Abstract
The anti-cancer activities of antibiotic anisomycin have been demonstrated in kidney, colon and ovarian cancers whereas its underlying mechanisms are not well elucidated. In this work, we investigated whether anisomycin is effective in sensitizes osteosarcoma cell response to chemotherapy. We show that anisomycin inhibits proliferation via inducing osteosarcoma cell arrest at G2/M phase, accompanied by the increased levels of mitotic marker cyclin B and the decreased levels of Rb and E2F-1. Anisomycin also induces apoptosis in a caspase-dependent manner in osteosarcoma cells. Importantly, anisomycin is less effective in normal control NIH3T3 cells compared to osteosarcoma cells. In addition, anisomycin inhibits osteosarcoma growth in xenograft mouse model and enhances the inhibitory effects of doxorubicin in osteosarcoma in vitro and in vivo. Mechanistically, anisomycin targets mitochondrial biogenesis in osteosarcoma as shown by the decreased mitochondrial membrane potential, suppressed mitochondrial respiration via decreasing complex I activity, reduced ATP production. Furthermore, mitochondrial biogenesis stimulator acetyl-L-Carnitine (ALCAR) significantly rescues the inhibitory effects of anisomycin in osteosarcoma cells. Our work demonstrates that anisomycin is active against osteosarcoma cells and the molecular mechanism of its action is the inhibition of mitochondrial biogenesis.
Similar content being viewed by others
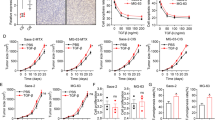
Avoid common mistakes on your manuscript.
Introduction
Osteosarcoma originates from primitive mesenchymal bone-forming cells and is the most prevalent bone cancer affecting children and adolescents worldwide (Abarrategi et al. 2016). Current treatment including surgery and chemotherapy (cisplatin, doxorubicin, and methotrexate) has moderately improved long-term survival rate and the clinical outcome for metastatic osteosarcoma remains poor (Wu et al. 2009). The molecular mechanism underlying osteosarcoma is complex mainly including genomic alterations and activation of oncogenic pathways (eg, Notch, Hedgehog, Wnt and PI3K/Atk) (Angulo et al. 2017). Mutations in retinoblastoma (RB) and p53 genes have been shown to be associated with osteosarcoma pathogenesis (Malkin et al. 1992; Tang et al. 2008). Nevertheless, novel agents and treatment regimens are required. Substantial evidence shows that tumour cells are highly influenced by mitochondrial metabolism (Weinberg and Chandel 2015), therefore, targeting mitochondrial functions may be the rationale for osteosarcoma treatment.
Anisomycin is an antibiotic which is active against protozoa and yeast by inhibiting DNA and protein synthesis (Grollman 1967). Recently, anisomycin has been found to be active against certain types of cancer, such as ovarian cancer, colon cancer and renal carcinoma (Li et al. 2017; Ushijima et al. 2016; Chen et al. 2016; Liu et al. 2014; Abayasiriwardana et al. 2007). It is also an agonist of p38-mitogen activated-protein kinase (MAPK) and c-Jun N-terminal kinase (JNK) (Barros et al. 1997). Anisomycin has been shown to sensitize glucocorticoid-resistant leukaemia cells to dexamethasone-induced apoptosis through p38-MAPK/JNK (Liu et al. 2014).
In this work, we investigated the in vitro and in vivo efficacy of anisomycin alone and whether it can enhance doxorubicin’s effects in osteosarcoma. We demonstrate that anisomycin is effective against osteosarcoma and acts synergistically with doxorubicin. We further demonstrate that the mechanism of action of anisomycin in osteosarcoma is the inhibition of mitochondrial biogenesis.
Materials and methods
Cells and drugs
Human osteosarcoma cell lines MG-63 and U-2 OS were purchased from ATCC Inc. Normal fibroblast cell line NIH3T3 was obtained from Shanghai Institute of Cell Biology and Biochemistry. According to ATCC’s recommendation, MG-63 and U-2 OS cells were cultured in Eagle’s Minimum Essential Medium and ATCC-formulated McCoy’s 5a Medium Modified (Catalog No. 30–2007), respectively. Both media were supplemented with 10% fetal bovine serum. Anisomycin, acetyl-L-carnitine (ALCAR), Z-VAD-fmk and doxorubicin (Sigma, US) were dissolved in DMSO.
