Abstract
This study was aimed to investigate the inhibition of the deteriorations of rice bran by infrared radiation (IR) heating and its effects on the chemical and biological profiles during short-term storage. Freshly milled paddy rice bran was exposed to the IR until the surface temperature reached 85 °C. The results indicated that after 20 days of storage, the activities of relevant enzymes, including lipase, lipoxygenase, and peroxidase all decreased after IR-treated. The rising trend of the free fatty acid content decreased significantly during storage. IR treatment can reduce the oxidation and hydroperoxides of rice bran, which can control the rise of peroxide value till the 15 days of storage (9.7 meq/kg). The contents of palmitic acid (C16:0), oleic acid (C18:1), and linoleic acid (C18:2) were well maintained by IR heating during the whole storage period. The aroma of fresh rice bran was acceptably preserved with abundant amount of aldehydes and alkanes. After quantification of the volatile chemical compounds in rice bran, potential biomarkers specific for rice bran storage should be highlighted, being 3-octen-2-one, acetophenone, 2,4-dimethylcumene, 5,6-dimethylindane, and phytane. This study illustrates that the IR processing can effectively guarantee the stabilization of rice bran in terms of desirable FAs profiles, relevant enzymes activities, and aroma features.

Graphical Abstract
Similar content being viewed by others
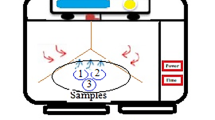
Explore related subjects
Discover the latest articles, news and stories from top researchers in related subjects.Avoid common mistakes on your manuscript.
Introduction
Rice bran is a by-product of the grain milling process, representing 5–8% of the total grain (Silventoinen et al. 2019). It is a natural source of protein (12–16%), fat (15–20%), crude fiber (23–28%), ash (7–8%), carbohydrates, vitamins, minerals, essential unsaturated fatty acids, and phenolic compounds (Zhu et al. 2017; Patil et al. 2016). It also has remarkable contents of naturally occurring antioxidants such as tocopherols, tocotrienols, and γ-oryzanol, which are known to have hypocholesterolemic effects and help lower the incidences of oxidative-stress-related diseases like cancer, cardiovascular disorders, inflammation, aging, and obesity (Chua et al., 2019; Kong et al. 2012). Therefore, rice bran, especially its natural nutrients, has important preventive and therapeutic significance for human health. However, despite its high nutritional value, rice bran is currently underutilized and frequently wasted, except for producing oil. The single most factor restricting its use as a food ingredient is its instability during storage. This instability is generally attributed to the lipase enzymes present in the outer layers of the rice kernel, which is primarily responsible for the hydrolysis of triglycerides into glycerol and free fatty acids (FFAs) (Shen et al. 1997). When rice is milled, the bran is scoured with the lipase exposed to the oxygen in air. Then, the oxidation starts, resulting in oil quality deterioration and off-flavor forming (Eshghi et al. 2014). The FFAs in the oil usually exceed 10% in only less than 1 week, and then, the rice bran oil is no longer suitable for human consumption (Qian et al. 2014). Besides, both lipoxygenase and peroxidase are also the key enzymes responsible for the deterioration of rice bran, although to a lesser extent (Orthoefer 2005). Therefore, it is a normal and meanwhile effective practice that the bran undergoes a stabilization process to inactivate the potent lipase enzymes immediately after milling to extend the storage time at ambient conditions with FFA concentration controlled at a low level (less than 10%) before oil extraction (Wang et al. 2017).
Currently, several approaches have been investigated to stabilize rice bran, including the chemical methods (Xia et al., 2020) and heating process (Peanparkdee et al. 2018, Kim et al. 2014). However, these stabilization processes are typically time-consuming and may not be appropriate for the on-line system and industry purposes. Infrared radiation (IR), as one of the newly emerging food processing technologies, has gradually drawn the attention of researchers for its remarkable reduction of technology costs. It may offer a promising potential in achieving efficient drying and simultaneously inactivating the lipase in rice bran without affecting its quality. When IR is used to heat or dry moist materials, the radiation impinges on the exposed material and penetrates it, and then the radiation energy is converted into heat (Krishnamurthy et al., 2008). The penetration could provide more uniform heating in the kernel of rice bran, and may reduce the moisture gradient during heating and drying. Our previously consecutive studies have confirmed that IR can be an effective approach to extend the storage stability and lives for both rough and brown rice by achieving high drying efficiency, good milling quality, and simultaneously inactivating the lipases, and thus extending the storage (Ding et al. 2015; Ding et al., 2018). This suggests that IR might provide us a more effective way to improve the utilization of rice bran without affecting its oil quality (Yılmaz et al. 2014). However, to date, there is no literature systematically investigating the effects of IR heating on the preservation of rice bran. In addition, the underlying mechanisms are also poorly understood.
