Abstract
Urbanization is one of the most significant human activities in the Anthropocene, with profound impacts on environmental quality. The lack of an understanding about the relationship between urbanization and ecological quality limits the effectiveness of urban planning and ecological policies in alleviating urban ecological problems. Based on the integrated ecological index RSEI (remote sensing ecological index), this study attempts to clarify the spatio-temporal characteristics of ecological quality in an urbanization process through an empirical study in China’s Pearl River Delta (PRD) and explores the relationship between urbanization and ecological quality. Our results show that the ecological development of the PRD in the period of 1986 to 2019 was a phased and polarized process. Two periods are distinguished, based on RSEI dispersion: the period of 1986 to 2003, with slight dispersion, and the period of 2004 to 2019, with higher dispersion. Plain areas show evidence of ecological degradation, whereas a considerable improvement was observed in hilly areas. Industrialization and consummation of legal system were the driving factors behind the phased development of ecological quality, while the differences in landform and land management were the fundamental reasons for the spatial differentiation of ecological quality. The findings of this study provide experience and enlightenment for ecological management and sustainable development strategies in regions seeking rapid growth in their prosperity.
Similar content being viewed by others
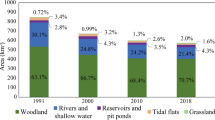
Explore related subjects
Discover the latest articles, news and stories from top researchers in related subjects.Avoid common mistakes on your manuscript.
Introduction
As one of the most significant human-driven changes, urbanization is usually characterized by high-density agglomeration, rapid expansion, and intense pollution (Gong et al. 2019; Hanfi et al. 2019; Chen et al. 2020). Urbanization’s continuous destruction and interference with the ecosystem poses a serious threat to regional ecological security (Watanabe and Ortega 2014; Peng et al. 2016; Wang et al. 2019). How to balance urbanization and ecological protection has been a burning issue in the quest for global sustainable development, especially for those developing regions seeking prosperity (Bryan et al. 2018; Wendling et al. 2018; Xie et al. 2020).
Ecological quality assessment/monitoring has become an important component of regional sustainable management; ecological footprint, ecosystem service supply, and other methods are widely used around the world (Wong et al. 2018; Wood et al. 2018). However, the index selection and process parameters of these methods are usually subjective, to a certain extent, thus bringing uncertainty to the evaluation results. Remote sensing-based methods, on the other hand, are efficient and accurate comparatively. In recent years, many remote sensing-based ecological evaluation indicators (such as normalized difference vegetation index, enhanced vegetation index, and wetness index) have been widely used in the field of regional ecological evaluation (Murray et al. 2018). Among them, a RSEI (remote sensing ecological index) can comprehensively express multiple ecosystem attributes, and RSEI’s process parameters are not subject to the subjective influence of people (Xu et al. 2019; Yuan et al. 2021). As such, using RSEI can provide objective and comprehensive results for regional long-term ecological quality assessment.
The ecological effects of urbanization have recently attracted considerable attention from the fields of landscape ecology and ecosystem services (Fang et al. 2016; Lü et al. 2019; Wang et al. 2019). Land use, population, income, and pollution are considered to be the main driving factors behind the ecological degradation of urban areas (Zhang et al. 2019; Ren et al. 2020; Nathaniel et al. 2021). However, does urbanization always bring about ecological degradation? Does the macro development background, such as economic transformation and land management policies, have an important impact on the ecological changes in urbanized areas? Answering these questions is crucial to discover how to formulate reasonable ecological planning and to implementing effective ecological management.
The Pearl River Delta (PRD) is the first national forest urban agglomeration in China, with a leading position both in urbanization and ecological protection. Revealing the relationship between urbanization and ecological quality in the PRD is of great significance in terms of demonstrating the ecological development of urban agglomerations in developing countries. This study evaluates the ecological quality in the PRD from 1986 to 2019, based on remote sensing. The area’s temporal tipping points and spatial differentiation characteristics are identified, and this study also analyzes the temporal phased and spatial polarized process of ecological quality from multiple perspectives, in combination with the area’s historical development background. This study provides inspiration for the ecological planning and management of regions that are pursuing rapid growth in their prosperity.
Study area and data sources
Study area
The PRD is an urban agglomeration area in southern China with the PRD as its core, surrounded by mountains in the East, West, and North and by the ocean in the South (Fig. 1). The central part of the PRD is a plain with dense river networks, surrounded by hills. It is located in the sub-tropical marine monsoon region; most of the precipitation falls in summer, with frequent typhoons in summer and autumn (Zhang et al. 2016; Xiao et al. 2017). The average annual precipitation in this region is about 1890 mm, and the average annual temperature is about 22° Celsius.