MTS assay
Cells proliferation activities were measured using MTS Cell Proliferation Assay Kit according to manufacturer’s instruction (Abcam, US). Briefly, cells were incubated with drug for 72 h. MTS tetrazolium compound was then added to the medium and incubated for 2–4 h. Absorbance was measured at 495 nm.
Flow cytometry
Drug-treated cells were stained with Annexin V-FITC and 7-AAD (BD Pharmingen, US) or Propidium iodide (PI) for apoptosis or cell cycle analysis according to manufacturer’s instruction. Stained cells were quantified by flow cytometry on a Beckman Coulter FC500. 5000 events were counted. The percentage of Annexin V-positive cells, Go/G1, S and G2/M were determined by CXP software.
Caspase activity assay
Caspase 9 or 3 activity was measured using Caspase 9 or 3 Assay Kit (Abcam, US) according to manufacturer’s instructions. Briefly, cells were treated with the drug for 24 h and then lysed. 2× Reaction Buffer (containing 10 mM DTT) and 4 mM LEHD-p-NA or DEVD-p-NA substrate were added to cell lysis for caspase 9 or 3 activity measurement. Absorbance was read at 405 nm.
Mitochondrial function assays
Oxygen consumption rate (OCR) at basal condition was measured using a Seahorse XF96 extracellular flux analyser (Seahorse Bioscience, US) according to Seahorse Bioscience protocols. The mitochondrial potential was measured by labelling cells with 5,5′,6,6′-tetrachloro-1,1′,3,3′-tetraethyl benzimidazolylcarbocyanine iodide (JC-1, Invitrogen) and analysed using flow cytometry on a Beckman Coulter FC500. ATP levels were measured using ATPlite Luminescent Assay kit (Perkin Elmer, US). Mitochondrial respiratory complex I and II activities were measured using Mitochondrial Complex I and II Activity Assay Kits (Novagen, US) according to manufacturers’ instructions.
Western blot analyses
Cells were lysed in 4% SDS lysis buffer containing protease inhibitor cocktail (Roche, US). Protein concentrations were determined by bicinchoninic acid protein assay kit (Thermo Scientific, US). Proteins were loaded for denaturing sodium dodecyl sulfate–polyacrylamide gel electrophoresis and then Western blot by using antibodies including anti-Cyclin B1, anti-Rb, anti-E2F-1 and anti-β-actin (Santa Cruz Inc., US).
Osteosarcoma xenograft in SCID mouse
Animal experiments were performed under the protocol approved by Yangtze University. SCID mice were inoculated with five million MG-63 cells under the flank skin. Following the development of the palpable tumours, the mice were intraperitoneally injected with vehicle alone, doxorubicin (0.5 mg/kg) alone, anisomycin (40 mg/kg) alone or combination of doxorubicin and anisomycin. Tumour length and width were measured every three days and size was determined using the formula: length x width2 × 0.5236.
Statistical analyses
All data are expressed as the mean and standard deviation (SD) to indicate data variability. Statistical analyses were performed by unpaired Student’s t test, with p-value <0.05 considered statistically significant.
Results
Anisomycin inhibits proliferation of osteosarcoma cells via arresting cell cycle at G2/M
We firstly performed MTS assay to investigate the effect of anisomycin on the proliferation of two human osteosarcoma cell lines. As shown in Fig. 1a, anisomycin at 10, 20 and 40 μM dose-dependently inhibited proliferation of MG-63 and U-2 OS cells. In contrast, anisomycin at 10 and 20 μM did not affect proliferation of normal fibroblast cell NIH3T3 (Supplementary Fig. S1A). Although anisomycin at 40 μM significantly inhibited proliferation of NIH3T3, it is less effective in NIH3T3 compare to osteosarcoma cells. To examine how anisomycin inhibits proliferation, we next performed cell cycle analysis using PI staining followed by flow cytometry. As shown in Fig. 1b, the percentage of cells stayed in G2/M phase was significantly increased after anisomycin treatment, indicating that anisomycin induces G2/M arrest. The effect of anisomycin in preventing progression through mitosis is further confirmed by the increased levels of mitotic marker protein cyclin B1 in anisomycin-treated osteosarcoma cells (Fig. 1c). Consistently, anisomycin decreased the protein levels of Rb and E2F-1in osteosarcoma cells (Fig. 1c).
