Abstract
Textiles are known to be the best substrates for growing a variety of microorganisms efficiently at appropriate temperatures and humidity in contact with the human body. Currently, increasing public concern about hygiene has been driving many investigations about antimicrobial surface modification of textiles. The present research reports on the synthesis and characterization of nanosized copper oxide nanoparticles (CuONPs) and their application on cotton fabric to increase the bactericidal and hydrophobic properties. The synthesized materials have been subjected to spectroscopic and microscopic characterizations to help in understanding their structure, morphology, size, and composition. Further, upon dispersion of the nanoparticles onto the fabric, its hydrophobicity and mechanical properties were evaluated using electron microscopy and universal testing machine. Treated cotton fabric exhibits higher tensile strength (32 MPa) than the untreated one (27 MPa), whereas copper nanoparticle-coated cotton fabric shows a fair hydrophobicity. Moreover, CuONPs-treated and untreated cotton fabrics have been analyzed for bactericidal activity against various gram-negative and gram-positive strains. Finally, the CuONPs-coated cotton fabric displays greater antibacterial activity against E. coli and exhibits superior antimicrobial activity even after 30 cycles of washing, indicating that the CuONPs-coated cotton fabric has a higher potential to be employed as a medical textile to avoid cross-infection within a clinical environment.
Similar content being viewed by others
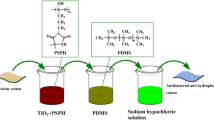
Explore related subjects
Discover the latest articles, news and stories from top researchers in related subjects.Avoid common mistakes on your manuscript.
Introduction
Antibacterial agents are compounds used to inhibit bacterial growth, and their modus operandi interrupts replication, transcription, and translation in the cell.1 It deteriorates the metabolic process of the bacteria and ends with mortality.2 These antibacterial agents have several industrial applications such as textiles, dermatological therapies, dental composites, food packaging and medicine that are of increasing demand nowadays. Several studies have reported that the use of antibiotics has led to the emergence of multidrug-resistant bacterial strains. Mainly, antibiotics attack bacteria in three different ways: (1) they inhibit the cell wall synthesis (2) disturb the translational mechanism and (3) denature the enzymes for DNA replication.3 By integrating nanotechnology and biotechnological techniques, it is possible to overcome the antibiotic-resistant problem with the use of nanoscale metals through green processes that have a huge potential in the field of medicine.4,5 One of the most important technologies emerging with the use of nanoparticles is antimicrobial textile technology.6 Nutrients present in the natural fibers are the major growth-promoting factors for many bacterial strains, especially cotton fabric.7 Cotton fabric must be sterile to avoid the dangerous infections caused after injury, or it can be treated with antibacterial agents. Cotton coated with NPs is known to inhibit the growth and avoid any associated infections.8 The leaves of Carica papaya possess potential biological antioxidants such as papain, chymopapain, flavonoids, and ascorbic acid. These components act as reductants for the formation of nanoparticles. The papaya leaf contains chemicals such as karpain, which interfere with the digestive function of microorganisms and kills them.9,10
Copper has the ability to inhibit the growth of microbes, fungi, and algae, as it plays an important role in the disinfection of water. Copper oxide nanoparticles (CuONPs) can be prepared by several well-known techniques such as sol–gel, quick precipitation, and chemical reduction.11 However, these methodologies have certain limitations as they use harsh chemicals that are toxic to human beings. To avoid toxicity, the best known practice is to make use of natural sources. Among the various natural methods known, plants are the best option as they are eco-friendly, cost-effective, more stable, mild, and nontoxic.12,13,14 Textile surface finishing is one of the new emerging technologies in the field of nanotechnology. Recent research interest toward the antibacterial textiles is increasing, and this plays a significant role in evading bacterial infections, especially during wound dressing and application on hospital clothing, where bacterial infections can be highly prevalent.15,16
The present research focuses on the synthesis of copper oxide nanoparticles from the green plant Carica papaya leaves and has reported its antimicrobial activity. Furthermore, nanocoated cotton fabric was also evaluated against gram-positive and gram-negative bacteria. The availability of copper has made it a better choice to work with as it shares similar properties as other expensive metals such as silver and gold. Carica papaya plant leaves have an inherent antimicrobial activity, but the combination of metal ions with plant phenols and phytochemicals increases their antimicrobial activity by several folds.
