/2 cooperatively reinforces VPA-induced HIF-1α and VEGF suppression
To test whether PI3K/Akt and ERK1/2 signals are involved in VPA-induced HIF-1α and VEGF suppression, HeLa and SiHa cells were treated with PI3K/Akt inhibitor (LY 294002, LY) and ERK1/2 inhibitor (PD98059, PD) alone or combination with VPA. Our results showed that both LY 294002 and PD98059 can obviously inhibit the expression of HIF-1α, further, the inhibition effects of VPA on HIF-1α expression were significantly reinforced with the cooperation of LY 294002 or PD98059 in both HeLa and SiHa cells (Fig. 6a). Similar results were also observed for the effects of VPA on VEGF protein (Fig. 6b, c) and mRNA (Fig. 6d, e) expression. These data suggested that the inhibition of PI3K/Akt and ERK1/2 signals are involved in VPA-induced HIF-1α and VEGF suppression of cervical cancer cells.
Inhibition of PI3K/Akt and ERK1/2 cooperatively reinforces VPA-induced HIF-1α and VEGF suppression. a HeLa or SiHa cells were treated with VPA (1 mM), LY294002 (10 μM), or PD98059 (10 μM) alone or together for 24 h, and then protein level of HIF-1α was measured by use of Western blot analysis; HeLa (b) or SiHa (c) cells were treated with VPA (1 mM), LY294002 (10 μM), or PD98059 (10 μM) alone or together for 24 h, the protein expression of VEGF was measured by ELISA; HeLa (d) or SiHa (e) cells were treated with VPA (1 mM), LY294002 (10 μM), or PD98059 (10 μM) alone or together for 12 h, the mRNA expression of VEGF was measured by qRT-PCR. Data represented the average of three independent experiments
Discussion
Tumors cannot grow larger than 1–2 mm in diameter without vessel formation, therefore anti-angiogenesis is considered as a new attractive strategy to overcome cancer. In the present study, VPA, with a concentration which has no significant effect on cell proliferation, can inhibit the VEGF expression and in vitro angiogenic potential of cervical cancer cells. VPA-treatment significantly suppresses the expression of HIF-1α, while not HIF-1β. Over expression of HIF-1α can reverse the suppression effects of VPA on VEGF expression. Further, the inhibition of PI3K/Akt and ERK1/2 also participates in VPA-induced HIF-1α/VEGF down-regulation. Collectively, our present study shows that VPA can inhibit the angiogenic potential of cervical cancer cells via HIF-1α/VEGF signals. The identified anti-angiogenic activities of VPA in the present study suggested that it can become a valuable new addition in the attempt to develop alternative therapeutic approaches for the treatment of cervical cancer.
Inhibition of VEGF production is a promising therapeutic approach for cancer. HDACI is a new class of anti-cancer therapeutics which show great promise at inhibiting VEGF and angiogenesis [12]. Due to the low cost and favorable safety profile, VPA has been considered as a candidate drug for various cancer cell types [27–29]. Several recent studies also indicated that VPA can inhibit proliferation, migration, and tube formation of human umbilical vein endothelial cells both in vitro and in vivo [30, 31]. In our present study, VPA can significantly inhibit the in vitro angiogenic potential and VEGF expression of both HeLa and SiHa cells. This is consistent with previous study that VPA can impair the secretion of VEGF in colon and prostate cancer cells [32, 33]. Several studies also indicated that VPA can regulate the expression of VEGFR, FGF and other angiogenesis related factors in colon and myeloma cancer cells [18, 32, 34]. These effects of VPA on cervical cancer need further studies.