Anisomycin arrests cell cycle at G2/M phase in osteosarcoma cell lines. a Anisomycin treatment for 72 h significantly inhibits proliferation of MG-63 and U-2 OS cells as assessed by MTS assay. b Anisomycin treatment for 24 h increases G2/M phase in osteosarcoma cells. c Representative western blot photo showing the levels of cyclin B1, Rb and E2F-1 in cells treated with anisomycin for 24 h. *, p < 0.05, compared to control
Anisomycin induces apoptosis of osteosarcoma cells in a caspase-dependent manner
As changes in cell cycle kinetics often lead to induction of apoptosis, we performed Annexin V assay to investigate whether anisomycin induces apoptosis. Anisomycin significantly increased the percentage of Annexin V-positive cells (Fig. 2a), demonstrating that anisomycin induces apoptosis in osteosarcoma cells. In addition, anisomycin at 40 but not 10 or 20 μM induced apoptosis in NIH3T3 cells (Supplementary Fig. S1B). Caspases play essential roles in apoptosis initiation and execution. We therefore conducted caspase 9 and 3 colorimetric assay examine their involvement. Expectedly, anisomycin significantly increased activities of caspase 9 and 3 in MG-63 and U-2 OS cells (Fig. 2b and c), demonstrating that anisomycin induces apoptosis via caspase pathways. To confirm that anisomycin induces apoptosis in a caspase-dependent manner, we treated cells with anisomycin in the presence of a pan-caspase inhibitor Z-VAD-fmk (Yang et al. 2004). We found that Z-VAD-fmk completely reversed the effect of anisomycin in inducing apoptosis (Fig. 2d).
Anisomycin significantly induces apoptosis of osteosarcoma cells in a caspase-dependent manner. a Annexin V-positive cells are increased by anisomycin treatment for 72 h. Anisomycin treatment for 24 h increases caspase 9 (b) and 3 (c) activities in MG-63 and U-2 OS cells. d Z-VAD-fmk abolishes the effect of anisomycin in inducing apoptosis. Anisomycin at 40 μM and Z-VAD-fmk at 50 μM were used. *, p < 0.05, compared to control
Anisomycin targets osteosarcoma cells through inhibition of mitochondrial biogenesis
Accumulating evidence indicate that mitochondrial functions are required for tumorigenesis (Weinberg and Chandel 2015). Various antibiotics targeting protein synthesis in bacteria have been shown to inhibit mitochondrial biogenesis in mammalian cells (Lamb et al. 2015; Kalghatgi et al. 2013). Since anisomycin is known to inhibit protein synthesis (Grollman 1967), we examined whether anisomycin affects mitochondrial biogenesis in osteosarcoma cells.
Anisomycin decreased mitochondrial membrane potential in MG-63 and U-2 OS cells (Fig. 3a). Anisomycin also decreased OCR levels as measured by the Seahorse XF96 extracellular flux analyzer in osteosarcoma cells (Fig. 3b), suggesting its inhibitory effect on mitochondrial respiration. Mitochondrial respiratory complex activity analysis showed that anisomycin suppressed mitochondrial respiratory complex I but not II in osteosarcoma cells (Fig. 3c). In addition, ATP production was decreased by anisomycin (Fig. 3d). Acetyl-L-Carnitine (ALCAR), a known mitochondrial biogenesis stimulator fuel (Cassano et al. 2006), significantly rescued anisomycin’s inhibitory effects in osteosarcoma cells (Fig. 3e and f). These results clearly demonstrate that anisomycin targets osteosarcoma cells via inhibiting mitochondrial biogenesis.