Considering this, this work aimed to investigate the effects of IR heating on the biological and chemical changes of rice bran, including (1) the activities of key enzymes, (2) the FFA concentration and peroxide value (POV) of the rice bran oil over the storage periods, (3) the composition of volatiles and FAs, and (4) sensory aroma characteristics. Then, the relationships between lipid oxidation and volatile compound composition were discussed with the underlying mechanisms explored.
Materials and Methods
Samples
As one of the main cultivated japonica rice in Jiangsu province in China, freshly harvested var. No.5 Huaiyin japonica rice was selected in this study. It was obtained from Shibuqiao Grain Reserve Depot (Nanjing, Jiangsu province). Then, freshly milled rice bran was collected from a commercial rice mill, and was cleaned using BS sieve no.40 (425 μm aperture) to remove the broken pieces of rice, husk, and other foreign materials. The moisture content (MC) of rice bran was 8.73 ± 0.10% in dry basis (d.b.). All MC of rice bran was determined by the standard air oven method at 105 °C for 90 min (ASAE 1995) in an electric dry oven (Model 101-3AS, Sujin Instrument Factory, Shang Hai, China), and was expressed as percentage in dry mass basis with triplicates. Subsequently, rice bran was packed in air tight double-layered polythene bag, and was stored at − 20 °C to prevent the hydrolysis of FAs by lipases.
IR heating treatment
A laboratory-scale ceramic infrared drying device provided by Maybo Innovation (MB-EHR12/10KW, Zhen Jiang) was used to treat the rice bran. The infrared drying device consists of an IR emitter, a circulating fan, a sample holder, and a control panel (Pei et al. 2018). The IR emitter is the source of IR radiation (HFS, Elstein-Werk, Germany). It has a maximum surface temperature of 630 °C, and a corresponding peak wavelength of 3.2 mm assuming a blackbody. The temperature of the IR emitter could be continuously adjusted during the range of 20 to 630 °C based on the experiment requirements. The rice bran was separately placed in one-layer steel container (size, length × width × height = 21 × 9 × 1.2 cm). To eliminate the inhomogeneous heating for IR processing, total 50 g sample was equally tiled on the bottom of container for 2 ± 0.5 mm thickness.
Before using, the IR emitter was adjusted to the preset temperature (300 °C) to warm up the device. After 20 min of preheating, the temperature of the IR device became stable. Then, the rice bran samples were placed in single layer at a 15-cm distance under the IR emitter. Then, they were heated to until the surface temperature reached 85 °C. The temperature of the IR emitter was controlled using the control panel. The temperature of the heated rice bran was measured using a type-K thermocouple and RDXL4SD thermometer (time constant of 0.15 s, OMEGA Engineering Inc., Stamford, USA). Four thermocouples were equidistantly fixed and were placed inside the rice bran layer to continuously measure the temperature.
Storage and testing
To imitate the actual shelf storage conditions, the rice bran was packed with air-tight double-layered polythene bag, and then was stored in an incubator at the temperature of 20 ± 2 °C. Each 100 g samples was randomly selected from IR and CK groups at different time points (0, 5, 10, 15, and 20 days), and then transferred to refrigerator at − 20 °C for subsequent analysis. Each analysis was carried out in triplicate, and the mean value was calculated and used in further analysis.
Preparation of rice bran oil
Bran samples were extracted with n-hexane. Briefly, 20 g of rice bran were dispersed in n-hexane (300 mL). Then, the dispersion was magnetic-stirred at room temperature (20 °C) for 1 h. After stirring, the beaker was placed in the fume hood for 30 min, and the supernatant liquid was later extracted. The residue was repeatedly extracted using 200 mL n-hexane. Later, both extracts were mixed and were centrifuged at 4000g for 30 min using a centrifuge (TG16-WS, Changsha, China). The supernatant was collected in the round bottom flask. Then, it was evaporated until n-hexane was removed using a rotary evaporator under vacuum system at 30 °C. The dried extracts were then analyzed for FFA concentration and POV. The defatted rice bran is naturally dried in the fume hood to determinate the activities of the key enzymes.
Measurement of enzymes activities
Lipase activity
Lipase activity was determined using a spectrophotometric assay (U-3900, Hitachi, Japan) with p-nitrophenol (p-NP) as substrate. Briefly, 2 mL of p-NPP (0.09 mg/mL) substrate solution was added in a 50-mL centrifuge tube, which was preheated in a water bath at 37 °C for 5 min. Then, 0.5 mL liquid enzyme was added. After 10 min, 2.5 mL trichloroacetic acid (0.5 mol/L) was immediately added, and was mixed evenly. After 5 min, the reaction was terminated. Then, 0.5 mol/L NaOH was added until the pH reached the same before the reaction. The absorbance at 405 nm was recorded. One unit of lipase activity was defined as the micromoles of p-NP liberated by the hydrolysis of lipase liquid per minute.