Materials and methods
Collection of plant material
Fresh leaves of Carica papaya were collected from the Rajkot district, Gujarat, India. All chemicals used in the experiment were purchased from HI Media, and all the assays were performed in triplicate.
Preparation of plant extracts
The Carica papaya plant parts were washed with distilled water (D/W) and dried in the sun to remove the residual moisture for the formulation of the extract. The dried leaves were finely ground using a mixer. Leaf extract was prepared by the addition of 6 g of dried powder in 100 mL of distilled water and boiled at 60°C for 10 min. The mixture was continuously stirred on a magnetic stirrer for 15–20 min and was cooled to room temperature followed by filtration, and stored at − 20°C for further downstream analysis.17,18,19,20
Green synthesis of CuONPs
CuONPs were synthesized by adding 0.01 M CuSO4 solution to the Carica papaya leaf extract in the volume ratio 95:5 by a reduction process. This mixture was stirred for 15 min and allowed to stand at room temperature for 5–10 min to obtain a colloidal suspension which turned green from blue with a brownish-black precipitate. The mixture was centrifuged and washed several times with distilled water and dried at 50°C in a hot air oven for a day to obtain the CuONPs. Carica papaya leaves have the best reduction capability against copper sulfate when compared to other parts of the plants (seeds and fruit) which was observed by the external color change.21
Characterization
Analysis using UV–visible spectra
The bioreduction of CuSO4 to CuONPs was monitored periodically by UV–visible spectroscopy. This refers to absorption spectroscopy in the UV–visible spectral region. After the dilution of the samples with deionized water, UV–visible spectrometric readings were recorded in the range of 200–700 nm.22,23
FTIR analysis
Fourier transform infrared (FTIR) spectroscopy was used to identify the possible biomolecules, and the functional group was responsible for the reduction of Cu+2 ions and capping of the reduced CuO nanoparticles. For FTIR analysis (FTIR Nicolet 5700, Thermo Corp., USA), samples were prepared by mixing 1% (w/w) specimen with 100 mg of KBr powder and pressed into a sheer slice.23 An average of 32 scans were collected for each measurement with a resolution of 2 cm−1. The FTIR spectrometric readings were recorded in the range of 4000–650 cm−1.24
X-ray diffraction analysis (XRD)
The crystal structure of the CuONPs was determined by analyzing the x-ray diffraction patterns obtained using Cu Kα (λ = 1.5417 Å) radiation on PANalytical X’Pert Pro x-ray diffractometer at the Department of Physics, Saurashtra University, Rajkot. The XRD machine was operated at a voltage of 40 kV and 30 mA. The measurements were taken with 0.02 steps and 1 s time per step at room temperature.25
Scanning electron microscopy (SEM) and transmission electron microscopy (TEM)
Scanning electron microscopy and transmission electron microscopy were used to check the size and surface morphology of nanoparticles as well as to study the surface characteristics of cotton fabric treated with CuONPs.26
Antimicrobial activity of CuONPs
The effect of copper oxide nanoparticles synthesized using C. papaya plant extract with standard antibiotic kanamycin was tested against gram-positive and gram-negative bacterial strains using agar well diffusion method. Luria–Bertani (LB) broth medium was used to grow a single colony of each bacterial strain and kept overnight on a rotary shaker at 37°C. The plates containing LB agar medium were inoculated with 100 µL of the overnight-grown activated culture of each strain. To determine the bacterial activity, the agar wells were inoculated with antibiotic kanamycin (30 µg/disk) as a positive control, 30 µl distilled water as a negative control, 30 µl of 0.01 M CuSO4 solution, 30 µl of plant extract and tested against 30 µg of CuONPs synthesized using C. papaya plant extract. The plates were then incubated at 37°C for 24 h and observed for the zone of inhibition.27
Evaluation of the bactericidal activity of CuONPs on cotton fabric
A colloidal solution of CuONPs with 100 mg/L concentration was used to coat cotton fabric using a dip-coating setup. After padding, treated samples were squeezed and dried in an oven at 80°C for 15–20 min. Antimicrobial activity of the cotton fiber and cotton fabric was evaluated against gram-positive bacterial strains such as Bacillus subtilis (MCC 2244), Bacillus megaterium (MCC 2336), Bacillus cereus (MCC 2240), Staphylococcus epidermidis (MCC 2044), and gram-negative bacterial strains such as Escherichia coli (MCC 2246), Klebsiella pneumonia (MCC 2716), Klebsiella oxytoca (ATCC 43165), and Salmonella typhimurium (ATCC 14028) using the agar well diffusion method. To evaluate the antimicrobial property of the cotton fabric, samples were subjected to agar well diffusion method.28 The plates containing LB agar medium were inoculated with 100 µL of the overnight-grown activated culture of each strain. Each cotton fabric sample coated with copper oxide nanoparticles was planted on an agar plate, and the plates were incubated at 37°C for 24 h. The zone of inhibition of treated cotton fabric with CuONPs and the untreated cotton fabric was measured.29,30,31