The PRD is adjacent to Hong Kong and Macao, making the area economically interesting. It was therefore one of the regions where the reform and opening-up policy of China was implemented early (Wu 2020). After 40 years of development, the PRD is now one of the most prosperous regions in China, with an enormous population and an international industrial system. Its administrative area covers seven cities, namely, Guangzhou, Shenzhen, Zhuhai, Foshan, Jiangmen, Dongguan, and Zhongshan, as well as the urban area of Huizhou and its three counties of Huiyang, Huidong, and Boluo, and the urban area of Zhaoqing and its two cities of Gaoyao and Sihui. In 2019, the PRD had a GDP of 8.69 × 106 million yuan (about 1.26 × 106 million dollar) and a permanent resident population of 64.47 million, making it one of the most densely populated urban agglomerations in the world. Its large economic aggregate, large population density, and rapid urbanization are typical of developing countries.
Data source
Information on the ecological quality of the PRD, from 1986 to 2019, was extracted from remote sensing images. This study also used elevation data, land use data, meteorological data, and social economic data. As a note, remote sensing images for 1986, 1989, 1992, 1995, 1998, 2003, 2004, 2006, 2008, 2016, and 2019 were selected based on their quality, while images for other years were abandoned, due to their poor quality, which was caused by clouds. Except for special cases, the temporal resolution of other data is consistent with that of remote sensing images. The spatial resolution of remote sensing image and land use data is 30 m.
The 30 m resolution remote sensing images of the PRD were obtained from the National Aeronautics and Space Administration (https://earthexplorer.usgs.gov/). Because of the wet summers and sub-tropical evergreen broad-leaved vegetation in the PRD, images from November and December were chosen, rather than images from the summer season. The 30 m resolution land use data of the PRD were obtained from the Landsat-derived annual land cover product of China (CLCD) (Yang and Huang 2021). Limited by data availability, land use in 1986 and 1989 was represented by the data for 1985 and 1990, respectively, which is acceptable for the early stage of urbanization.
The distribution data on elevation, residential areas, and rivers were obtained from the RESDC (https://www.resdc.cn/). Monthly average precipitation (MP), annual precipitation (AP), annual average temperature (AT), annual minimum temperature (MINT), and annual maximum temperature (MAXT) were measured at various meteorological stations in the PRD, including Fogang (59,087), Guangning (59,271), Gaoyao (59,278), Guangzhou (59,287), Heyuan (59,293), Zengcheng (59,294), Huiyang (59,298), and Taishan (59,478), from 1986 to 2019. These data were obtained from the National Meteorological Science Data Center (http://data.cma.cn/). Data on the permanent resident population and the GDP of cities in the PRD from 1986 to 2019 were derived from the Guangdong Province statistical yearbooks and the PRD Region statistical yearbooks.
Methodology
This study evaluates the urbanization level and ecological quality of the PRD from 1986 to 2019 and analyzes the relationship between urbanization and ecological quality. The main methods include spatial statistical analysis and the RSEI.
The assessment of urbanization level includes landscape patterns and social economy. In terms of landscape patterns, land use change is a common indicator used to reflect the urbanization process. In addition, a chord map can quantitatively and visually describe the conversion of different land types (Laspidou et al. 2020). In this study, ArcGIS 10.2 software is used to calculate the area of main land use types in the PRD from 1985 to 2019 and to carry out the land use transfer matrix. On this basis, the chord diagrams are created by Origin 9.1 software. In terms of social economy, the five indicators of population (POP), gross domestic product (GDP), proportion of primary industry (PIP), secondary industry (SIP), and tertiary industry (TIP) have been chosen to represent the demographic and economic changes in the rapid urbanization process of the PRD.
The RSEI index is used to evaluate the ecological quality. The RSEI is a comprehensive index that integrates multi-ecological information for urban ecosystems. It contains four traditional indices, namely, the normalized vegetation index (NDVI), the wetness index (WET), the dryness index (NDSI), and the land surface temperature (LST), to represent the greenness, wetness, dryness, and heat of land, respectively. These factors are closely related to human wellbeing and can reflect the ecological quality. After normalization, these indices were integrated into the comprehensive RSEI by principal components analysis (PCA), using the following equation (Xu 2013; Ye et al. 2020; Yuan et al. 2021):
where RSEI represents the remote sensing ecological index, with a value range of 0–1. The greater the RSEI value, the better the ecological quality. The parameter PC represents the principal component. This study used the ENVI software (Exelis Visual Solutions Inc. 2014) to conduct principal component analysis on normalized NDVI, WET, NDSI, and LST values to obtain the eigenvalue contribution rate and the principal component eigenvalue of each factor in each principal component. Generally, if the cumulative contribution rate of the principal component eigenvalue exceeds 85%, that component has good accountability. We used the first and second principal components and their eigenvalue contribution rates to calculate the comprehensive score, ensuring a cumulative contribution rate higher than 90%. The NDVI is a characteristic factor of vegetation density distribution and closely related to biomass, leaf area index, and vegetation coverage density, with a value range of -1–1. The greater the NDVI value is, the greater is the vegetation coverage. The WET is a factor related to soil wetness and vegetation and expressed by the humidity component in the tassel hat transformation, with a value range of -1–1; greater values indicate greater soil wetness. The NDSI is synthesized by the built-up index (IBI) and the soil index (SI) and reflects land surface dryness, with a value range of -1–1. The greater the NDSI value is, the greater is the surface dryness, as described in Nichol (2005) and Xu (2008). The LST (in °C) is calculated via the single-channel method based on the TM6 band of Landsat 5 and the Tirs10 band of Landsat 8; the specific algorithm is provided elsewhere (Xu 2015).
