Abstract
Mature granules and flocs from aerobic sludge were collected from the wastewater treatment plant (WWTP) treating both municipal and industrial effluents in Haining city China. This plant has been operating under conditions favoring aerobic granular sludge formation, for over 3 years, suggesting that the granules, which stably retained under changing effluent conditions, may contain unique microbial populations. Microbial analysis indicated that the granular sludge was primarily composed of Planctomycetes, Proteobacteria and Bacteroidetes from the bacterial phyla. Interestingly, microbial communities were also observed to be stratified between the structural features of the sludge. For example, Euryarchaeota was found to make up the majority of the archaea found in the granules while Methanosaeta was dominant in the flocs. Additionally, granules were found to contain, 34 phyla and 222 genera of bacteria, 4 phyla and 13 genera of fungi, and 2 phyla and 17 genera of archaea. While flocs contained, 32 phyla and 203 genera of bacteria, 6 phyla and 26 genera of fungi, and 2 phyla and 12 genera of archaea. This biodiversity signifying a preservation of bacterial and archaeal population in granules, and fungal populations in flocs may result from the sedimentary characteristics of the granules. This suggests microbes uniquely associated in the granules are playing a key role in structure formation and stability of the granular ecosystem, which is maintained by the longer sludge retention time.
Similar content being viewed by others
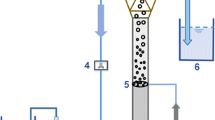
Explore related subjects
Discover the latest articles, news and stories from top researchers in related subjects.Avoid common mistakes on your manuscript.
Introduction
Over the last decade, aerobic granular sludge (AGS) has become a hot research topic because of its excellent settleability, compact structure, high biomass and ability to withstand toxicity in comparison to conventional activated sludge (AS) (Adav et al. 2008; Khan et al. 2013). AGS was defined as “self-immobilized microbial aggregates” by the International Water Association (IWA) in 2005. However, microbial populations of matured AGS have not been analyzed (Lee et al. 2010), possible due to a lack of modern analytical approaches. Initial studies of AGS have been conducted in laboratory-scale reactors (de Kreuk 2006; Ni et al. 2009; Liu et al. 2011; Rocktaschel et al. 2015) and very little information is known about AGS collected from full-scale operational WWTP (Giesen et al. 2013; Li et al. 2014).
Molecular biological techniques, such as polymerase chain reaction (Guo et al. 2011), denaturing gradient gel electrophoresis (Liu et al. 2011), fluorescence in situ hybridization (Jungles et al. 2011), terminal restriction fragment length polymorphism (Liu et al. 2008) and high-throughput sequencing (Huang et al. 2015) have been applied to analyze AGS microbial communities. 454 pyrosequencing is a new technique based on the high-throughput sequencing approach, which provides robust microbial population sequencing data. 454 pyrosequencing has been shown its utility in the analysis of AS (Zhang et al. 2012), drinking water (Huang et al. 2014) and soil (Roesch et al. 2007) microbial communities.
AGS was cultivated in the sequential batch reactor (SBR) of a full-scale WWTP simultaneously treating municipal and industrial wastewater. Here we characterize the bacterial, fungal and archaeal communities associated with mature and stable AGS using 454 pyrosequencing.
Materials and methods
WWTP operation
The WWTP located in Haining City, China, has a treatment capacity of 50,000 m3/day. This treatment facility was divided into four separate tanks for alternating operation. The height/depth (H/D) ratio of each tank was 0.09, and each tank had a reactor volume of 12,540 m3 (L 55 m × W 38 m × D 6 m). The volumetric exchange ratio was maintained between 50 and 70%. This recently constructed WWTP became operational at the end of 2010 (samples were collected after 3 years). After 180 days of SBR operation, the full-scale WWTP attained stable operational parameters as follows: filling (40 min), aeration (240 min), settling (50 min) and effluent discharge (30 min).
The influent wastewater was composed of 30% municipal and 70% industrial wastewater, primarily originating from printing, dyeing, chemical, textile and beverage industries.