Various mechanisms have been reported to be involved in HDACI-induced anti-angiogenesis of cancer cells [12, 35]. In the present study, our results show that VPA can inhibit the HIF-1α/VEGF signals and then suppress the angiogenic potential. Increasing evidences suggest that HIF-1 plays a critical role in tumor progression and acts as a key regulator of VEGF expression. It has been documented that HDACIs, including VPA, were able to repress HIF-1 function in cancer cells [9, 36]. HIF-1α activates its target genes such as erythropoietin (EPO), VEGF, and phosphoglycerate kinase 1 (PGK1) through dimerization with HIF-1β. In the present study, our data showed that VPA-treatment can significantly suppress the expression of HIF-1α, while not HIF-1β, in cervical cancer cells. This might be due to the fact that VPA can significantly inhibit the transcription of HIF-1α. Further, overexpression of HIF-1α abolishes VPA-induced VEGF down-regulation. It confirms the essential role of HIF-1α in the suppression effects of VPA on angiogenic potential of cervical cancer cells.
The mechanisms responsible for HDACIs, including VPA, induced HIF-1α down regulation are not fully illustrated. Some literature suggests that HDACIs decrease the stability of HIF-1α through enhancing the acetylation of HIF-1α and/or other proteins involved in modulating the degradation of HIF-1α, thus accelerating the degradation of HIF-1α [37]. Enhanced signaling through the PI3K/Akt and MAPK pathways are a significant contributor to activate HIF-1α/VEGF signals [26]. However, the pathways responsible for VEGF production may be dependent on the cell lines. It has been reported that activation of the Akt pathway, but not the ERK pathway, induced VEGF production in the PC3 human prostate cancer cell line [38]. Inversely, activation of the ERK pathway, but not the Akt pathway, promoted VEGF production in human HaCaT keratinocytes [39]. Our present study reveals that both the activation PI3K/Akt and ERK1/2 are inhibited by VPA-treatment; furthermore, the inhibitors of PI3K/Akt and ERK1/2 can cooperatively reinforce the inhibition effects of VPA on HIF-1α and VEGF expression. It suggests that both PI3K/Akt and ERK1/2 are involved in the suppression effects of VPA on angiogenic potential of cervical cancer cells.
In conclusion, VPA inhibits angiogenic potential of cervical cancer cells via suppression of HIF-1α/VEGF signals. The suppression of PI3K/Akt and ERK1/2 signals are both involved in this process. Though the precise mechanism remains undetermined, these findings will help to better understand the roles and mechanisms of VPA as a potential anti-cancer drug for cervical cancer patients.
References
Arbyn M, Castellsague X, de Sanjose S, Bruni L, Saraiya M, Bray F, et al. Worldwide burden of cervical cancer in 2008. Ann Oncol. 2011;22:2675–86.
Maestri D, Reis RJ, Bacha OM, Muller B, Corleta OC. A comparison of radical vaginal hysterectomy combined with extraperitoneal or laparoscopic pelvic lymphadenectomy in the treatment of cervical cancer. Int J Gynecol Cancer. 2012;22:1238–43.
Jones PA, Baylin SB. The epigenomics of cancer. Cell. 2007;128:683–92.
Baylin SB, Jones PA. A decade of exploring the cancer epigenome—biological and translational implications. Nat Rev Cancer. 2011;11:726–34.
Zhang J, Zhong Q. Histone deacetylase inhibitors and cell death. Cell Mol Life Sci. 2014;71:3885–901.
van Breemen MS, Rijsman RM, Taphoorn MJ, Walchenbach R, Zwinkels H, Vecht CJ. Efficacy of anti-epileptic drugs in patients with gliomas and seizures. J Neurol. 2009;256:1519–26.
Gottlicher M, Minucci S, Zhu P, Kramer OH, Schimpf A, Giavara S, et al. Valproic acid defines a novel class of HDAC inhibitors inducing differentiation of transformed cells. EMBO J. 2001;20:6969–78.
Liu X, Chen L, Sun F, Zhang G. Enhanced suppression of proliferation and migration in highly-metastatic lung cancer cells by combination of valproic acid and coumarin-3-carboxylic acid and its molecular mechanisms of action. Cytotechnology. 2013;65:597–608.
Yang FQ, Liu M, Yang FP, Che J, Li W, Zhai W, et al. VPA inhibits renal cancer cell migration by targeting HDAC2 and down-regulating HIF-1alpha. Mol Biol Rep. 2014;41:1511–8.