Textile properties of cotton fabric
Washing fastness
AATCC 61(2A) (American Association of Textile Chemists and Colorists) 1996 test method was used to test the washing fastness of treated cotton fabric with CuONPs. The antimicrobial stability of cotton fabric was checked up to 30 cycles of washing.32
Tensile strength
Tensile strength of the cotton fabric was measured using ASTM test method D5035. The strip test method was applied on the cotton fabric of 2.5 cm × 8 cm with the use of tensile testing machine. Tensile strength was measured in terms of determination of the breaking load and breaking elongation.32
Water absorption
Water absorption capacity of treated fabric with CuONPs and the untreated fabric was checked by AATCC test method 79-2000.32
Results and discussion
Spectral analysis
UV–visible spectroscopy was used to detect the surface plasmon resonance property of CuONPs. It never shows absorption in the visible range; however, distinct broad bands are observed at ~ 264, 280, 288, and 295 nm, according to Mie’s theory (dipole oscillation). The increase in the intensity of the peak around the maximum value could be attributed to the increasing concentration of the reducing agent. The solvent type used for the preparation of nanoparticles, pH, stabilizing agent used, and particle size are responsible for the exact position of the plasmon absorption. Here, CuONPs showed no surface plasmon resonance (Fig. 1). A sharp peak observed at 270 nm indicates that the particles are smaller in size which is in line with the observations reported by Kayani et al.33
FTIR spectrum of the CuONPs displays peaks at 1623 cm−1, 1382 cm−1, 1034 cm−1, and 2922 cm−1 (Fig. 2). These peaks can be attributed to the C=O bond (1382 cm−1), H–OH bending (1623 cm−1), and OH bond stretching (2922 cm−1), respectively (Table 1). A recent study reports on a similar pattern for CuONPs synthesized by sol–gel method.34 Previous studies have also reported the same for copper oxide nanoparticles using gum karaya.35
Figure 3 shows the x-ray diffraction spectra of CuONPs synthesized from Carica papaya leaf extract. The x-ray diffraction pattern was recorded in the 2θ range of 20°–80°. The sharp peaks in the diffraction patterns indicate the crystalline nature of the sample. Analysis of the spectra indicates that the sample contains some amount of Cu2O due to the absorption of excess amounts of oxygen during the synthesis process. Few intense crystallographic reflections such as (110), (111), (020), (202) of CuONPs are shown in Fig. 3. Our result suggests the mixed phase formation of the CuONPs.
The morphology and size of CuONPs were further characterized using SEM and TEM histogram. The size of the NPs was found to be less than 100 nm which may have probably contributed to their unique antimicrobial activity (Fig. 4a). The CuONPs seem to have been evenly distributed on the cotton fabric as evidenced from the SEM image (Fig. 4b). The surface morphology of CuONPs from TEM imaging reveals that most of the particles are present in the form of a square or rectangle (Figs. 5a and 5b).
Antimicrobial activity
Antimicrobial activity of CuONPs was tested using agar well diffusion method. The study demonstrated a higher antimicrobial property of CuONPs as compared to the plant extract and CuSO4 (Figs. 6 and 7). The study found similar bactericidal activity of CuONPs as positive control in kanamycin. CuONPs showed lesser zone of inhibition in Staphylococcus aureus and Bacillus subtilis (gram-positive) than E. coli (gram-negative). The difference in the zone of inhibition may be due to the cell wall composition of gram-positive and gram-negative bacteria because of the thick and wide peptidoglycan layer in the cell wall of gram-positive bacteria than the cell wall of gram-negative bacteria.36,37,38
Antibacterial evaluation of surface finished textile
Disk diffusion method was used for the antimicrobial assay of CuONPs and cotton fabric. Generally, the traditional cotton textile does not show any antibacterial resistance, as nutrients present in the natural fiber lead to accumulation of growth of microorganisms. The present study observed no zone of inhibition for untreated cotton fabric and excellent bactericidal activity for the surface-treated cotton with CuONPs (Fig. 9). The mechanism behind the bacterial resistance is that free Cu ions released from CuONPs get easily absorbed by the bacteria (Figs. 8 and 9).