Lipoxygenase activity
Lipoxygenase (LOX) activity was determined as described by Ramezanzadeh et al. (1999) with some modifications. A total of 2.9 mL of substrate was pipetted into a cuvet (10-mm light path), and was oxygenated thoroughly by bubbing oxygen for a few minutes. Then, 0.1 mL of enzyme solution was rapidly added and was well mixed. Then, the increase in absorbance at 234 nm versus the blank was recorded with a spectrophotometer (U-3900, Hitachi, Japan). One unit of LOX activity was defined as the change in the absorbance of 0.001/min in a 3-mL volume (1-cm light path) when linoleic acid was used as a substrate.
Peroxidase activity
Peroxidase (POD) activity was determined by a modified method from Schmidt et al. (2014). The rate of POD-dependent oxidation of guaiacol was measured in the presence of hydrogen peroxide (H2O2), which was determined using 0.2 ml of enzyme extract, 1 ml of 30 mM H2O2, and 2 mL of a 5-mM guaiacol solution, with the final volume of the tube being completed to 4 ml with buffer (pH = 7). The reaction lasted 10 min at 30 °C. Then, the absorbance was detected at 470 nm.
FFAs concentration and the POV value
The FFA concentration of the rice bran oil was detected using the method described by Pourali et al. (2009), and was expressed with unit in rice bran oil (g/100 g RBO). The POV was evaluated using the method proposed by Shantha and Decker (1994). The results were presented in terms of milliequivalents per kilogram of rice bran.
Major volatile compounds and FFA composition
Major volatile compounds were detected according to the method described by Liu et al. (2016). The GC (6890A; Agilent Technologies, USA) coupled to an MS system (5975C, Agilent Technologies, USA) with a HP-5MS column (30 m × 0.32 mm × 0.5 μm; Agilent, CA, USA) was utilized in this work.
The FFA profiles were characterized by measuring FA methyl esters. Extraction and methyl esterification of FFAs were carried out according to the procedures as previously described by Goffman and Bergman (2003) with some modifications. Briefly, samples (100 mg) were mixed with sodium methoxide (0.5 M, 1 mL), followed by the addition of 100 μL of methyl heptadecanoate (2 mg/L; B21161-20 mg, GC grade), which was dissolved in isooctane (I103237-500 mL, GC grade), and was used as internal standard. Then, the solvent was evaporated in vacuum, and the samples were added with 1 mL of solution with isooctane in sodium bisulfate (5%, w/v). Then, the mix solution was centrifuged at 3400g for 10 min. The obtained isooctane phase (1 μL) was preserved and was injected for further GC analysis.
The GC system (namely the Agilent 6890 N) equipped with a CD-2560 column (100 m × 0.25 mm × 0.2 μm) was used to identify the chemical composition. Conditions were set as follows: (1) the oven temperature program started at 70 °C with 5 min holding time, then increased to 200 °C at 25 °C/min, and was followed by 2 °C/min increase up to 260 °C; (2) the temperatures for injection, detector, and ion source were set at 270, 150, and 230 °C, respectively; (3) the flowing rate of the carrier gas (99.999% purified helium) was 1 mL/min, and the split ratio was 5:1; (4) MSD was operated in electron impact mode at 70 eV, using full scan m/z from 33 to 500.
Statistical analysis
The entire experiment was performed in triplicates, and the data were presented as mean values. Two way analysis of variance (ANOVA) and correlation analysis was performed using the GraphPad Prism 7 software to identify significant differences among different treatments. These calculations were performed after firstly computing the means of side-by-side replicates. Then, those means were further analyzed.
Results
Changes in the enzyme activities among different treatments
As shown in Fig. 1a, the lipase activity of the IR-treated rice bran was lower than those of the untreated (p < 0.05). In addition, depending on the storage period, the lipase activity of rice bran gradually increased and then reached the maximum values (276.86 and 251.52 U/mL) after 20 days of storage.
Storage period had a significant (p < 0.05) effect on the LOX activity of both untreated and IR-treated rice bran in most samples (Fig. 1b). Significant fluctuations were observed in LOX activity for all samples with significant changes observed at every storage time. The LOX activity in rice bran, regardless of treatment methods, did not deviate significantly after 20 days of storage. The LOX activity of untreated rice bran decreased significantly only between 10 and 15 days of storage. It was higher than that of the IR-treated one after 20 days of storage. Although a large decrease of LOX activity was observed during 20 days of storage, the trend generally increased.