Anisomycin targets osteosarcoma cells through inhibiting mitochondrial biogenesis. Anisomycin decreases mitochondrial membrane potential (a), mitochondrial respiration (b) in MG-63 and U-2 OS cells. Anisomycin inhibits mitochondrial respiratory complex I but not II activity in osteosarcoma cells (c). Anisomycin decreases ATP production in osteosarcoma cells. Cells were treated with anisomycin for 24 h prior to mitochondrial function assays. Mitochondrial biogenesis stimulator ALCAR rescues the effects of anisomycin in inhibiting proliferation (e) and inducing apoptosis (f) in osteosarcoma cells. *, p < 0.05, compared to control
Anisomycin enhances doxorubicin’s inhibitory effect in osteosarcoma
We investigated whether anisomycin enhances chemotherapy efficacy in osteosarcoma. We exposed osteosarcoma cells to anisomycin or doxorubicin alone, or combination of anisomycin and doxorubicin. We found that the combination is significantly more effective than anisomycin or doxorubicin alone in inhibiting proliferation and inducing apoptosis in cultured osteosarcoma cells (Fig. 4a and b), suggesting that anisomycin enhances doxorubicin’s effects in vitro.
Anisomycin enhances the inhibitory effects of doxorubicin in osteosarcoma in vitro and in vivo. Combination of anisomycin and doxorubicin inhibits more proliferation (a) and induces more apoptosis (b) than anisomycin or doxorubicin alone. Anisomycin at 10 μM and doxorubicin at 10 nM were used in combination studies. c Representative images of MG-63 tumor isolated from mice treated with control, doxorubicin or anisomycin alone, or combination of doxorubicin and anisomycin. d Combination of anisomycin and doxorubicin is more effective in arresting tumor growth than anisomycin or doxorubicin alone. *p < 0.05, compared to single arm treatment
We next challenged anisomycin in a xenograft osteosarcoma mouse model and investigated in vivo efficacy of the combination of anisomycin and doxorubicin. We did not observe significant body weight loss or abnormal appearance in mice treated with anisomycin alone at 40 mg/kg, doxorubicin alone at 0.5 mg/kg or combination of both (data not shown), suggesting that anisomycin at 40 mg/kg is not toxic to mice. In contrast, smaller tumor size and decreased tumor growth were seen in mice given by anisomycin treatment (Fig. 4c and d). Importantly, a significant further decrease in tumor growth was observed in mice given by anisomycin and doxorubicin combination compared to anisomycin or doxorubicin alone (Fig. 4c and d), demonstrating that the synergism between anisomycin and doxorubicin in osteosarcoma.
Discussion
The anticancer effects of inhibiting translation machinery have been well documented (Bhat et al. 2015; Novac et al. 2004). Following the concept of “translational inhibition targets cancer”, we speculate that anisomycin may be a good candidate for cancer treatment. Anisomycin is a known protein synthesis inhibitor which inhibits translation by binding to the ribosomal 28S rRNA in the 60S subunit (Grollman 1967; Song et al. 2016). In this work, we investigated the efficacy of anisomycin in osteosarcoma using cultured cells as well as in vivo preclinical model. Here, we report that the antibiotic anisomycin has toxicity for osteosarcoma cells both in vitro and in vivo. In addition, we provide evidence that anisomycin acts on osteosarcoma cells via inhibiting mitochondrial biogenesis.