CuONPs exhibit high bactericidal effects because of their interaction with the cell membrane of microbes.39 CuONPs play an important role in denaturation of intracellular proteins, DNA, and cell wall by electrostatic interaction. CuONPs produce reactive oxygen species (ROS) by creating oxidative stress in the cell which ends up with lipid peroxidation, protein oxidation, DNA degradation and finally creating necrosis of the cell.15,40
Textile properties of cotton fabric
Washing fastness
The present study assessed the washing fastness of treated cotton fabric after 10, 20 and 30 cycles of washing for antibacterial stability (Fig. 10). It showed negligible effect of washing on the surface of cotton fabric when treated with CuONPs due to its higher insolubility in water. Although antimicrobial activity of cotton fabric displayed a slight decrease after 30 washing cycles, multiple washing cycles have shown excellent antibacterial stability.
Water absorption
Water absorption capacity of treated fabric with CuONPs and untreated fabric was checked by AATCC test method 79-2000. The absorption time for untreated fabric and treated fabric was found to be 10 s and 127 s to absorb one droplet of water, respectively. CuONPs create blockage at the hydrophilic site of the cotton fabric due to their binding capacity with cellulose fibers. Hence, CuONPs-treated cotton fabric shows a higher hydrophobicity than the untreated cotton fabric. Similar results were also reported by earlier studies for silver and copper nanoparticles.32
Tensile strength
The tensile strength of cotton fabric was measured with the help of universal testing machine (UTM). Untreated cotton fabric showed 37 mm breaking elongation when 4000 N breaking load was applied, whereas treated fabric showed 53 mm elongation when 4800 N breaking load was applied (Table 2). The present study indicates that surface finishing with CuONPs increases the tensile strength of cotton fabric due to incorporation of copper ions into the cotton fabric.
Conclusion
CuONPs have been successfully synthesized by using Carica papaya leaf extract. Different characterization techniques were used to study their structure, morphology binding and mechanical properties. The SEM analysis indicates that the CuONPs were well dispersed on the cotton fabric and exhibit good antimicrobial activity against gram-negative E. coli. The cotton fabric surface containing CuONPs displayed excellent bacterial resistance even after 30 cycles of washing. Textile surface finishing with CuONPs also increased the fabric hydrophobicity and tensile strength. The antimicrobial activity of the synthesized CuONPs via green approach is a promising candidate for the medicinal and food industries. Yet there is a strong need for its in vivo studies using CuONPs before it is practically applied in the field.
References
Clardy, J, Fischbach, MA, Currie, CR, “The Natural History of Antibiotics.” Curr Biol., 19 437–441 (2009)
Hajipour, MJ, Fromm, KM, Ashkarran, AA, de Aberasturi, DJ, de Larramendi, IR, “Antibacterial Properties of Nanoparticles.” Trends Biotechnol., 30 499–511 (2012)
Usman, MS, El Zowalaty, ME, Shameli, K, Zainuddin, N, Salama, M, “Synthesis, Characterization, and Antimicrobial Properties of Copper Nanoparticles.” Int. J. Nanomedicine., 8 4467–4479 (2013)
Hsueh, PR, “New Delhi Metallo-ß-lactamase-1 (NDM-1): An Emerging Threat Among Enterobacteriaceae.” J. Formos Med. Assoc., 109 685–687 (2010)
Poole, K, “Mechanisms of Bacterial Biocide and Antibiotic Resistance.” J. Appl Microbiol., 92 55–64 (2002)