Sludge sampling
Uniformly mixed sludge samples were obtained after 1.5 h of aeration. The sludge was collected in a 1 L volumetric cylinder and allowed to settle for 5 min. Flocs suspended in the upper phase of the cylinder were collected and transferred into a separate container. The settled granules were gently washed twice with fresh water and transferred to another container. Collected granules and flocs were stored immediately at −20 °C for further microbial analysis.
DNA extraction and 454 pyrosequencing
Collected flocs and granules samples were aliquoted into three equal portions. The total DNA extracted from each sample portion was subjected to 454 Pyrosequencing in triplicate. Total DNA was extracted using a FastDNA SPIN Kit (MP Biomedicals, USA) as per manufacturer’s instructions. PCR amplicon libraries were prepared using universal primers and three sets of primers were used to distinguish microbial phyla. The first primer set amplified the V1–V3 (hyper-variable) regions of the bacterial 16S rRNA gene (forward 5′-AGAGTTTGATCCTGGCTCAG-3′, reverse 5′-TTACCGCGGCTGCTGGCAC-3′). The second primer set amplified the V3–V6 (hyper-variable) regions of the archaeal 16S rRNA gene (forward 5′-ACGGGGYGCAGCAGGCGCGA-3′, reverse 5′-GTGCTCCCCCGCCAATTCCT-3′). The third primer set amplified the ITS (internal transcribed spacer) region of fungal 18S rRNA (forward 5′-GGAAGTAAAAGTCGTAACAAGG-3′, reverse 5′-AGTCCTCCGCTTATTGATATGC-3′). 454 Pyrosequencing was conducted using a GS-FLX+ system at Personal Biotechnology Co., Ltd (Shanghai, China). The obtained sequence data was processed with Quantitative Insights into Microbial Ecology (Qiime) pipeline and Mothur software. Biodiversity and species richness was analyzed using high-quality reads (HQR). HQR were obtained by removing the sequences based on the following criteria; lengths shorter than 200 nt, greater than 1000 nt, mean quality <25, ambiguous bases or homopolymer length >6, and a 5′ primer mismatch >1. The majority of observed HQR fell in the range of 495–530 nt. The sequences were clustered and assigned to Operational Taxonomic Units (OTUs) using the Qiime implementation based on >97% similarity. The indexes of biodiversity and species richness, such as abundance-based coverage estimator (ACE), Chao1, Simpson, Shannon and coverage were calculated in Mothur software.
Analytical methods
The sludge volume index (SVI), mixed liquid suspended solids (MLSS), chemical oxygen demand (CODCr), biological oxygen demand (BOD), total phosphorus (TP) and ammonia (NH4 +–N) concentrations were measured regularly using standard methods (APHA 2006). The morphology of aerobic sludge granules and flocs were observed using an optical microscope (CX31, Olympus) and imaged with a Canon digital camera (Canon, 30d, Japan).
Results
Characteristics of AGS
Over 3 years of operation, aerobic granules were observed to be the primary component of the sludge. The MLSS, SVI30 and granules diameter were observed to be within the ranges of 2.7–4 g/L, 40–56 mL/g and 0.5–0.6 mm, respectively. This is similar to the values previously reported at this WWTP: SVI30 47.1 mL/g, diameter 0.5 mm, and settling velocity of 42 m/h (Li et al. 2014). Granules were observed to have a well-defined elliptical shape (Fig. 1a) compared to the flocs with irregular and loose structure (Fig. 1b). The influent COD, BOD5, NH4 +–N and TP values were 200–600, 50–105, 28–40 and 2–4 mg/L, respectively. In the treated effluent water the COD, NH4 +–N, BOD5 and TP values were observed to be 30–90, 0–1.68, 15–20 and 1–2 mg/L, respectively.
Also, the WWTP had a good performance regarding COD and NH4 +–N removal rates, which were maintained at about 85 and 96%. The average BOD/COD value of influent wastewater was 0.23, which is indicative of the biorefractory nature of wastewater. It is worth noting that raw wastewater contained a large amount of salt in the WWTP (Li et al. 2014).