Similarly, as shown in Fig. 1c, the activity of POD fluctuated. The enzyme activity of untreated rice bran was significantly higher than that of the infrared treated one (p < 0.01). After infrared treatment, the POD activity of the samples decreased significantly, and the activity was all below 15%. During the 20 days of storage, the POD activity of the IR-treated bran slightly increased to the peak values at the 10th day.
Changes in FFA concentration and POV in rice bran during storage
The above results have indicated that the IR processing can remove a large amount of moisture, as well as inhibited the enzyme activity in regard to degradation. Hence, the contents of FFAs and POV responses of the rice bran were measured immediately after the milling procedures, and are shown in Fig. 2.
The FFA content in rice bran exhibited an increased trend till the end of storage regardless of the treatment methods (Fig. 2a). At the beginning of storage, the FFA contents of in both the control check (CK) and IR rice bran were 5.41% and 5.21%, respectively, with no significant difference (P > 0.05) observed. It increased sharply to 49.87% after 20 days of storage in the CK samples, while limited increase of the FFA content was observed in the IR group (14.11% for 20 days storage). More specifically, the FFA content increased to more than 15% in both treatments during less than 5 days of storage. All IR-treatment samples exhibited significantly lower FFA contents than the CK group at the first 5 days of storage.
Figure 2b shows the changes in the POV of rice bran samples during storage. At the beginning, the POV of the CK and IR treatment was 2.67 and 2.80 meq/kg, respectively. Then, the values increased to 43.93 meq/kg (for CK) and 15.67 meq/kg (for IR), respectively, during the 20 days of storage. The POV of the CK treatment reached the maximum level of 49.10 meq/kg during the first 15 days of storage, then not significantly decreased to 43.93 meq/kg. In contrast, the POV slowly increased to near 15 meq/kg after 20 days of storage with a significant effect observed in treatment methods (p < 0.01).
Composition of FAs in rice bran
The content of FAs, including saturated fatty acids (SFA), monounsaturated fatty acids (MUFA) and polyunsaturated fatty acids (PUFA) in rice bran, was identified and quantified with the data shown in Table 1. Initially, the content of PUFA was higher than those of MUFA and SFA in rice bran. Specifically, palmitic acid (C16:0), oleic acid (C18:1), and linoleic acid (C18:2) were the major acids of the SFA, MUFA, and PUFA, respectively. These FAs were compressed over 95% of the SFA, MUFA, and PUFA, respectively. In the CK treatment, the total SFA content decreased constantly from 17.9 to 8.0 mg/g during the 20 days of storage. Similar results were also observed in the total MUFA and PUFA contents, which decreased significantly from 35.83 to 18.5 mg/g (p < 0.05), and 37.5 to 19.4 mg/g (p < 0.05), respectively. The contents of the total MUFA and PUFA were both twice higher than that of SFA among the 20 days of storage.
Dislike the results of the CK treatment, the total contents of SFA, MUFA, and PUFA in the IR-treated rice bran increased significantly by 1.2, 1.6, and 2.57 mg/g, respectively. In addition, we found that the MUFA and PUFA contents were both higher than that of SFA during 20 days of storage in treated group. The correlations among the several quality attributes are shown in Table 2. The data in the CK treatment indicated that the FA content was significantly correlated (p < 0.01) with the lipase activity. Dislike the results in CK, the contents of essential fatty acids had no significant difference with that of FFAs, POV, and the activities of key enzymes in the treated group during storage period. The results suggested that the FAs, including palmitic acid, stearic acid, oleic acid, linoleic acid, and linolenic acid, can be highly influenced by the IR pretreatment.
Composition of volatile compounds in rice bran
The chemical compounds in rice bran might be changed by the IR treatment in this experiment, so the flavor of the samples was analyzed to assess the hydrolytic rancidity using GC/MS. Forty-seven volatile compounds were identified and qualified by GC/MS from the extract of rice bran. The results are shown in Table 3. Two ester, six aldehydes, three ketones, one ethers, two alcohols, two phenols, twenty-five alkanes, and six other volatile chemical compounds are identified and quantified, which were defined as the major key volatile chemical components in rice bran. Alkanes were the major category components in CK at the beginning of the storage with a value of 3.10 ± 0.4 μg/kg. However, the content decreased significantly to 0.15 ± 0.02 μg/kg (p < 0.05) after 20 days of storage. As expected, similar results (from 3.87 ± 0.51 to 0.12 ± 0.02 μg/kg) were also found in the alkane content in the IR-treated samples, suggesting that alkanes may contribute to the fresh aroma of the samples throughout the storage. However, the total amount of aldehydes and alcohols increased significantly (p < 0.05) during the whole storage period in all samples.