For the PRD as a whole, the medium value, mean value, upper and lower quartile, and outliers of RSEI were counted and made into a box-plot. In order to further clarify the area’s spatial differentiation, the medium value, mean value, and upper and lower quartile of RSEI have been counted by the spatial region of plains (DEM ≤ 15 m) and hilly areas (DEM > 15 m) and made into a violin plot.
Results
Spatio-temporal patterns and trend change of land cover
Forest and cropland are the main land use types in the PRD, followed by water and impervious surfaces (Fig. 2a–b). In 1985 and 2019, the proportions of forest were 46.08% and 45.00%; the proportions of cropland were 45.59% and 32.00%; the proportions of water were 5.99% and 7.02%, and the proportions of impervious surfaces were 2.12% and 15.84%, respectively. The rapid urbanization greatly promoted the increase in impervious area; more than 12% of the total land area of the PRD (mainly cropland and forest) was sealed off, mainly around the Pearl River Estuary.
The land cover in the PRD from 1985 to 2019 changed in phases, and significant differences were observed in the change trend among different land types (Fig. 3). From 1985 to 2003, a considerable amount of cropland was sealed off or turned into aquatic areas, with an interconversion between cropland and forest (Fig. 4a). Calculated by the net conversion area, about 147.28 km2 of cropland was sealed off, and 73.79 km2 was turned into water bodies. Approximately 42.20 km2 of forest was transformed into cropland, and 12.73 km2 was sealed off each year. During this period, the average annual net loss of cropland was 180.43 km2, whereas that of forest was 56.12 km2. From 2003 to 2008, the annual net loss of cropland increased sharply, reaching 450.70 km2; cropland was mainly sealed off (202.63 km2), transformed into forest (186.94 km2) or turned into water bodies (48.77 km2) (Fig. 4b). Cropland sealing started to slow down in 2008; the average annual net conversion area in 2008–2019 was 64.56% in the first period and 46.92% in the second period (Fig. 4c). In this timeframe, about 60.55 km2 of water area and 25.29 km2 of forest area were transformed into cropland per year, with an annual cropland net loss of only 6.95 km2. This indicates that the urban growth model in the PRD at the cost of lost cropland has been phased out.
Spatio-temporal variations of the RSEI
The mean and median RSEI values of the PRD as a whole changed very little, but its probability distribution of RSEI changed significantly (Fig. 5a). Two periods could be distinguished, based on RSEI dispersion. Specifically, from 1986 to 2003, there was slight dispersion, with the difference between the upper and lower quartiles not exceeding 0.28 in each year. From 2004 to 2019, dispersion was higher, with the difference between upper and lower quartiles between 0.27 and 0.39 in each year.
Even more specifically, in this study, the PRD has been divided into plains (DEM ≤ 15 m) and hilly areas (DEM > 15 m) for further statistical analysis. The median value of the RSEI in plains decreased from 0.75 to 0.65, and the numerical distribution gradually changed from right-skewed in 1986 to a uniform distribution (Fig. 5b, blue block). This means that the ecological quality in the plain area was declining, and its spatial differentiation was enhanced. Conversely, in the hilly areas, the median RSEI value increased from 0.85 to 0.91, with right-skewed distribution (Fig. 5b, orange block). This shows that the ecological quality in hilly areas improved, and its probability distribution was concentrated to the higher value.
A significant spatial polarization was detected in terms of ecological degradation in plains, while ecological improvement was detected in hilly areas in the PRD, during the study period of 1986 to 2019 (Fig. 6a and b). Areas surrounding the Pearl River Estuary showed significant ecological degradation, with severe (-1.0 ≤ ΔRSEI < -0.37) and moderate (-0.37 ≤ ΔRSEI < -0.13) degradation occurring in 30% of the total area (excluding the water area). Meanwhile, the hilly areas (about 39% of the total area) showed a stable ecological condition, and the condition in the other peripheral areas (about 31% of the total area) improved (Fig. 6c). In terms of specific cities (Fig. 6d), Dongguan was the city with the most severe degradation; 22% of the area was severely degraded, and 30% was moderately degraded. The most significant ecological improvement was found in Zhaoqing; an area accounting for 16% was dramatically improved, and an area of 43% was moderately improved.
The RSEI results were reclassified from high to low, according to natural breakpoints, corresponding to five grades of ecological quality: excellent, good, moderate, fair, and poor. Areas that degraded to poor quality areas between 1986 and 2003 formerly showed excellent, good, moderate, and fair quality, whereas areas that transformed between 2003 and 2019 mainly showed moderate quality, followed by good and fair quality (Tables 1 and 2). In contrast, the proportion of areas with good quality that degraded to areas with fair quality during the years from 2003 to 2019 was 2.25 times that during the 1986 to 2003 period.