Analysis of microbial populations in granules and flocs by 454 pyrosequencing
Bacteria
Although the flocs were found to have more HQR (Table 1), Chao1 and ACE values observed to be higher in granules (Table 2). The Chao1 and ACE values suggest that bacterial richness of granules is higher than flocs, but Shannon and Simpson indexes did not reveal significant differences in bacterial diversity between the granules and flocs. This inconsistency may have resulted from the inability of Chao1 and ACE values to estimate the bacterial diversity of small samples (Hughes et al. 2001). The OTUs abundance curve suggested that granules had more bacterial species richness and evenness as displayed by the broader OTU rank (Fig. 2a) compared to the flocs. Granules contained 2241 OTUs whereas the flocs were found to contain 2009 OTUs. Remarkably the granules and flocs only shared 817 OTUs (Fig. 3a), which represents 40% of the floc OTUs and 36% of the granule OTUs.
In granules (Fig. 4a1), 34 phylum and 222 genera were detected. The dominant phyla were Planctomycetes (30.3%), Proteobacteria (28.2%), Bacteroidetes (22.2%), Chloroflexi (9.4%), Firmicutes (2.8%) and Chlorobi (2.5%). In the case of flocs bacterial populations 32 phyla and 203 genera were observed. This indicated that flocs contained slightly fewer genera than the granules. Among flocs, Proteobacteria were found to be the dominant phylum representing 51.9% (Fig. 4a1). This was consistent with previous studies of bacterial populations in sewage (McLellan et al. 2010) and AS (Zhang et al. 2012), where proteobacteria were also found to be the dominant phylum. The other dominant flocs phyla were found to be Bacteroidetes (15.8%), Planctomycetes (12.2%), Chloroflexi (7.4%), Firmicutes (5.2%), Chlorobi (2.1%) and TM7 (1.0%). Granules and flocs were found to share 130 genera (Fig. 4a2). Flavobacterium, Acinetobacter and Pseudomonas were observed to be the predominant genera in both granules and flocs, but their abundances were different between flocs and granules. The well-known ammonia oxidizing bacteria, Nitrosomonas and Nitrospira were found in both granules and flocs.
Microbial communities in granules and flocs at phylum and genus levels. a Bacterial composition in granules and flocs at phylum (a1) and genus (a2) levels, b fungal composition in granules and flocs at phylum (b1) and genus (b2) levels, c archaeal composition in granules and flocs at phylum (c1) and genus (c2) levels
Fungi
In the case of fungal populations, flocs not only have higher HQR (Table 1), but the fungal richness and diversity are superior to the granules (Table 2; Fig. 2b). Flocs contained 364 OTUs whereas the granules were found to contain 308 OTUs. The granules and flocs were found to share 237 OTUs (Fig. 3b), which represents 65% of the floc OTUs and 77% of the granule OTUs. These results suggest that the flocs may be better at supporting fungal growth, which could be the reason for less fungal diversity in the granules.
The fungal populations of the samples indicated that the granules had 4 phyla and 13 genera, whereas the flocs contained 6 phyla and 26 genera (Fig. 4b). Most of the fungal sequences obtained from the granules and flocs were unidentified. The large number of unidentified fungal OTUs stem from less exploration of the fungal domain as has been reported previously (Weber et al. 2009a, b). Ascomycota, Basidiomycota and Glomeromycota, phyla were found in both granules and flocs. Ninety five percent of the fungal genera were unidentified. However, the most abundant identified genus in both flocs and granules was Scopulariopsis (Fig. 4b2).
Archaea
The archaeal HQR were observed to be higher in granules than flocs (Table 1), Chao1 and ACE values were also found higher in granules (Table 2). There were more OTUs associated with the granules (Fig. 2c), which confirms the richness and evenness of archaea in granules. Flocs contained only 104 OTUs whereas the granules were found to contain 171 OTUs. The granules and flocs were found to share 70 OTUs (Fig. 3c), which represents 67% of the floc OTUs and 41% of the granule OTUs.
Granules contain 2 phyla and 17 genera of archaea whereas the flocs contain 2 phyla and 12 genera (Fig. 4c). Euryarchaeota and Thaumarchaeota were observed to be dominant phyla in both granules (65 and 36%) and flocs (64 and 37%), respectively (Fig. 4c1). Methanosaeta was the most abundant genera in both flocs (60.2%) and granules (53.9%) (Fig. 4c2). The methanogens including Methanobacterium, Methanobrevibacter, Methanosphaera, Methanolinea, Methanospirillum, Methanomethylovorans and Methanosarcina were higher in granules. The abundant Methanosaeta populations suggest that though the methanogens in flocs and granules are exposed to oxygen, they remain viable in the sludge signifying that they may be metabolically active. The presence of Methanosaeta populations in sludge has been reported previously in the literature (Gray et al. 2002; Hiraswa et al. 2008).