The compositions of the volatile chemical compounds in both treatments were different. 3-Octen-2-one, acetophenone, 2,4-dimethylcumene, 5,6-dimethylindane, and phytane were only detected in the control, while butylated hydroxytoluene was found only in the IR-treated sample. Additionally, the contents of esters, aldehydes, and phenols in the IR-treated group were significantly higher (p < 0.05) than those in the CK.
Discussion
According to the results above, it took only less than 1 min to heat the rice bran up to the 85 °C during the IR treatment, then the lipase activity of the rice bran was significantly decreased from 145.21 to 116.66 U/mL. By comparison, the inactivation effect of lipase in treated group still maintained during 20 days of storage at 20 °C. Similar result from Li et al. (2016) also investigated the application of IR on wheat germ, and the result indicated that lipase activity of wheat germ deceased (p < 0.05) with the processing temperature and processing period. The present result also agrees with our previous studies (Ding et al. 2015; Pei et al. 2018), where IR treatment can be represented an effective processing to inactive of lipase in raw and brown rice, as well as reduce the moisture of rice kernel at the same. After IR processing, the spatial structure or three-dimensional conformation of lipase in rice bran was damaged or affected to the certain extent. Then, its activity was partially compromised, thus reducing the lipid hydrolysis in rice bran (Krishnamurthy et al. 2008; Sawai et al. 2003) investigated the inactivation of the lipase and a-amylase during treatment by IR, and found that certain enzyme reactions were inactivated by IR at the bulk temperature of 30 to 40 °C.
Similarly, the activities of LOX and POD were sharply decreased (p < 0.01) from 4.82 to 1.41 U/mL, and from 13.83 to 9.93 U/mL, respectively. From the results, the stabilization of LOX and POD activities in IR-treated rice bran both had significant decrease (p < 0.05) with that of the control during the 20 days of storage at 20 °C. Especially, the initial reducing rate for LOX and POD inactivation was both at least 40% after IR-treated immediately. Similar results have also shown that the infrared treatment was sufficient for complete inactivation the LOX in soybean samples (Yalcin and Basman, 2015). This phenomenon can be explained from the perspective of the energy absorption and moisture content in samples. When the radiant spectra impinge upon the sample surface, it can induce changes in the vibrational and rotational states of molecules, which can lead the constantly radiative heating (Krishnamurthy et al. 2008). The activities of enzymes were then significantly decreased with the processing temperature of the samples (Li et al. 2016). Moreover, previous study demonstrated that there is the significantly positive correlation between enzyme activity with the moisture content and water activity. The lipase and LOX activity of the most germ samples significantly decreased after IR-treated during the storage period. Brunschwiler et al. (2013) also found that rice bran moisture content was an important parameter for an effective lipase/esterase inactivation. In addition, Wang et al. (2017) suggested that both short and medium wave infrared drying could reduce the water activity appreciably (p < 0.01) by controlling the content of immobilized water in samples, which can reduce the enzyme activity to inhibit the oxidation of lipids in the sample. As the result, IR can quickly rise the temperature of sample at the high level which then directly destroy the structure of enzymes in the short-time period, as well as can reduce the water activity appreciably by controlling the content of immobilized water in samples (Ramezanzadeh et al. 1999). For a better understanding of the potential mechanisms, the activities of lipase, LOX, and POD should be investigated in the IR-treated bran with different moisture content, as warrants further studies.
Based on the above result, the storage stability (including FFA content and POV) was taken into consideration. The initial FFA content in treated and CK samples was very close, with 5.41% and 5.21%, respectively. The POVs of treated and CK in the initial storage period (0 d) in rice bran are 2.80 ± 1.04 and 2.67 ± 0.58 meq/kg, respectively. It is known that the multiple effects of the external (such as light, temperature, oxygen) and internal environments (such as moisture content and enzyme activity) affect rate of oxidation (Jaisut et al., 2009; Irakli et al., 2018); high amounts of peroxide were produced during the reactions, which led to reduced quality of rice bran. Generally, the decreased concentration of FFAs can reduce the subsequent lipid oxidation and the production of aldehydes and ketones, since FFAs is the substrate of lipid oxidase, thus improving the lipid stability of rice bran. However, the rapid oxidation reaction existed in raw rice bran (data in CK group), and the FFA content increased to more than 15% during 5 days of storage. Wang et al. (2017) also study the infrared radiation heating on rough rice and rice bran. Their results showed that it takes only less than 7 days to make the FFAs in the rice bran oil exceed 10%, and then oil is no longer acceptable for human consumption. Similar results also be found in POV in fresh rice bran during storage. As expect, lower FFA content and POV during storage were achieved using IR heating. After 20 days of storage, the corresponding FFA content and POV were 14.11 and 15.67 meq/kg, respectively. It is important to notice that the considerable enzyme inactivation reduced the oxidation and hydroperoxides in the rice bran. According to Codex Alimentarious Commission, the acceptable limitation of POV for rice bran oil is less than 10 meq/kg. Hence, results of the present study demonstrated the efficacy of IR in controlling the rise of POV till the 15 days of storage (9.7 meq/kg). Similar results were also found that IR with energy efficient heating process can induce the decomposition of some of the formed hydro-peroxide, thus resulting in the production of volatile degradation products (Eshghi et al. 2014).