Correlation between urbanization and the RSEI
Correlation between population, economy, and the RSEI
The PRD experienced rapid urbanization from 1986 through 2019, with a population growth of 2.10 times and a GDP growth of 220.09 times. The proportion of secondary and tertiary industries increased from 40.52% and 32.95% to 45.40% and 50.50%, respectively. We examined the effects of population (POP), gross domestic product (GDP), proportion of primary industry (PIP), second industry (SIP), and tertiary industry (TIP) on the RSEI through bivariate correlation analysis (Table 3).
Overall, significant correlations were observed between GDP, GDP square, and RSEI. Taking specific cities as units, the elements significantly related to RSEI were more specific. In cities with rapid socio-economic development, such as Dongguan, Guangzhou, Shenzhen, Zhongshan, and Zhuhai, the RSEI showed a significant negative correlation with population and GDP. In Foshan and Huizhou, the RSEI was significantly negatively correlated with GDP or GDP square, whereas in Jiangmen and Zhaoqing, the correlation between the RSEI, POP, and GDP was not significant. In cities where the RSEI was significantly related to the economy, the RSEI of Dongguan and Zhuhai showed a significant positive correlation with their PIP and a significant negative correlation with TIP. The RSEI of Shenzhen and Zhongshan was significantly negatively correlated with TIP. Further, the RSEI of Guangzhou was significantly positively correlated with SIP, whereas in Zhaoqing, the RSEI showed a significant positive correlation with TIP.
Correlation between land cover and RSEI
Considering the natural conditions, land use change, and socio-economic development, an arc-shaped transect with Zhuhai as the center of the circle and a radius of 90 km could be set up. The RSEI values for 1986 and 2019 were extracted along this transect to determine the impacts of land use changes on the ecological development, from the perspective of profile comparison (Fig. 7). The eastern and middle sections of the transect (located in Shenzhen, Dongguan, Guangzhou, and Foshan) were the main areas of rapid urbanization in the PRD, whereas urbanization was slow in the western section (mainly in Jiangmen). According to the comparison of the RSEI values between 1986 and 2019, along the transect, the ecological quality of most areas (except mountains) in the urbanized section decreased significantly, with interval average values decreasing from 0.78 to 0.56. In contrast, the ecological quality of the non-urbanized section remained stable, and this section’s interval average value decreased from 0.82 to 0.80.
The mean RSEI values for different land types changed over time, and the differences among different land types were significant (Fig. 8). Specifically, forest land showed the highest ecological quality, with an average RSEI of 0.90, followed by shrubs (0.75), cropland (0.70), impervious surfaces (0.64), and grassland and wasteland (0.40). Regarding the change trend, the RSEI of forest was always relatively stable, whereas cropland, shrub, grassland, wasteland, and impervious surface lands showed phased trend differences, with 2003 as the turning point. This indicates a growth or stable trend from 1985 to 2003, and a downward trend, in varying degrees, from 2003 to 2019. In the previous period, the average RSEI values for cropland, shrub, grassland, barren land, and impervious surface land were 0.71, 0.79, 0.53, 0.53, and 0.68, respectively. In the later period, the average RSEI values of cropland, shrub, grassland, wasteland, and impervious surface land were 0.70, 0.71, 0.43, 0.44, and 0.60, respectively. During the study period, the transformation from cropland to impervious surface decreased the ecological quality of 11.42% of the land area in the PRD by 9.38%. The transformation from forest to impervious surface decreased the ecological quality of 1.33% of the land area by 29.40%, and the transformation from forest to cropland decreased the ecological quality of 4.44% of the land area by 22.10%. In contrast, the transformation from cropland to forest increased the ecological quality of 2.59% of the land area by 28.37%.
The correlation between land transformation and the RSEI varied in different cities (Table 4). There was a significant correlation between land transformation and the RSEI in Dongguan, Shenzhen, Zhongshan, and Zhuhai. The decline of the RSEI in Dongguan and Shenzhen was significantly correlated with the transformation of cropland to impervious surface land, whereas the decline of the RSEI in Zhongshan and Zhuhai was significantly correlated with the transformation of cropland and forest to impervious surface land.
Discussion
In this section, the underlying mechanisms of the temporal phased and spatial polarized development of ecological quality in the PRD are analyzed from multiple perspectives, based on the historical background. The uncertainty pertaining to the data and methods is also discussed.
Underlying mechanisms for the phased development of ecological quality
Combined with the development background of the PRD, this study believes that industrial transformation and the promulgation of local environmental protection laws are the main driving factors behind the phased change of ecological quality.