Kamyar, S, Mansor, BA, Wan Md Zin Wan, Y, Abdolhossein, R, Nor Azowa, I, Mohsen, Z, “Highly Effective Antibacterial Textile Containing Silver Nanoparticles.” Int. J. Nanomed., 5 875–887 (2010)
Gao, Y, Cranston, R, “Recent Advances in Antimicrobial Treatments of Textiles.” Text. Res. J., 78 60–72 (2008)
Jayaraman, R, “Antibiotic Resistance: An Overview of Mechanisms and a Paradigm Shift.” Curr. Sci., 96 1475–1484 (2009)
Otsuki, N, Dang, NH, Kumagai, E, Kondo, A, Iwata, S, Morimoto, C, “Aqueous Extract of Carica papaya Leaves Exhibits Anti-tumor Activity and Immunomodulatory Effects.” J. Ethnopharmacol., 127 (3) 760–767 (2010)
Sankar, R, Manikandan, P, Malarvizhi, V, Fathima, T, Shivashangari, KS, Ravikumar, V, “Green Synthesis of Colloidal Copper Oxide Nanoparticles Using Carica papaya and Its Application in Photocatalytic Dye Degradation.” Spectrochim. Acta Part A Mol. Biomol. Spectrosc., 121 746–750 (2014)
Knetsch, ML, Koole, LH, “New Strategies in the Development of Antimicrobial Coatings: The Example of Increasing Usage of Silver and Silver Nanoparticles.” Polymers, 3 340–366 (2011)
Chatzimitakos, TG, Stalikas, CD, “Qualitative Alterations of Bacterial Metabolome after Exposure to Metal Nanoparticles with Bactericidal Properties: A Comprehensive Workflow Based on 1H NMR, UHPLC-HRMS, and Metabolic Databases.” J. Proteome Res., 15 3322–3330 (2016)
Shameli, K, Ahmad, BM, Jaffar Al-Mulla, EA, “Green Biosynthesis of Silver Nanoparticles Using Callicarpa maingayi Stem Bark Extraction.” Molecules, 17 8506–8517 (2012)
Balazs, AC, Emrick, T, Russell, TP, “Nanoparticle Polymer Composites: Where Two Small Worlds Meet.” Science, 314 1107–1110 (2006)
Adonizio, A, Kong, KF, Mathee, K, “Inhibition of Quorum Sensing-Controlled Virulence Factor Production in Pseudomonas aeruginosa by South Florida Plant Extracts.” Antimicrob. Agents Chemother., 52 198–203 (2007)
Ahmad, A, Mukherjee, P, Senapati, S, Mandal, D, Khan, MI, Kumar, R, Sastry, M, “Extracellular Biosynthesis of Silver Nanoparticles Using the Fungus Fusarium oxysporum.” Colloids Surf. B Biointerfaces, 28 313–318 (2003)
Neely, AN, Maley, MP, “Survival of Enterococci and Staphylococci on Hospital Fabrics and Plastic.” J. Clin. Microbiol., 38 724–726 (2000)
Daren, AJ, Bonden, JM, “Vancomycin-Resistant Enterococci in Intensive Care Hospital Settings.” Mem inst oswaldo Cruz, Rio de Jamino, 93 587–588 (1998)
Kanagasubbulakshmi, S, Kadirvelu, K, “Green Synthesis of Iron Oxide Nanoparticles Using Lagenaria siceraria and Evaluation of Its Antimicrobial Activity.” Defence Life Sci. J., 2 422–427 (2017)
Pattanayak, M, Nayak, PL, “Green Synthesis and Characterization of Zero Valent Iron Nanoparticles from the Leaf Extract of Azadirachta indica (Neem).” World J. Nano Sci. Technol., 2 06–09 (2013)
Gottimukkala, KSV, Harika, RP, Zamare, D, “Green Synthesis of Iron Nanoparticles Using Green Tea Leaves Extract.” J. Nanomed. Biother. Discov., 7 151 (2017)
Balamurughan, MG, Mohanraj, S, Kodhaiyoli, S, Pugalenthi, V, “Ocimum Sanctum Leaf Extract Mediated Green Synthesis of Iron Oxide Nanoparticles: Spectroscopic and Microscopic Studies.” J. Chem. Pharmaceut. Sci., 4 201–204 (2014)
Turakhia, B, Turakhia, P, Shah, S, “Green Synthesis of Zero Valent Iron Nanoparticles from Spinacia oleracea (Spinach) and Its Application in Waste Water Treatment.” J. Adv. Res. Appl. Sci., 5 (1) 46–51 (2018)
Turakhia, B, Chikkala, S, Shah, S, “Novelty of Bioengineered Iron Nanoparticles in Nanocoated Surgical Cotton: A Green Chemistry.” Adv. Pharmacolog. Sci., (2019).