During storage, lipids in rice bran break down quickly and release FFAs, which are decomposed into small-molecule volatiles. Oxidative degradation of unsaturated FAs produces ketones and aldehydes, and the oxidative degradation of linoleic acids generates hexane (Mottram, 1998). With the analysis of volatile components in rice bran, hexanal is one of the most important chemicals in action during the stabilized procedure, and constantly rises during the whole storage period. This might be attributed to the main aldehydes produced from catalytic lipid oxidation by LOX enzyme. Similar result was also found in the POV in rice bran. The secondary oxidation product, namely aldehyde ketones, is relative to the intermediate of peroxide oxidation. In addition, when rice bran was kept at a high temperature under the infrared treatment, the lipase activity decreased by 19.4% compared with the control. Since rice bran contains much protein, the Maillard reaction was liable to occur at high temperatures, leading to the changes of the color of rice bran (Ding et al. 2015). Then, the carbonyl and amino groups in rice bran would react to produce aldehydes or ketones (Irakli et al. 2018). Therefore, the content of volatile components stabilized by IR was mostly higher than that of the control, but the FFA concentration and the composition of FAs are much lower. The changes of the volatile compounds resulted from the IR processing might be used as the markers of the FFA consumption. Also, protein degradation produces sulfur compounds and the degradation of carbohydrates produces high amounts of alcohols. Changes in species and contents of volatiles could be due to the degradation of macromolecular substances in rice bran (Frankel, 1984).
Heating effects from IR rapidly raised the temperature of rice bran, as can lead to the changes of activities of the key enzymes as well as the chemical composition. The total SFA, MUFA, and PUFA in rice bran ranged from 18.2 to 39.0 mg/g in the IR treatment and 8.0 to 19.4 mg/g in control after 20 days storage. According to Curti et al. (2018), the increased contents of both MUFA and PUFA observed in rice bran could be beneficial for the reduction of chronic diseases risk, and can also that of cardiovascular disease by reducing blood lipids. From the present results, IR treatment exerted little effect on the main FA composition of rice bran. The contents of total SFA, MUFA, and PUFA increased only 1.2, 2.6, and 3.1 mg/g, respectively. However, Wanyo et al. (2015) found that the PUFA levels in both rice bran and husk decreased significantly (~ 23 mg/g). This discrepancy might be attributed to the difference in the procedures of sample preparation and IR processing. In this work, the rice bran was polished and ground from the brown rice; the total surface area in contact with air was enlarged. The oxidation deterioration of the lipids or fats in rice bran was then accelerated even in the limited storage period (Wang et al. 2017). After the IR treatment, molecules in rice bran generally absorb the radiation of the energy, affecting active vibration and generating heat. The activities for linked enzymes were sharply controlled in acceptable condition. The fresh aroma characters for rice bran were well preserved, with larger amount of both aldehydes and alkanes. It seems that the IR pretreatment applied in rice bran can be more effective than the utilized one on the paddy rice kernel based on our experiment.
Conclusion
The present study showed that the IR pretreatment was effective in stabilizing rice bran, as might be due to the decreased levels of FFA concentration and POV accompanied by the lower contents of FFAs as well as the persevered fresh volatile components. The underlying mechanisms might be related to the disruptions of membrane lipid metabolism. The present results can provide us practicable information for the stabilization of rice and its by-products to improve their utilization.
Abbreviations
- CK:
-
Control check
- d.b:
-
Dry basis
- FAs:
-
Fatty acids
- FFAs:
-
Free fatty acids
- IR:
-
Infrared radiation
- LOX:
-
Lipoxygenase
- MC:
-
Moisture content
- MUFA:
-
Monounsaturated fatty acids
- POD:
-
Peroxidase
- POV:
-
Peroxide value
- PUFA:
-
Polyunsaturated fatty acids
- RB:
-
Rice bran
- RBO:
-
Rice bran oil
- SFA:
-
Saturated fatty acids
References
ASAE. (1995). Moisture relationships of grains. Moisture measurements-ungrounded grain seeds (42nd ed., Vol. S 352.2). Street Joseph, Michigan: ASAE.
Brunschwiler, C., Heine, D., Kappeler, S., Conde-Petit, B., & Nyström, L. (2013). Direct measurement of rice bran lipase activity for inactivation kinetics and storage stability prediction. Journal of Cereal Science, 58(2), 272–277.