Wang D, Jing Y, Ouyang S, Liu B, Zhu T, Niu H, et al. Inhibitory effect of valproic acid on bladder cancer in combination with chemotherapeutic agents in vitro and in vivo. Oncol Lett. 2013;6:1492–8.
Moreaux J, Reme T, Leonard W, Veyrune JL, Requirand G, Goldschmidt H, et al. Gene expression-based prediction of myeloma cell sensitivity to histone deacetylase inhibitors. Br J Cancer. 2013;109:676–85.
Ellis L, Hammers H, Pili R. Targeting tumor angiogenesis with histone deacetylase inhibitors. Cancer Lett. 2009;280:145–53.
Lin EY, Pollard JW. Tumor-associated macrophages press the angiogenic switch in breast cancer. Cancer Res. 2007;67:5064–6.
Deroanne CF, Bonjean K, Servotte S, Devy L, Colige A, Clausse N, et al. Histone deacetylases inhibitors as anti-angiogenic agents altering vascular endothelial growth factor signaling. Oncogene. 2002;21:427–36.
Kwon HJ, Kim MS, Kim MJ, Nakajima H, Kim KW. Histone deacetylase inhibitor FK228 inhibits tumor angiogenesis. Int J Cancer. 2002;97:290–6.
Shan Z, Feng-Nian R, Jie G, Ting Z. Effects of valproic acid on proliferation, apoptosis, angiogenesis and metastasis of ovarian cancer in vitro and in vivo. Asian Pac J Cancer Prev. 2012;13:3977–82.
Osuka S, Takano S, Yamamoto T, Ishikawa E, Matsumura A. Histone deacetylase inhibitor, valproic acid inhibits glioma angiogenesis in vitro and in vivo in the brain. Neuro-oncol. 2009;11:962.
Zhang ZH, Hao CL, Liu P, Tian X, Wang LH, Zhao L, et al. Valproic acid inhibits tumor angiogenesis in mice transplanted with Kasumi1 leukemia cells. Mol Med Rep. 2013;9:443–9.
Ge LC, Chen ZJ, Liu HY, Zhang KS, Liu H, Huang HB, et al. Involvement of activating ERK1/2 through G protein coupled receptor 30 and estrogen receptor alpha/beta in low doses of bisphenol A promoting growth of Sertoli TM4 cells. Toxicol Lett. 2014;226:81–9.
Fang J, Xia C, Cao Z, Zheng JZ, Reed E, Jiang BH. Apigenin inhibits VEGF and HIF-1 expression via PI3K/AKT/p70S6K1 and HDM2/p53 pathways. FASEB J. 2005;19:342–53.
Mawatari T, Ninomiya I, Inokuchi M, Harada S, Hayashi H, Oyama K, et al. Valproic acid inhibits proliferation of HER2-expressing breast cancer cells by inducing cell cycle arrest and apoptosis through Hsp70 acetylation. Int J Oncol. 2015;47:2073–81.
Sami S, Hoti N, Xu HM, Shen ZL, Huang XF. Valproic acid inhibits the growth of cervical cancer both in vitro and in vivo. J Biochem (Tokyo). 2008;144:357–62.
Forsythe JA, Jiang BH, Iyer NV, Agani F, Leung SW, Koos RD, et al. Activation of vascular endothelial growth factor gene transcription by hypoxia-inducible factor 1. Mol Cell Biol. 1996;16:4604–13.
Jiang BH, Rue E, Wang GL, Roe R, Semenza GL. Dimerization, DNA binding, and transactivation properties of hypoxia-inducible factor 1. J Biol Chem. 1996;271:17771–8.
Blancher C, Moore JW, Robertson N, Harris AL. Effects of ras and von Hippel-Lindau (VHL) gene mutations on hypoxia-inducible factor (HIF)-1alpha, HIF-2alpha, and vascular endothelial growth factor expression and their regulation by the phosphatidylinositol 3′-kinase/Akt signaling pathway. Cancer Res. 2001;61:7349–55.