We first demonstrated the efficacy of anisomycin using two osteosarcoma cell lines: MG-63 and U-2 OS, which represents the different cellular origin and genetic background of osteosarcoma. Anisomycin inhibits proliferation and induces caspase-dependent apoptosis in both MG-63 and U-2 OS cells (Figs. 1 and 2), suggesting that anisomycin is effective in targeting osteosarcoma cells. E2F-1 and Rb play the crucial role in the control of cell cycle by coordinating the expression of genes involved in DNA replication and cell cycle progression (Wu et al. 2001). The reduction of E2F-1 and RB in osteosarcoma cells exposed to anisomycin (Fig. 1c) suggests that cell cycle is arrested. We further show that anisomycin arrests cell cycle at G2/M phase as assessed by flow cytometry of PI staining and the mitotic marker protein cyclin B1 is upregulated in anisomycin-treated cells (Fig. 1b and c). These data are well correlated and clearly demonstrate cell cycle arrest by anisomycin. Interestingly, anisomycin induces cell cycle arrest at G2/M phase in osteosarcoma (Fig. 1b and c) and colorectal cancer cell (Ushijima et al. 2016) whereas G0/G1 phase in acute lymphoblastic leukemia cell (Liu et al. 2014), suggesting that anisomycin induces cell cycle arrest in a cell-specific manner. Importantly, NIH3T3 cells are less sensitive to anisomycin than osteosarcoma cells (Supplementary Fig. S1). Anisomycin is also effective in inhibiting tumor growth in the xenograft osteosarcoma mouse model without significant toxicity (Fig. 4c and d). The results of in vitro studies using NIH3T3 cells and in vivo mouse model are consistent with each other, demonstrating the anti-osteosarcoma activity of anisomycin and less toxicity of anisomycin to normal cells. The inhibitory effects of anisomycin we observed on osteosarcoma support the previous report on its anticancer activities. Our work adds osteosarcoma to the growing list of anisomycin targeted cancers.
One approach to developing therapies for osteosarcoma is to combine current chemotherapy with an anticancer agent that targets essential component of cancer cells. It has been reported that anisomycin enhances the inhibitory activities of 5-FU and dexamethasone in colorectal cancer and lymphoblastic leukemia (Ushijima et al. 2016; Liu et al. 2014). In agreement with these studies, the combination of anisomycin and doxorubicin significantly improves the efficacy of doxorubicin on osteosarcoma in vitro as well as in vivo (Fig. 4), suggesting that the combination can eliminate doxorubicin-resistant osteosarcoma cells. The synergism between anisomycin and chemotherapeutic agents makes anisomycin an attractive candidate for cancer treatment.
Although the anticancer activity of anisomycin has been shown in various types of cancers, the underlying mechanisms are not well elucidated. Some demonstrate that the anti-cancer activities of anisomycin are attributed to its activation of p38-MAPK/JNK or targeting BACE1 (Chen et al. 2016; Liu et al. 2014; Abayasiriwardana et al. 2007). We are the first to demonstrate that the inhibitory effects of anisomycin in osteosarcoma are attributed to its inhibition of mitochondrial biogenesis. Anisomycin decreases membrane potential and suppresses mitochondrial respiration via decreasing mitochondrial respiratory complex I but not II activity (Fig. 3a to c). Notably, E2F-1 has been demonstrated to regulate oxidative metabolism by controlling genes that regulate energy homeostasis and mitochondrial functions in muscle tissue (Blanchet et al. 2011). The decreased levels of E2F-1 and mitochondrial dysfunction observed in our study also suggest a possible association between E2F-1 level and proper mitochondrial function in osteosarcoma cells. The mitochondrial dysfunctions induced by anisomycin then lead to energy crisis (Fig. 3d). In contrast, mitochondrial biogenesis stimulator ALCAR reverses the inhibitory effects of anisomycin in osteosarcoma cells in aspects of growth and survival (Fig. 3e and f), confirming that anisomycin acts on osteosarcoma via inhibiting mitochondrial biogenesis. Many antibiotics that target mitochondrial functions, such as tigecycline and levofloxacin, have been shown to display more inhibitory effects on cancer cells (eg, leukemia and breast cancer) compared to normal cells (Yu et al. 2016; Skrtic et al. 2011). Our work shows that anisomycin acts on osteosarcoma cells through inhibiting mitochondrial biogenesis, such as oxidative phosphorylation and ATP production. The mechanisms of the action of anisomycin might explain its selective activity to osteosarcoma cells rather than normal cells. Targeting mitochondrial metabolism has recently attracted attention for cancer therapy. However, the therapeutic value of targeting mitochondria in osteosarcoma remains largely unknown. Besides identifying the anti-osteosarcoma activity of anisomycin, our work also serves as the “proof-of-concept” to demonstrate the essential roles of mitochondria in osteosarcoma.
In conclusion, we show that anisomycin targets osteosarcoma cells through inhibition of mitochondrial biogenesis. Blockage of mitochondrial biogenesis significantly enhances the efficacy of current chemotherapy agent. Therefore, targeting mitochondrial biogenesis may represent a new therapeutic strategy against osteosarcoma.