Discussion
In this study, bacterial populations were found to be the dominant microorganism in the sludge (38.8%), followed by fungi (33.4%) and archaea (27.8%) (Table 1). These microbial compositions are similar to previously reported studies in WWTP treating industrial wastewater (Yang et al. 2012). In the bacterial community, Planctomycetes were found to favor granules (Fig. 4a1), which may be due to their affinity for salty and anaerobic conditions. The influent wastewater contains high concentrations of salts (Li et al. 2014) and the anaerobic environment of the granules would provide the ideal ecosystem for Planctomycetes. Planctomycetes, Proteobacteria, Acinetobacter and Flavobacterium detection in the AGS, suggested that in addition to producing extracellular polymeric substances favoring granulation they also contributed to the degradation of organic compounds (Adav et al. 2008; Jungles et al. 2011). Extracellular polymeric substances are beneficial in granules formation providing a matrix that offers suitable environments for other microbial cells to inhabit.
Even though bacteria were observed to be the major population, the minor communities of archaea and fungi also contributed to the ecology of AGS structures. In the fungal population, Scopulariopsis and Penicillium were found to be a major part of the AGS. These organisms have been suggested to assist in degradation of organic pollutants such as soluble protein and sugar and the improvement of sludge settleability (Subramanian et al. 2008, 2010; More et al. 2010). Fungi have been suggested to serve as a backbone for floc formation benefitting the granulation of sludge (Morgenroth et al. 1997; Subramanian et al. 2008, 2010).
In archaea, Methanosaeta were observed to be the dominant genera in AGS, similar to earlier studies of archaeal communities in AS systems (Morales et al. 2013). Our observation in agreement with literature suggests that Methanosaeta plays an important role in the structural integrity of granules and degradation of pollutants present in wastewater (Enrigt et al. 2007; Mckeown et al. 2009). Ammonia-oxidizing archaea were not observed in the sludge. However, ammonia oxidizing bacteria (Nitrosomonas and Nitrospira) were detected, indicating that these bacteria may have supplanted the ammonia oxidizing archaea in the high salt environment of the industrial wastewater.
Even though the AGS granules and flocs were cultivated in the same bioreactor, different biodiversity and richness were observed between them (Tables 1, 2; Figs. 2, 3). Microbial diversity is closely related to wastewater characteristic and sludge structure (Gao et al. 2011; Show et al. 2011). In the AGS bacteria and archaea were observed to be higher in the granules, while fungal populations were higher in flocs. As fungi are aerobic species their association with aerobic environment provided by the flocs is not surprising. The lower association of fungi in granules supports the idea that granules provide anaerobic environmental conditions in the sludge (Cydzik-Kwiatkowska and Wojnowska-Baryła 2015; Gonzalez-Gil and Holliger 2014; Weber et al. 2009a, b).
Therefore, our study supports a notion the sludge structure and composition is a primary factor in the biodiversity and richness of the AGS. Excellent granules settleability provides a unique environment retaining anaerobic organisms, which would not usually thrive in the aerobic conditions of WWTP.
Conclusions
The following conclusion could be drawn from the above study;
-
1.
The AGS process was capable of treating mixed influent wastewater from both municipal and industrial origin.
-
2.
Microbial analysis revealed a unique community in the sludge that was dominated by bacterial populations in comparison to Fungi and Archaea.
-
3.
The AGS was composed structurally of both granules and flocs where bacterial and archaeal populations were observed to be higher in the granules while fungal populations were higher in flocs.
-
4.
These results demonstrated that the shape and structural property of granules and flocs favored and maintained the unique microbial compositions.
-
5.
Wastewater characteristics associated with the mixing of industrial and domestic influent also played an important role in maintaining microbial richness and evenness in the AGS.