Chua, L. Y. W., Chong, C. H., Chua, B. L., & Figiel, A. (2019). Influence of drying methods on the antibacterial, antioxidant and essential oil volatile composition of herbs: a review. Food and Bioprocess Technology, 12(3), 450–476.
Curti, C. A., Curti, R. N., Bonini, N., & Ramón, A. N. (2018). Changes in the fatty acid composition in bitter Lupinus species depend on the debittering process. Food Chemistry, 263, 151–154.
Ding, C., Khir, R., Pan, Z., Zhao, L., Tu, K., El-Mashad, H., & McHugh, T. H. (2015). Improvement in shelf life of rough and brown rice using infrared radiation heating. Food and Bioprocess Technology, 8(5), 1149–1159.
Ding, C., Khir, R., Pan, Z., Wood, D. F., Berrios, J., et al. (2018). Influence of infrared drying on storage characteristics of brown rice. Food Chemistry, 264, 149–156.
Eshghi, N., Asnaashari, M., Haddad Khodaparast, M. H., & Hosseini, F. (2014). Evaluating the potential of natural curcumin for oxidative stability of soybean oil. Natural Product Research, 28(17), 1375–1378.
Frankel, E. (1984). Lipid oxidation: mechanisms, products and biological significance. Journal of the American Oil Chemists' Society, 61(12), 1908–1917.
Irakli, M., Kleisiaris, F., Mygdalia, A., & Katsantonis, D. (2018). Stabilization of rice bran and its effect on bioactive compounds content, antioxidant activity and storage stability during infrared radiation heating. Journal of Cereal Science, 80, 135–142.
Goffman, F. D., & Bergman, C. (2003). Hydrolytic degradation of triacylglycerols and changes in fatty acid composition in rice bran during storage. Cereal Chemistry, 80(4), 459–461.
Jaisut, D., Prachayawarakorn, S., Varanyanond, W., Tungtrakul, P., & Soponronnarit, S. (2009). Accelerated aging of jasmine brown rice by high-temperature fluidization technique. Food Research International, 42(5-6), 674–681.
Kim, S. M., Chung, H. J., & Lim, S. T. (2014). Effect of various heat treatments on rancidity and some bioactive compounds of rice bran. Journal of Cereal Science, 60(1), 243–248.
Krishnamurthy, K., Khurana, H. K., Soojin, J., Irudayaraj, J., & Demirci, A. (2008). Infrared heating in food processing: an overview. Comprehensive Reviews in Food Science and Food Safety, 7(1), 2–13.
Kong, Z., Dong, F., Xu, J., Liu, X., Li, J., Li, Y., Tian, Y., Guo, L., Shan, W., & Zheng, Y. (2012). Degradation of acephate and its metabolite methamidophos in rice during processing and storage. Food Control, 23(1), 149–153.
Li, B., Zhao, L., Chen, H., Sun, D., Deng, B., Li, J., Liu, Y., & Wang, F. (2016). Inactivation of lipase and lipoxygenase of wheat germ with temperature-controlled short wave infrared radiation and its effect on storage stability and quality of wheat germ oil. PLoS One, 11(12), e0167330.
Liu, K., Li, Y., Chen, F., & Yong, F. (2016). Lipid oxidation of brown rice stored at different temperatures. International Journal of Food Science & Technology, 52(1), 188–195.
Mottram, D. S. (1998). Flavour formation in meat and meat products: a review. Food Chemistry, 62(4), 415–424.
Orthoefer, F.T. (2005). Rice bran oil. In: Shahidi, F. (Ed.), Bailey’s Industrial Oil and Fat Products (pp. 65–489). Hoboken, NY: John Wiley & Sons Inc.
Patil, S. S., Kar, A., & Mohapatra, D. (2016). Stabilization of rice bran using microwave: process optimization and storage studies. Food and Bioproducts Processing, 99, 204–211.
Peanparkdee, M., Yamauchi, R., & Iwamoto, S. (2018). Characterization of antioxidants extracted from Thai riceberry bran using ultrasonic-assisted and conventional solvent extraction methods. Food and Bioprocess Technology, 11(4), 713–722.
Pei, Y., Tao, T., Yang, G., Wang, Y., Yan, W., & Ding, C. (2018). Lethal effects and mechanism of infrared radiation on Sitophilus zeamais and Tribolium castaneum in rough rice. Food Control, 88, 149–158.
Pourali, O., Asghari, F. S., & Yoshida, H. (2009). Simultaneous rice bran oil stabilization and extraction using sub-critical water medium. Journal of Food Engineering, 95(3), 510–516.