References
Abarrategi A, Tornin J, Martinez-Cruzado L, Hamilton A, Martinez-Campos E, Rodrigo JP et al (2016) Osteosarcoma: cells-of-origin, cancer stem cells, and targeted therapies. Stem Cells Int 2016:3631764. https://doi.org/10.1155/2016/3631764.
Abayasiriwardana KS, Barbone D, Kim KU, Vivo C, Lee KK, Dansen TB et al (2007) Malignant mesothelioma cells are rapidly sensitized to TRAIL-induced apoptosis by low-dose anisomycin via Bim. Mol Cancer Ther 6(10):2766–2776. https://doi.org/10.1158/1535-7163.MCT-07-0278
Angulo P, Kaushik G, Subramaniam D, Dandawate P, Neville K, Chastain K et al (2017) Natural compounds targeting major cell signaling pathways: a novel paradigm for osteosarcoma therapy. J Hematol Oncol 10(1):10. https://doi.org/10.1186/s13045-016-0373-z.
Barros LF, Young M, Saklatvala J, Baldwin SA (1997) Evidence of two mechanisms for the activation of the glucose transporter GLUT1 by anisomycin: p38(MAP kinase) activation and protein synthesis inhibition in mammalian cells. J Physiol 504(Pt 3):517–525
Bhat M, Robichaud N, Hulea L, Sonenberg N, Pelletier J, Topisirovic I (2015) Targeting the translation machinery in cancer. Nat Rev Drug Discov 14(4):261–278. https://doi.org/10.1038/nrd4505
Blanchet E, Annicotte JS, Lagarrigue S, Aguilar V, Clape C, Chavey C et al (2011) E2F transcription factor-1 regulates oxidative metabolism. Nat Cell Biol 13(9):1146–1152. https://doi.org/10.1038/ncb2309
Cassano P, Sciancalepore AG, Pesce V, Fluck M, Hoppeler H, Calvani M et al (2006) Acetyl-L-carnitine feeding to unloaded rats triggers in soleus muscle the coordinated expression of genes involved in mitochondrial biogenesis. Biochim Biophys Acta 1757(9–10):1421–1428. https://doi.org/10.1016/j.bbabio.2006.05.019
Chen Q, Liu X, Xu L, Wang Y, Wang S, Li Q et al (2016) Long non-coding RNA BACE1-AS is a novel target for anisomycin-mediated suppression of ovarian cancer stem cell proliferation and invasion. Oncol Rep 35(4):1916–1924. https://doi.org/10.3892/or.2016.4571
Grollman AP (1967) Inhibitors of protein biosynthesis. II. Mode of action of anisomycin. J Biol Chem 242(13):3226–3233
Kalghatgi S, Spina CS, Costello JC, Liesa M, Morones-Ramirez JR, Slomovic S et al (2013) Bactericidal antibiotics induce mitochondrial dysfunction and oxidative damage in mammalian cells. Sci Transl Med 5(192):192ra85. https://doi.org/10.1126/scitranslmed.3006055
Lamb R, Ozsvari B, Lisanti CL, Tanowitz HB, Howell A, Martinez-Outschoorn UE et al (2015) Antibiotics that target mitochondria effectively eradicate cancer stem cells, across multiple tumor types: treating cancer like an infectious disease. Oncotarget 6(7):4569–4584. 10.18632/oncotarget.3174
Li Y, Wu X, Jin X, Wang J, Togo Y, Suzuki T et al (2017) Enhancement of death receptor 4-mediated apoptosis and cytotoxicity in renal cell carcinoma cells by anisomycin. Anti-Cancer Drugs 28(2):180–186. https://doi.org/10.1097/CAD.0000000000000450
Liu Y, Ge J, Li Q, Guo X, Gu L, Ma ZG et al (2014) Low-dose anisomycin sensitizes glucocorticoid-resistant T-acute lymphoblastic leukemia CEM-C1 cells to dexamethasone-induced apoptosis through activation of glucocorticoid receptor and p38-MAPK/JNK. Leuk Lymphoma 55(9):2179–2188. https://doi.org/10.3109/10428194.2013.866664