Industrialization was an important historical process in the PRD during 1986–2019 and had an important impact on ecological quality. Before the 1980s, the social economy of the PRD mainly depended on the primary industry. With the natural productivity being protected, the ecological quality was correspondingly maintained at a high level. In 1986, the PRD was listed as a coastal economic open zone. Subsequently, a large amount of overseas investment, advanced production technology,and management methods were introduced (Liang et al. 2021). These accelerated the PRD’s industrialization process. In particular, a large number of family workshops emerged in the vast rural areas, to engage in commodity production. From 1985 to 2003, a large amount of cropland was taken and used for the construction of factories and urban infrastructure, causing the ecological quality of the surrounding region to decline. At the same time, due to the decentralized distribution and lack of supervision of family workshops, the industrial wastes of those workshops were not effectively and innocuously treated before discharge, which further aggravated the harm caused by industrialization to the ecological environment (Zhang et al. 2017). After 2003, as costs of production factors continued to rise, some labor-intensive manufacturing industries began to move out. In 2008, under the impact of the global financial storm, the industrial structure of the PRD began to transform into technology-intensive industries and financial and service industries. These environment-friendly industrial transformations have reduced the negative impact of industrial activities, to a certain extent (Shen et al. 2019). Also, the construction of industrial parks, with continuous scale and standardization, has also caused industrial wastes to be more centralized and harmless, thereby also reducing the negative ecological impact of industrial wastes (Wu et al. 2020).
The consummation of legal system is an important reason for the ecological recovery of parts of the PRD. From 1986 to 1995, the PRD was in the early stage of China’s “reform and opening up.” People were eager to pursue social and economic prosperity, but their awareness of ecological protection was limited. In 1996, the managers of this region realized the importance of environmental protection and began to seek sustainable development. By 2004, the government had issued the Environmental Protection Regulations of Guangdong Province, and an environmental protection plan was formulated. Environmental pollution and ecological damage have been curbed through the joint efforts of many departments, which carry out special governance actions. Since then, resource conservation and environmental protection have been important elements of the PRD’s social and economic development plans (Liang et al. 2019). In 2014, the government further revised and improved the local environmental protection laws and issued the Supporting Environmental Protection Policy on the Main Functional Area Planning of Guangdong Province. The aim was to better ensure the implementation of the environmental protection plan. The implementation of these environmental protection laws and plans plays an important role in promoting the restoration and maintenance of ecological quality.
Underlying mechanisms for the spatial polarization of ecological quality
Landform and land management policies have an important impact on the spatial polarization of ecological quality in the PRD.
Landform determines the spatial differences of social and economic activity intensity and further leads to the spatial differences of ecological quality changes. The rapid growth of both the population and the economy in the PRD has generated a huge demand for construction land, resulting in the loss of ecological land. As the plains area has better social, economic, and land use development conditions than the hilly area, the ecological land loss was concentrated in the PRD’s central plain. The remarkable differences in social economic activities have driven the constant ecological degradation in the plains and ecological maintenance in the hilly areas. These inferences are in agreement with Li et al. (2020) and Zhou et al. (2020).
The differences in land management policies drove the ecological quality in the plains and hilly areas to move in opposite directions after 2002. The “Grain for Green” (GFG) program and the construction of reserves are the main reasons for the maintenance and optimization of the ecological quality in hilly areas. Since 2002, the large-scale GFG revegetation program has been in operation; this is a major ecological project with the largest investment and the strongest policy in China (Li et al. 2019). Under the influence of the GFG program, a large amount of arable land that is suitable for forestry in the hilly areas has been restored to forest land (Zhao et al. 2019; Zheng et al. 2019). The ecological quality of hilly areas has improved with the increase of forest area. With more forest parks and nature reserves being established, the ecosystem in hilly areas has been more scientifically and systematically protected. Under the influence of these land management policies, the ecological quality in hilly areas has gradually transformed to a higher value.
Since 2003, the spatial differentiation of ecological quality in the plains has gradually intensified, having been influenced by the cropland protection policy and urban spatial planning, to a certain extent. Against the background of food security, Guangdong Province began to strictly restrict the conversion of cropland into construction land; in 2002, basic cropland began to be protected at different levels. Under the strict cropland protection policy, the ecological quality of rural areas in the central plain gradually tended to stabilize. After 2003, the scope limitation of urban expansion and the zoning management of main functions greatly promoted the ecological land protection of cities and their suburbs (Phalan 2018; Liu et al. 2019). From 2008, the implementation of the land policy of intensified utilization improved land use efficiency through the transformation of old towns, factories, and villages. Under the guidance of these policies, the land in the plains area is either protected as ecological land (such as cropland and city parks) or developed as highly-efficient construction land. The spatial differentiation of ecological quality is also gradually intensifying.