References
Adav SS, Lee DJ, Show KY, Tay JH (2008) Aerobic granular sludge: recent advances. Biotechnol Adv 26(5):411–423
APHA (2006) Standard methods for the examination of water and wastewater, 21st edn. American Public Health Association, Washington
Cydzik-Kwiatkowska A, Wojnowska-Baryła I (2015) Nitrogen-converting communities in aerobic granules at different hydraulic retention times (HRTs) and operational modes. World J Microbiol Biotechnol 31:75–83
de Kreuk MK (2006) Aerobic granular sludge: scaling up a new technology. Delft University of Technology, TU Delft
Enrigt AM, Collins G, Flaherty VO (2007) Temporal microbial diversity changes in solvent degrading anaerobic granular sludge from low temperature (15 °C) wastewater treatment bioreactors. Syst Appl Microbiol 30(6):471–482
Gao DW, Liu L, Liang H, Wu WM (2011) Aerobic granular sludge: characterization, mechanism of granulation and application to wastewater treatment. Crit Rev Biotechnol 31(2):137–152
Giesen A, de Bruin LMM, Niermans RP, van der Roest HF (2013) Advancements in the application of aerobic granular biomass technology for sustainable treatment of wastewater. Water Pract Technol 8(1):47–54
Gonzalez-Gil G, Holliger C (2014) Aerobic granules: microbial landscape and architecture, stages, and practical implications. Appl Environ Microbiol 80(11):3433–3441
Gray ND, Miskin IP, Kornilova O, Curtis TP, Head IM (2002) Occurrence and activity of archaea in aerated activated sludge wastewater treatment plants. Environ Microbiol 4(3):158–168
Guo F, Zhang SH, Yu X, Wei B (2011) Variations of both bacterial community and extracellular polymers: the inducements of increase of cell hydrophobicity from biofloc to aerobic granular sludge. Bioresour Technol 102(11):6421–6428
Hiraswa JS, Sarti A, Aguila NKS, Varesche MBA (2008) Application of molecular techniques to evaluate the methanogenic archaea and anaerobic bacteria in the presence of oxygen with different COD: sulfate ratios in a UASB reactor. Anaerobe 14(4):209–210
Huang KL, Zhang XX, Shi P, Wu B, Ren HQ (2014) A comprehensive insight into bacterial virulence in drinking water using 454 pyrosequencing and illumina high-throughput sequencing. Ecotoxicol Environ Saf 109:15–21
Huang WL, Li B, Zhang C, Zhang ZY, Lei ZF, Lu BW, Zhou BL (2015) Effect of algae growth on aerobic granulation and nutrients removal from synthetic wastewater by using sequencing batch reactors. Bioresour Technol 179:187–192
Hughes JB, Hellmann JJ, Ricketts TH, Bohannan BJ (2001) Counting the uncountable: statistical approaches to estimating microbial diversity. Appl Environ Microbiol 67(10):4399–4406
Jungles MK, Figueroa M, Morales N, Val del Río A, da Costa RHR, Campos JL, Mendez R (2011) Start up of a pilot scale aerobic granular reactor for organic matter and nitrogen removal. J Chem Technol Biotechnol 86(5):763–768
Khan MZ, Mondal PK, Sabir S (2013) Aerobic granulation for wastewater bioremediation: a review. Can J Chem Eng 91(6):1045–1058
Lee DJ, Chen TY, Show KY, Whiteley CG, Tay JH (2010) Advances in aerobic granule formation and granule stability in the course of storage and reactor operation. Biotechnol Adv 28(6):919–934
Li J, Ding LB, Cai A, Huang GX, Horn H (2014) Aerobic sludge granulation in a full-scale sequencing batch reactor. BioMed Res Int. doi:10.1155/2014/268789
Liu H, Li GW, Li XF, Chen J (2008) Molecular characterization of bacterial community in aerobic granular sludge stressed by pentachlorophenol. J Environ Sci (China) 20(10):1243–1249
Liu YQ, Kong YH, Tay JH, Zhu JR (2011) Enhancement of start-up of pilot-scale granular SBR fed with real wastewater. Sep Purif Technol 82:190–196
