Abstract
Lead-free MnO2-doped K0.5Na0.5Nb0.92Sb0.08O3 ceramics have been fabricated by a conventional ceramic technique and their dielectric and piezoelectric properties have been studied. Our results show that a small amount of MnO2 (0.5–1.0 mol%) is enough to improve the densification of the ceramics and decrease the sintering temperature of the ceramics. The co-effects of MnO2 doping and Sb-substitution lead to significant improvements in the ferroelectric and piezoelectric properties. The K0.5Na0.5Nb0.92Sb0.08O3 ceramic with 0.5 mol%MnO2 doping possesses optimum propeties: d 33 = 187 pC/N, k P = 47.2%, ε r = 980, tanδ = 2.71% and T c = 287 °C. Due to high tetragonal-orthorhombic phase transition temperature (T O-T ~ 150 °C), the K0.5Na0.5Nb0.92Sb0.08O3 ceramic with 0.5 mol%MnO2 doping exhibits a good thermal stability of piezoelectric properties.
Similar content being viewed by others
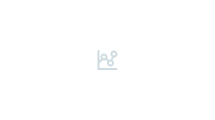
Avoid common mistakes on your manuscript.
1 Introduction
Lead-containing piezoelectric materials with perovskite structure, such as Pb(Zr,Ti)O3 and Pb(Mg1/3Nb2/3)-PbTiO3, have been widely used in electronic and microelectronic devices because of their excellent piezoelectric and ferroelectric properties. However, the strong toxicity and high evaporation of lead oxide during sintering have caused a crucial environmental pollution. Therefore, it is necessary to develop lead-free piezoelectric ceramics with good piezoelectric properties for replacing the lead-containing ceramics in various applications.
Alkali niobate K0.5Na0.5NbO3 (KNN), a solid solution of ferroelectric KNbO3 and antiferroelectric NaNbO3, is considered as a promising candidate for lead-free piezoelectric ceramics because of its high Curie temperature (about 420 °C), good ferroelectric properties (P r = 33 μC/cm2) and large electromechanical coupling factors [1, 2]. However, it is very difficult to obtain dense and well-sintered KNN ceramics using an ordinary sintering process because of the volatility of alkaline elements at high temperature. For a well-sintered KNN ceramic (e.g. prepared by hot-pressing technique), it possesses good piezoelectric properties (d 33 = 160 pC/N, k p = 45%) and high density (ρ = 4.46 g/cm3). [2] However, there is severe degradation in piezoelectric properties (d 33 = 80 pC/N, k p = 36%) and density (ρ = 4.25 g/cm3) for an air-fired KNN ceramics. [1, 3] A number of studies have been carried out to improve the sinterability and electrical properties of KNN ceramics, including the formation of solid solutions such as Li- and Ta-modified KNN [4], KNN-LiSbO3 [5, 6], KNN-BaTiO3 [7], K0.475Na0.475Li0.05NbO3-Bi0.48Na0.48Ba0.04TiO3 [8], KNN-LiNbO3 [9], KNN-Bi0.5Na0.5TiO3 [10] and KNN-Li(Nb,Ta,Sb)O3 [11], and the use of sintering aids, e.g. K5.4Cu1.3Ta10O29 [12] and CuO [13]. It has been known that Sb has a much larger Pauling electronegativity than Nb (2.05 vs. 1.5). Therefore, the partial substitution of Sb5+ for Nb5+ induces a strong covalency in the KNN perovskite, which favors the ferroelectricity and piezoelectricity. [11, 14] It is also noted that MnO2 is an effective dopant in the Pb-containing piezoelectric ceramics to enhance densification and reduce loss tangent tanδ. [15, 16] In the present work, MnO2-doped K0.5Na0.5Nb0.92Sb0.08O3 ceramics, K0.5Na0.5Nb0.92Sb0.08O3 + xmol%MnO2, were prepared by ordinary solid-state sintering, and the effects of MnO2 doping on the microstructures, dielectric and piezoelectric properties of K0.5Na0.5Nb0.92Sb0.08O3 ceramics were investigated.