Limitations and future prospects
Although the methods and models in this study have been widely used, there are still uncertainties in the assessment of ecological quality. First, from the perspective of data, because remote sensing images in some years were blocked by clouds and could not be used, the time interval of data in this study is not fixed. This shortcoming may cause some uncertainties in the recognition accuracy of ecological turning points. Since November and December are the harvest seasons for some crops (such as rice) in the PRD, using remote sensing images from this period as the data basis for calculating the RSEI may, to a certain extent, lead to the underestimation of the ecological quality of cropland. In addition, the average RSEI value varies slightly in different years, having not only been affected by the polarization of ecological quality in the plains and hilly areas, but also having been affected by the uncertainty of data sources. Second, from the perspective of methodology, the RSEI index represents the ecological quality based on NDVI, WET, NDSI, and LST. Of these factors, WET and NDSI are sensitive to precipitation (Xu 2013, 2015), which may lead to some uncertainty in the results. In addition, this study mainly considers the biophysical properties of the ecosystem, but lacks adequate consideration of the ecosystem process, which may cause uncertainty in the results (Desouhant et al. 2019; Figueiredo et al. 2019). Therefore, in future research, the combination of remote sensing and ecosystem process models (for example, Biome BGC) should receive more attention (Xu et al. 2016). These efforts will provide insights into ecological protection and spatial planning in areas experiencing rapid urbanizing.
Conclusions
This study used the RSEI model and multivariate data to analyze the temporal and spatial distribution pattern of the ecological quality of China’s PRD urban agglomeration, from 1986 to 2019, considering the driving factors of temporal and spatial changes of ecological quality in urbanization areas in a long time series. Based on the results, the evolution of ecological quality in the PRD showed a phased trend transformation in time and a bipolar differentiation in space. Significant negative correlations existed between population, economy and land urbanization, and ecological quality. Industrialization, consummation of legal system, and land management have played important roles in eco-environmental protection and the control of urban growth in the PRD. The results provide important insights for healthy urbanization, urban landscape planning, and ecological management in rapidly-urbanizing areas.
Data availability
All data generated or analyzed during this study are included in this article.
References
Bryan BA, Gao L, Ye YQ, Sun XF, Connor JD, Crossman ND, Stafford-Smith M, Wu JG, He CY, Yu DY, Liu ZF, Li A, Huang QX, Ren H, Deng XZ, Zheng H, Niu JM, Han GD, Hou XY (2018) China’s response to a national land-system sustainability emergency. Nature 559:193–204. https://doi.org/10.1038/s41586-018-0280-2
Chen THK, Qiu C, Schmitt M, Zhu XX, Prishchepov AV (2020) Mapping horizontal and vertical urban densification in Denmark with Landsat time-series from 1985 to 2018: a semantic segmentation solution. Remote Sens Environ 251:112096. https://doi.org/10.1016/j.rse.2020.112096
Desouhant E, Gomes E, Mondy N, Amat I (2019) Mechanistic, ecological, and evolutionary consequences of artificial light at night for insects: review and prospective. Entomol Exp Appl 167(1):37–58. https://doi.org/10.1111/eea.12754
Exelis Visual Solutions Inc (2014) ENVI Manual. http://www.exelisvis.com/ProductsServices/ENVIProducts.aspx. Accessed 25 Nov 2014
Fang CL, Zhou CH, Gu CL, Chen LD, Li SC (2016) Theoretical analysis of interactive coupled effects between urbanization and eco-environment in mega-urban agglomerations. Acta Geographica Sinica 71:531–550. https://doi.org/10.11821/dlxb201604001
Figueiredo L, Krauss J, Steffan-Dewenter I, Cabral JS (2019) Understanding extinction debts: spatio-temporal scales, mechanisms and a roadmap for future research. Ecography 42:1973–1990. https://doi.org/10.1111/ecog.04740
Gong P, Li X, Zhang W (2019) 40-year (1978–2017) Human settlement changes in China reflected by impervious surfaces from satellite remote sensing. Sci Bull 64(11):756–763. https://doi.org/10.1016/j.scib.2019.04.024
Hanfi MY, Mostafa M, Zhukovsky MV (2019) Heavy metal contamination in urban surface sediments: sources, distribution, contamination control, and remediation. Environ Monit Assess 192:32. https://doi.org/10.1007/s10661-019-7947-5
Laspidou CS, Mellios NK, Spyropoulou AE, Kofinas DT, Papadopoulou MP (2020) Systems thinking on the resource nexus: modeling and visualisation tools to identify critical interlinkages for resilient and sustainable societies and institutions. Sci Total Environ 717:137264. https://doi.org/10.1016/j.scitotenv.2020.137264