Mckeown RM, Scully C, Enrigt AM, Chinalia FA, Lee C, Mahony T, Collins G, O’Flaherty V (2009) Psychrophilic methanogenic community development during long term cultivation of anaerobic granular biofilms. ISME J 3(11):1231–1242
McLellan SL, Huse SM, Mueller-Spitz SR, Andreishcheva EN, Sogin ML (2010) Diversity and population structure of sewage-derived microorganisms in wastewater treatment plant influent. Environ Microbiol 12(2):378-392
Morales N, Figueroa M, Fra-Vázquez A, Val del Río A, Campos JL, Mosquera-Corral A, Méndez R (2013) Operation of an aerobic granular pilot scale SBR plant to treat swine slurry. Process Biochem 48(8):1216–1221
More TT, Yan S, Tyagi RD, Surampalli RY (2010) Potential use of filamentous fungi for wastewater sludge treatment. Bioresour Technol 101(20):7691–7700
Morgenroth E, Sherden T, Van Loosdrecht MCM, Heijnen JJ, Wilderer PA (1997) Aerobic granular sludge in a sequencing batch reactor. Water Res 31(12):3191–3194
Ni BJ, Xie WM, Liu SG, Yu HQ, Wang YZ, Wang G, Dai XL (2009) Granulation of activated sludge in a pilot-scale sequencing batch reactor for treatment of low-strength municipal wastewater. Water Res 43(3):751–761
Rocktaschel T, Klarmann C, Ochoa J, Boisson P, Sorensen K, Horn H (2015) Influence of the granulation grade on the concentration of suspended solids in the effluent of a pilot scale sequencing batch reactor operated with aerobic granular sludge. Sep Purif Technol 142:234–241
Roesch L, Fulthorpe R, Riva A, Casella G, Hadwin A, Ken AD, Daroub SH, Canargo FA, Farmerie WG, Triplett EW (2007) Pyrosequencing enumerates and contrasts soil microbial diversity. ISME J 1(4):283–290
Show KY, Lee DJ, Tay JH (2011) Aerobic granulation: advances and challenges. Appl Biochem Biotechnol 167(6):1622–1640
Subramanian SB, Yan S, Tyagi RD, Surampalli RY (2008) A new, pellet-forming fungal strain: its isolation, molecular identification, and performance for simultaneous sludge-solids reduction, flocculation, and dewatering. Water Environ Res 80(9):840–852
Subramanian S, Yan S, Tyagi R, Surampalli R (2010) SSPRSD using a filamentous fungal strain Penicillium expansum BS30 isolated from wastewater sludge. J Environ Eng 136(7):719–730
Weber SD, Hofmann A, Pilhofer M, Wanner G, Agerer R, Ludwig W, Schleifer KH, Fried J (2009a) The diversity of fungi in aerobic sewage granules assessed by 18S rRNA gene and ITS sequence analyses. FEMS Microbiol Ecol 68(2):246–254
Weber SD, Ludwig W, Schleifer KH, Fried J (2009b) Microbial composition and structure of aerobic granular sewage biofilms. Appl Environ Microbiol 73(19):6233–6240
Yang QX, Wang J, Wang HT, Chen XY, Ren SW, Li XL, Zhang H, Li XM (2012) Evolution of the microbial community in a full-scale printing and dyeing wastewater treatment system. Bioresour Technol 117:155–163
Zhang T, Shao MF, Lin Y (2012) 454 Pyrosequencing reveals bacterial diversity of activated sludge from 14 sewage treatment plant. ISME J 6(6):1137–1147
Acknowledgements
This study was funded by the National Natural Science Foundation of China (51478433). The authors thank Dr. Jonathan Perreault and Philippe Constant for helpful discussions and revision.
Author information
Authors and Affiliations
Corresponding author
Rights and permissions
About this article
Cite this article
Liu, J., Li, J., Tao, Y. et al. Analysis of bacterial, fungal and archaeal populations from a municipal wastewater treatment plant developing an innovative aerobic granular sludge process. World J Microbiol Biotechnol 33, 14 (2017). https://doi.org/10.1007/s11274-016-2179-0
Received:
Accepted:
Published:
DOI: https://doi.org/10.1007/s11274-016-2179-0