Qian, J. Y., Gu, Y. P., Jiang, W., & Chen, W. (2014). Inactivating effect of pulsed electric field on lipase in brown rice. Innovative Food Science & Emerging Technologies, 22, 89–94.
Ramezanzadeh, F. M., Rao, R. M., Windhauser, M., Prinyawiwatkul, W., Tulley, R., & Marshall, W. E. (1999). Prevention of hydrolytic rancidity in rice bran during storage. Journal of Agricultural and Food Chemistry, 47(8), 3050–3052.
Sawai, J., Sagara, K., Hashimoto, A., Igarashi, H., & Shimizu, M. (2003). Inactivation characteristics shown by enzymes and bacteria treated with far-infrared radiative heating. International Journal of Food Science & Technology, 38(6), 661–667.
Schmidt, C. G., Gonçalves, L. M., Prietto, L., Hackbart, H. S., & Furlong, E. B. (2014). Antioxidant activity and enzyme inhibition of phenolic acids from fermented rice bran with fungus Rizhopus oryzae. Food Chemistry, 146, 371–377.
Shantha, N. C., & Decker, E. A. (1994). Rapid, sensitive, iron-based spectrophotometric methods for determination of peroxide values of food lipids. Journal of AOAC International, 77(2), 421–424.
Shen, Z., Palmer, M. V., Ting, S. S., & Fairclough, R. J. (1997). Pilot scale extraction and fractionation of rice bran oil using supercritical carbon dioxide. Journal of Agricultural and Food Chemistry, 45(12), 4540–4544.
Silventoinen, P., Rommi, K., Holopainen-Mantila, U., et al. (2019). Biochemical and techno-functional properties of protein- and fibre-rich hybrid ingredients produced by dry fractionation from rice bran. Food and Bioprocess Technology, 9(12), 1487–1499.
Wang, T., Khir, R., Pan, Z., & Yuan, Q. (2017). Simultaneous rough rice drying and rice bran stabilization using infrared radiation heating. LWT- Food Science and Technology, 78, 281–288.
Wanyo, P., Kaewseejan, N., Meeso, N., & Siriamornpun, S. (2015). Marigold flower-powder exhibits significant potential to inhibit lipid oxidation in rice bran tea. Food & function, 6(6), 1808–1817.
Xia, Q., Tao, H., Li, Y., Pan, D., Cao, J., et al. (2020). Characterizing physicochemical, nutritional and quality attributes of wholegrain Oryza sativa L. subjected to high intensity ultrasound-stimulated pre-germination. Food Control, 108, 106827.
Yalcin, S., & Basman, A. (2015). Effects of infrared treatment on urease, trypsin inhibitor and lipoxygenase activities of soybean samples. Food Chemistry, 169, 203–210.
Yılmaz, N., Tuncel, N. B., & Kocabıyık, H. (2014). Infrared stabilization of rice bran and its effects on γ-oryzanol content, tocopherols and fatty acid composition. Journal of the Science of Food and Agriculture, 94(8), 1568–1576.
Zhu, S. M., Lin, S. L., Ramaswamy, H. S., Yu, Y., & Zhang, Q. T. (2017). Enhancement of functional properties of rice bran proteins by high pressure treatment and their correlation with surface hydrophobicity. Food and Bioprocess Technology, 10(2), 317–327.
Acknowledgments
We would like to express our gratitude to Dr. Xiangfei Li for his valuable assistance on the manuscript.
Funding
The authors thank the National Natural Science Foundation of China (NSFC: 31601402) for financial support, and the National Key Research and Development Program of China (Contract No.2017YFD0401403), the Jiangsu Province (China) Agricultural Innovation Project (Contract No. CX (17)1002-05), the Priority Academic Program Development of Jiangsu Higher Education Institutions (PAPD), and the Postgraduate Research & Practice Innovation Program of Jiangsu Province (KYCX18_1409).
Author information
Authors and Affiliations
Corresponding authors
Ethics declarations
Conflict of interest
The authors declare that they have no conflict of interest.
Additional information
Publisher’s Note
Springer Nature remains neutral with regard to jurisdictional claims in published maps and institutional affiliations.
Highlights
•Infrared radiation heating can improve the storage stability of RB;
•Enzyme activity of RB significantly inhibited after infrared radiation heating;
•The composition of the essential FAs can be highly influenced by IR pretreatment;
•Fresh aroma characters for RB were well preserved after infrared treatment.
Rights and permissions
About this article
Cite this article
Yan, W., Liu, Q., Wang, Y. et al. Inhibition of Lipid and Aroma Deterioration in Rice Bran by Infrared Heating. Food Bioprocess Technol 13, 1677–1687 (2020). https://doi.org/10.1007/s11947-020-02503-z
Received:
Accepted:
Published:
Issue Date:
DOI: https://doi.org/10.1007/s11947-020-02503-z