Li G, Sun SB, Han JC, Yan JW, Liu WB, Wei Y, Lu N, Sun YY (2019) Impacts of Chinese grain for green program and climate change on vegetation in the loess plateau during 1982–2015. Sci Total Environ 660:177–187. https://doi.org/10.1016/j.scitotenv.2019.01.028
Li M, Hu MM, Xia BC (2020) Spatial differentiation and aggregation characteristics of ecological security of urban agglomeration in the Pearl River Delta. Acta Scientiarum Naturalium Universitatis Sunyatseni 59:96–104. https://doi.org/10.13471/j.cnki.acta.snus.2020.01.012
Liang LW, Chen MX, Luo XY, Xian Y (2021) Changes pattern in the population and economic gravity centers since the reform and opening up in China: the widening gaps between the South and North. J Clean Prod 310:127379. https://doi.org/10.1016/j.jclepro.2021.127379
Liang YT, Zhou ZK, Li X (2019) Dynamic of regional planning and sustainable development in the Pearl River Delta, China. Sustainability 11(21):6074. https://doi.org/10.3390/su11216074
Liu W, Zhan JY, Zhao F, Yan HM, Zhang F, Wei XQ (2019) Impacts of urbanization-induced land-use changes on ecosystem services: a case study of the Pearl River Delta Metropolitan Region, China. Ecol Ind 98:228–238. https://doi.org/10.1016/j.ecolind.2018.10.054
Lü LT, Zhang J, Peng QZ, Ren FP, Jiang Y (2019) Landscape pattern analysis and prediction in the Dongjiang River Basin. Acta Ecol Sin 39:6850–6859. https://doi.org/10.5846/stxb201808281844
Murray NJ, Keith DA, Bland LM, Ferrari R, Lyons MB, Lucas R, Pettorelli N, Nicholson E (2018) The role of satellite remote sensing in structured ecosystem risk assessments. Sci Total Environ 619–620:249–257. https://doi.org/10.1016/j.scitotenv.2017.11.034
Nathaniel SP, Nwulu N, Bekun F (2021) Natural resource, globalization, urbanization, human capital, and environmental degradation in Latin American and Caribbean Countries. Environ Sci Pollut Res 28:6207–6221. https://doi.org/10.1007/s11356-020-10850-9
Nichol J (2005) Remote sensing of urban heat islands by day and night. Photogrammetric Eng Remote Sens 71:613–621. https://doi.org/10.14358/PERS.71.5.613
Peng J, Xie P, Liu YX, Ma J (2016) Urban thermal environment dynamics and associated landscape pattern factors: a case study in the Beijing Metropolitan Region. Remote Sens Environ 173:145–155. https://doi.org/10.1016/j.rse.2015.11.027
Phalan B (2018) What have we learned from the land sparing-sharing model? Sustainability 10(6):1760. https://doi.org/10.3390/su10061760
Ren YJ, Lü YH, Fu BJ, Comber A, Li T, Hu J (2020) Driving factors of land change in China’s Loess Plateau: quantification using geographically weighted regression and management implications. Remote Sensing 12:453. https://doi.org/10.3390/rs12030453
Shen J, Wang SJ, Liu W, Chu JX (2019) Does migration of pollution-intensive industries impact environmental efficiency? Evidence supporting “Pollution Haven Hypothesis.” J Environ Manage 242:142–152. https://doi.org/10.1016/j.jenvman.2019.04.072
Watanabe MDB, Ortega E (2014) Dynamic emergy accounting of water and carbon ecosystem services: a model to simulate the impacts of land-use change. Ecol Model 271:113–131. https://doi.org/10.1016/j.ecolmodel.2013.03.006
Wang ZB, Liang LW, Sun Z, Wang XM (2019) Spatiotemporal differentiation and the factors influencing urbanization and ecological environment synergistic effects within the Beijing-Tianjin-Hebei Urban Agglomeration. J Environ Manage 243:227–239. https://doi.org/10.1016/j.jenvman.2019.04.088
Wendling LA, Huovila A, Castell-Rudenhausen MZ, Hukkalainen M, Airaksinen M (2018) Benchmarking nature-based solution and smart city assessment schemes against the sustainable development goal indicator framework. Front Environ Sci 6:69. https://doi.org/10.3389/fenvs.2018.00069
Wong CP, Jiang B, Kinzig AP, Ouyang Z (2018) Quantifying multiple ecosystem services for adaptive management of green infrastructure. Ecosphere 9(11):02495. https://doi.org/10.1002/ecs2.2495
Wood SLR, Jones SK, Johnson JA, Brauman KA, Chaplin-Kramer R, Fremier A, Girvetz E, Gordon LJ, Kappel CV, Mandle L, Mulligan M, O’Farrell P, Smith WK, Willemen L, Zhang W, DeClerck FA (2018) Distilling the role of ecosystem services in the sustainable development goals. Ecosyst Serv 29:70–82. https://doi.org/10.1016/j.ecoser.2017.10.010
Wu JL, Wang X, Wang H, Gong DC, Yang J, Jiang B, Zhang CL, Deng XJ, Zhou L, Wang BG (2020) Rapidly alleviating particulate matter pollution while maintaining high-speed economic development in the “world’s factory.” J Clean Prod 266:121844. https://doi.org/10.1016/j.jclepro.2020.121844
Wu L (2020) Forty years of reform and opening up: course and experience. Contemporary China Press, Beijing