2 Experimental
K0.5Na0.5Nb0.92Sb0.08O3 + xmol%MnO2 ceramics (0 ≤ x ≤ 3.0) were prepared by an ordinary sintering technique using analytical-grade metal oxides or carbonate powders: Na2CO3 (99.8%), K2CO3 (99.9%), Nb2O5 (99.5%), Sb2O3 (99%) and MnO2 (99%). The stoichiometric K0.5Na0.5Nb0.92Sb0.08O3 powder was first synthesized at 880 °C for 6 h by a solid state reaction. After the calcination, K0.5Na0.5Nb0.92Sb0.08O3 and MnO2 powders were weighed according to the formula of K0.5Na0.5Nb0.92Sb0.08O3 + xmol%MnO2 and then ball-milled for 8 h. The resulting powders were mixed thoroughly with a PVA binder solution and then pressed into disk samples with the diameter of 15.0 mm and thickness of 1.20 mm. The disk samples were finally sintered at 1,080–1,120 °C for 4 h in air. Silver electrodes were fired on the top and bottom surfaces of the sintered samples. The ceramics were poled under a dc field of 5–6 kV/mm at room temperature in a silicon oil bath for 30 min.
The crystalline structure of the sintered samples was examined using X-ray diffraction (XRD) analysis with CuKα radiation (DX-1000). The bulk density ρ was measured by the Archimedes’ method. The microstructures were observed using scanning electron microscopy (JEOL JSM-5900LV). The relative permittivity ε r and loss tangent tanδ of the ceramics at 1, 10 and 100 kHz were measured as functions of temperature using an impedance analyzer (Agilent 4192A). A conventional Sawyer–Tower circuit was used to measure the polarization hysteresis (P-E) loop at 100 Hz. The electromechanical coupling factor k p and electromechanical quality factor Q m were determined by the resonance method according to the IEEE Standard using an impedance analyzer (Agilent 4294A). The piezoelectric coefficient d 33 was measured using a piezo-d 33 meter (ZJ-3A, China).
3 Results and discussions
Figure 1 shows the XRD patterns of K0.5Na0.5Nb0.92Sb0.08O3 + xmol%MnO2 ceramics. From Fig. 1, all the K0.5Na0.5Nb0.92Sb0.08O3 + xmol%MnO2 ceramics possess a pure perovskite structure with orthorhombic symmetry and no second phase can be detected. The XRD patterns of the ceramics have been indexed by JCPDF card 71-2171. The addition of MnO2 to K0.5Na0.5Nb0.92Sb0.08O3 does not cause any significant change to the crystalline structure.
The SEM micrographs of the K0.5Na0.5Nb0.92Sb0.08O3 + xmol%MnO2 ceramics with x = 0, 1.0, 2.0 and 3.0 are shown in Fig. 2, while Fig. 3 shows the variations of the bulk density ρ and optimum sintering temperature T s with x. As shown in Fig. 2a, although the sintering temperature is high (1,120 °C), the K0.5Na0.5Nb0.92Sb0.08O3 + xmol%MnO2 ceramic with x = 0 (i.e., without MnO2 doping) has a very loose structure and a large amount of pores can be found. For the K0.5Na0.5Nb0.92Sb0.08O3 ceramic (i.e. x = 0), the grains have a diameter of about 1.2 μm. However,after the doping of a small amount of MnO2 (≥0.5 mol%), the grain size increases significantly to about 2.5 μm, the ceramics can be well sintered at 1,080–1,110 °C and become considerably dense, and there is almost no pore on the surface of the ceramics (Fig. 2b–d). As shown in Fig. 3, the K0.5Na0.5Nb0.92Sb0.08O3 ceramic without MnO2-doping has a low bulk density of 4.12 g/cm3. On the basis of the XRD results, the relatively density of the ceramic with x = 0 is about 90%. However, after the doping of 0.5–1.0 mol%MnO2, the ceramics give a high density of 4.44–4.48 g/cm3 (relative density > 97%). As x further increase to 3.0, the bulk density decreases slightly to 4.37 g/cm3 (relative density ~ 95%). For each composition, the ceramics were sintered at different temperatures and their density was measured. The optimum sintering temperature was determined as the sintering temperature by which the ceramic has the largest density. From Fig. 3, as x increases, the optimum sintering temperature for the K0.5Na0.5Nb0.92Sb0.08O3 + xmol%MnO2 ceramics decreases. It can be clearly seen that the addition of a small amount of MnO2 to the K0.5Na0.5Nb0.92Sb0.08O3 ceramics results in the significant improvement in densification and a decrease in sintering temperature.