Shen K, Ji L, Gong C, Ma Y, Yang L, Fan Y, et al. Notoginsenoside Ft1 promotes angiogenesis via HIF-1alpha mediated VEGF secretion and the regulation of PI3K/AKT and Raf/MEK/ERK signaling pathways. Biochem Pharmacol. 2012;84:784–92.
Das CM, Aguilera D, Vasquez H, Prasad P, Zhang M, Wolff JE, et al. Valproic acid induces p21 and topoisomerase-II (alpha/beta) expression and synergistically enhances etoposide cytotoxicity in human glioblastoma cell lines. J Neurooncol. 2007;85:159–70.
Byler TK, Leocadio D, Shapiro O, Bratslavsky G, Stodgell CJ, Wood RW, et al. Valproic acid decreases urothelial cancer cell proliferation and induces thrombospondin-1 expression. BMC Urol. 2012;12:21.
Soriano AO, Yang H, Faderl S, Estrov Z, Giles F, Ravandi F, et al. Safety and clinical activity of the combination of 5-azacytidine, valproic acid, and all-trans retinoic acid in acute myeloid leukemia and myelodysplastic syndrome. Blood. 2007;110:2302–8.
Michaelis M, Michaelis UR, Fleming I, Suhan T, Cinatl J, Blaheta RA, et al. Valproic acid inhibits angiogenesis in vitro and in vivo. Mol Pharmacol. 2004;65:520–7.
Isenberg JS, Jia Y, Field L, Ridnour LA, Sparatore A, Del Soldato P, et al. Modulation of angiogenesis by dithiolethione-modified NSAIDs and valproic acid. Br J Pharmacol. 2007;151:63–72.
Zgouras D, Becker U, Loitsch S, Stein J. Modulation of angiogenesis-related protein synthesis by valproic acid. Biochem Biophys Res Commun. 2004;316:693–7.
Gao D, Xia Q, Lv J, Zhang H. Chronic administration of valproic acid inhibits PC3 cell growth by suppressing tumor angiogenesis in vivo. Int J Urol. 2007;14:838–45.
Kitazoe K, Abe M, Hiasa M, Oda A, Amou H, Harada T, et al. Valproic acid exerts anti-tumor as well as anti-angiogenic effects on myeloma. Int J Hematol. 2009;89:45–57.
Kim MS, Kwon HJ, Lee YM, Baek JH, Jang JE, Lee SW, et al. Histone deacetylases induce angiogenesis by negative regulation of tumor suppressor genes. Nat Med. 2001;7:437–43.
Luo HM, Du MH, Lin ZL, Zhang L, Ma L, Wang H, et al. Valproic acid treatment inhibits hypoxia-inducible factor 1alpha accumulation and protects against burn-induced gut barrier dysfunction in a rodent model. PLoS One. 2013;8:e77523.
Chen SY, Sang NL. Histone deacetylase inhibitors: the epigenetic therapeutics that repress hypoxia-inducible factors. J Biomed Biotechnol. 2011;2011:197946.
Wang JH, Wang JC, Sun YX, Song WY, Nor JE, Wang CY, et al. Diverse signaling pathways through the SDF-1/CXCR4 chemokine axis in prostate cancer cell lines leads to altered patterns of cytokine secretion and angiogenesis. Cell Signal. 2005;17:1578–92.
Zhou CL, Yu XJ, Chen LM, Jiang H, Li CY. Corticotropin-releasing hormone attenuates vascular endothelial growth factor release from human HaCaT keratinocytes. Regul Pept. 2010;160:115–20.
Author information
Authors and Affiliations
Corresponding author
Ethics declarations
Conflict of interest
The authors declare no conflict of interest.
Rights and permissions
About this article
Cite this article
Zhao, Y., You, W., Zheng, J. et al. Valproic acid inhibits the angiogenic potential of cervical cancer cells via HIF-1α/VEGF signals. Clin Transl Oncol 18, 1123–1130 (2016). https://doi.org/10.1007/s12094-016-1494-0
Received:
Accepted:
Published:
Issue Date:
DOI: https://doi.org/10.1007/s12094-016-1494-0