Xiao R, Li Z, Wu Z, Wu C, Yi W (2017) Urbanization process and eco-environmental effects in the Pearl River Delta Region. Longmen Publishing House of Science Press, Beijing
Xie H, Zhang Y, Zeng X, He Y (2020) Sustainable land use and management research: a scientometric review. Landscape Ecol 35(2):2381–2411. https://doi.org/10.1007/s10980-020-01002-y
Xu HQ (2008) A new index for delineating built-up land features in satellite imagery. Int J Remote Sens 29:4269–4276. https://doi.org/10.1080/01431160802039957
Xu HQ (2013) A remote sensing urban ecological index and its application. Acta Ecol Sin 33:7853–7862. https://doi.org/10.5846/stxb201208301223
Xu HQ (2015) Retrieval of the reflectance and land surface temperature of the newly-launched Landsat 8 satellite. Chin J Geophys 58:741–747. https://doi.org/10.6038/cjg20150304
Xu HQ, Wang YF, Guan HD, Shi TT, Hu XS (2019) Remote sensing detecting ecological changes with a remote sensing based ecological index (RSEI) produced time series and change vector analysis. Remote Sensing 11:2345. https://doi.org/10.3390/rs11202345
Xu XB, Yang GS, Tan Y, Zhuang QL, Li HP, Wan RR, Su WZ, Zhang J (2016) Ecological risk assessment of ecosystem services in the Taihu Lake Basin of China from 1985 to 2020. Sci Total Environ 554–555:7–16. https://doi.org/10.1016/j.scitotenv.2016.02.120
Yang J, Huang X (2021) 30 m annual land cover and its dynamics in China from 1990 to 2019 (1.0.0). Zenodo. https://doi.org/10.5281/zenodo.4417810
Ye JP, Liu SY, Sheng F, Liu Z, Yang M, Li J (2020) Landscape pattern evolution and ecological environment effect of Xunwu watershed. Acta Ecol Sin 40:4737–4748. https://doi.org/10.5846/stxb201909201966
Yuan BD, Fu LN, Zou YA, Zhang SQ, Chen XS, Li F, Deng ZM, Xie YH (2021) Spatiotemporal change detection of ecological quality and the associated affecting factors in Dongting Lake Basin, based on RSEI. J Clean Prod 302:126995. https://doi.org/10.1016/j.jclepro.2021.126995
Zhang GL, Bai JH, Xiao R, Zhao QQ, Jia J, Cui BS, Liu XH (2017) Heavy metal fractions and ecological risk assessment in sediments from urban, rural and reclamation-affected rivers of the Pearl River Estuary, China. Chemosphere 184:278–288. https://doi.org/10.1016/j.chemosphere.2017.05.155
Zhang Y, Long H, Tu S, Ge D, Ma L, Wang L (2019) Spatial identification of land use functions and their tradeoffs/synergies in China: implications for sustainable land management. Ecol Ind 107:105550. https://doi.org/10.1016/j.ecolind.2019.105550
Zhang ZS, Chen CL, Liao WQ (2016) Geography of Guangdong. Beijing Normal University Press, Beijing
Zhao AZ, Zhang AB, Liu JH, Feng LL, Zhao YL (2019) Assessing the effects of drought and “grain for green” program on vegetation dynamics in China’s Loess Plateau from 2000 to 2014. CATENA 175:446–455. https://doi.org/10.1016/j.catena.2019.01.013
Zheng K, Wei JZ, Pei JY, Cheng H, Zhang XL, Huang FQ, Li FM, Ye JS (2019) Impacts of climate change and human activities on grassland vegetation variation in the Chinese Loess Plateau. Sci Total Environ 660:236–244. https://doi.org/10.1016/j.scitotenv.2019.01.022
Zhou R, Lin M, Wu Z, Liu H, Zhong L (2020) Construction of ecological security in Guangdong-Hong Kong-Macao Greater Bay Area from the perspective of importance of ecosystem services. Ecological Economy 36:189–196
Funding
This study was funded by the National Natural Science Foundation of China (No. 41771096 and No. 42201098) and Science and Technology Program of Guangzhou, China (No. 202102080254).
Author information
Authors and Affiliations
Contributions
Ting Li and Yuxiang Dong made substantial contributions to the study design and led the writing of the manuscript. Both authors contributed critically to the drafts, and both approved the final manuscript.
Corresponding author
Ethics declarations
Ethics approval and consent to participate
Not applicable.
Consent for publication
The manuscript is approved by all authors for publication.
Competing interests
The authors declare no competing interests.
Additional information
Responsible Editor: Philippe Garrigues
Publisher's note
Springer Nature remains neutral with regard to jurisdictional claims in published maps and institutional affiliations.
Rights and permissions
Springer Nature or its licensor (e.g. a society or other partner) holds exclusive rights to this article under a publishing agreement with the author(s) or other rightsholder(s); author self-archiving of the accepted manuscript version of this article is solely governed by the terms of such publishing agreement and applicable law.
About this article
Cite this article
Li, T., Dong, Y. Phased and polarized development of ecological quality in the rapidly-urbanized Pearl River Delta, China. Environ Sci Pollut Res 30, 36176–36189 (2023). https://doi.org/10.1007/s11356-022-24852-2
Received:
Accepted:
Published:
Issue Date:
DOI: https://doi.org/10.1007/s11356-022-24852-2