Malkin D, Jolly KW, Barbier N, Look AT, Friend SH, Gebhardt MC et al (1992) Germline mutations of the p53 tumor-suppressor gene in children and young adults with second malignant neoplasms. N Engl J Med 326(20):1309–1315. https://doi.org/10.1056/NEJM199205143262002
Novac O, Guenier AS, Pelletier J (2004) Inhibitors of protein synthesis identified by a high throughput multiplexed translation screen. Nucleic Acids Res 32(3):902–915. https://doi.org/10.1093/nar/gkh235
Skrtic M, Sriskanthadevan S, Jhas B, Gebbia M, Wang X, Wang Z et al (2011) Inhibition of mitochondrial translation as a therapeutic strategy for human acute myeloid leukemia. Cancer Cell 20(5):674–688. https://doi.org/10.1016/j.ccr.2011.10.015
Song H, Xiong H, Che J, Xi QS, Huang L, Xiong HH et al (2016) Gel-based chemical cross-linking analysis of 20S proteasome subunit-subunit interactions in breast cancer. J Huazhong Univ Sci Technol Med Sci = Hua zhong ke ji da xue xue bao Yi xue Ying De wen ban = Huazhong keji daxue xuebao Yixue Yingdewen ban 36(4):564–570. https://doi.org/10.1007/s11596-016-1626-3.
Tang N, Song WX, Luo J, Haydon RC, He TC (2008) Osteosarcoma development and stem cell differentiation. Clin Orthop Relat Res 466(9):2114–2130. https://doi.org/10.1007/s11999-008-0335-z
Ushijima H, Horyozaki A, Maeda M (2016) Anisomycin-induced GATA-6 degradation accompanying a decrease of proliferation of colorectal cancer cell. Biochem Biophys Res Commun 478(1):481–485. https://doi.org/10.1016/j.bbrc.2016.05.139
Weinberg SE, Chandel NS (2015) Targeting mitochondria metabolism for cancer therapy. Nat Chem Biol 11(1):9–15. https://doi.org/10.1038/nchembio.1712.
Wu L, Timmers C, Maiti B, Saavedra HI, Sang L, Chong GT et al (2001) The E2F1-3 transcription factors are essential for cellular proliferation. Nature 414(6862):457–462. https://doi.org/10.1038/35106593
Wu PK, Chen WM, Chen CF, Lee OK, Haung CK, Chen TH (2009) Primary osteogenic sarcoma with pulmonary metastasis: clinical results and prognostic factors in 91 patients. Jpn J Clin Oncol 39(8):514–522. https://doi.org/10.1093/jjco/hyp057
Yang B, El Nahas AM, Fisher M, Wagner B, Huang L, Storie I et al (2004) Inhibitors directed towards caspase-1 and -3 are less effective than pan caspase inhibition in preventing renal proximal tubular cell apoptosis. Nephron Exp Nephrol 96(2):e39–e51. https://doi.org/10.1159/000076403
Yu M, Li R, Zhang J (2016) Repositioning of antibiotic levofloxacin as a mitochondrial biogenesis inhibitor to target breast cancer. Biochem Biophys Res Commun. https://doi.org/10.1016/j.bbrc.2016.02.072
Acknowledgements
This work was supported by a research grant provided by Xiangyang Central Hospital (201416081869).
Author information
Authors and Affiliations
Corresponding authors
Ethics declarations
Conflicts of interest
All authors declare no conflicts of interest.
Additional information
Chuanhua Cao and Haiying Yu are equal contributors and co-first authors.
Electronic supplementary material
Supplementary Fig. S1
(DOC 32 kb)
Rights and permissions
About this article
Cite this article
Cao, C., Yu, H., Wu, F. et al. Antibiotic anisomycin induces cell cycle arrest and apoptosis through inhibiting mitochondrial biogenesis in osteosarcoma. J Bioenerg Biomembr 49, 437–443 (2017). https://doi.org/10.1007/s10863-017-9734-8
Received:
Accepted:
Published:
Issue Date:
DOI: https://doi.org/10.1007/s10863-017-9734-8