Sivaraj, R, Rahman, PK, Rajiv, P, Narendhran, S, Venckatesh, R, “Biosynthesis and Characterization of Acalypha indica Mediated Copper Oxide Nanoparticles and Evaluation of Its Antimicrobial and Anticancer Activity.” R Spectrochim. Acta A Mol Biomol. Spectrosc., 129 255–258 (2014)
Zhou, K, Wang, R, Xu, B, Li, Y, “Synthesis, Characterization and Catalytic Properties of CuO Nanocrystals with Various Shapes.” Nanotechnology, 17 68–78 (2006)
Wiegand, I, Hilpert, K, Hancock, RE, “Agar and Broth Dilution Methods to Determine the Minimal Inhibitory Concentration (MIC) of Antimicrobial Substances.” Nat. Protoc., 3 163–175 (2008)
Xu, JF, Ji, W, Shen, ZX, “Preparation and Characterization of CuO Nanocrystals.” J. Solid State Chem., 147 516–519 (1999)
Abdel-Aziz, MS, Shaheen, MS, El-Nekeety, AA, Abdel-Wahhab, MA, “Antioxidant and Antibacterial Activity of Silver Nanoparticles Biosynthesized Using Chenopodium murale Leaf Extract.” J. Saudi Chem. Soc., 18 356–363 (2014)
Janardhanan, SK, Ramasamy, I, Nair, BU, “Synthesis of Iron Oxide Nanoparticles Using Chitosan and Starch Templates.” Transit. Met. Chem., 33 127–131 (2008)
Shishtawy, E, Asiri, RM, Abdelwahed, AM, Al-Otaibi, MM, “In Situ Production of Silver Nanoparticle on Cotton Fabric and Its Antimicrobial Evaluation.” Cellulose, 18 75–82 (2011)
Patel, BH, Chaudhari, SB, Patel, PN, “Nano Silica Loaded Cotton Fabric; Characterization and Mechanical Testing.” Res. J. Eng. Sci., 3 19–24 (2014)
Kayani, ZN, Umer, M, Riaz, S, Naseem, S, “Characterization of Copper Oxide Nanoparticles Fabricated by the Sol-Gel Method.” J. Electron. Mater., 44 3704–3709 (2015)
Padil, VVT, Černík, M, “Green Synthesis of Copper Oxide Nanoparticles Using Gum Karaya as a Biotemplate and Their Antibacterial Application.” Int. J. Nanomed., 8 889 (2013)
Das, D, Nath, BC, Phukon, P, Dolui, SK, “Synthesis and Evaluation of Antioxidant and Antibacterial Behavior of CuO Nanoparticles.” Colloids Surf. B Biointerfaces, 101 430–433 (2013)
Heinlaan, M, Ivask, A, Blinova, I, Dubourguier, HC, Kakru, A, “Toxicity of Nanosized and Bulk ZnO, CuO and TiO2 to Bacteria Vibrio fischeri and Crustaceans Daphnia magna and Thamnocephalus platyurus.” Chemosphere, 71 1308–1316 (2008)
Tawale, JS, Dey, K, Pasricha, R, Sood, KN, Srivastava, AK, “Modification at Lattice Scale for an Optimized Optical Response of Alx(ZnO)1-x Nanostructures.” Thin Solid Films, 519 1244–1247 (2010)
Ghosh, S, Yadav, S, Reynolds, N, “Antibacterial Properties of Cotton Fabric Treated with Silver Nanoparticles.” J. Text. Inst., 101 917–924 (2010)
Tiwari, DK, Behari, J, Sen, P, “Application of Nanoparticles in Waste Water Treatment.” World Appl. Sci. J., 3 417–433 (2008)
Chatterjee, AK, Chakraborty, R, Basu, T, “Mechanism of Antibacterial Activity of Copper Nanoparticles.” Nanotechnology, 25 135101 (2014)
Author information
Authors and Affiliations
Corresponding authors
Additional information
Publisher's Note
Springer Nature remains neutral with regard to jurisdictional claims in published maps and institutional affiliations.
Rights and permissions
About this article
Cite this article
Turakhia, B., Divakara, M.B., Santosh, M.S. et al. Green synthesis of copper oxide nanoparticles: a promising approach in the development of antibacterial textiles. J Coat Technol Res 17, 531–540 (2020). https://doi.org/10.1007/s11998-019-00303-5
Published:
Issue Date:
DOI: https://doi.org/10.1007/s11998-019-00303-5