The temperature dependences of ε r and tanδ for K0.5Na0.5Nb0.92Sb0.08O3 + xmol%MnO2 ceramics at 1 kHz are shown in Fig. 4. Similar to the pure KNN [1, 2], two transition peaks are observed: one is associated with the paraelectric cubic-ferroelectric tetragonal phase transition at T c and the other is the ferroelectric tetragonal-ferroelectric orthorhombic phase transition at T O-T. As shown in Fig. 4a, the observed T O-T for K0.5Na0.5Nb0.92Sb0.08O3 + xmol%MnO2 ceramics is insensitive to the doping level x of MnO2. For the ceramics with x = 0.5–3.0, the observed T O-T is about 150 °C. Unlike T O-T, the observed T c increases from 287 to 298 °C with x increasing from 0.5 to 3.0. It can be also seen that as x increases, the value of the dielectric peak at T c increases significantly. From Fig. 4b, for the ceramics with x = 0.5, 1.0, 2.0 and 3.0, the observed tanδ has a very weak dependence on temperature below T c and retain almost unchangeable with temperature increasing. However, as temperature increases above T c, a sharp increase in tanδ is observed in the ceramics with x = 0.5, 1.0, 2.0 and 3.0, which should be attributed to a decrease in electrical resistivity of the ceramics at higher temperatures, at which the ceramics become electrically conductive.
Figure 5 shows the temperature dependences of ε r and tanδ for the K0.5Na0.5NbO3 + 0.5 mol%MnO2 and K0.5Na0.5Nb0.92Sb0.08O3 + 0.5 mol%MnO2 ceramics at 1, 10 and 100 kHz. It can be seen that the K0.5Na0.5NbO3 + 0.5 mol%MnO2 ceramic (i.e., Sb-free KNN ceramic with 0.5 mol%MnO2 doping) retains the classic ferroelectric characteristic, and undergoes the cubic-tetragonal phase transition at 421 °C (T c) and the tetragonal-orthorhombic phase transition at 210 °C (T O-T) (Fig. 5a). After the substitution of 8% Sb5+ for Nb5+, the ceramic (i.e., K0.5Na0.5Nb0.92Sb0.08O3 + 0.5 mol%MnO2) undergoes the same two phase transitions, with both T c and T O-T shifted to lower temperatures (287 and 151 °C, respectively) (Fig. 5b).
The K0.5Na0.5NbO3 + 0.5 mol%MnO2 ceramic is a normal ferroelectric, thus exhibiting a sharp transition peak at T c in the plot of ε r versus temperature as shown in Fig. 5a. However, the substitution of 8% Sb5+ for Nb5+ in the K0.5Na0.5NbO3 + 0.5 mol%MnO2 ceramic leads to a broadening of the transition peak at T c, suggesting the appearance of a diffuse phase transition. A diffuse phase transition has been observed in many ABO3-type perovskites and Bi-layered structure compounds, e.g. Ba0.5Na0.5TiO3-based ceramics [17], K0.5La0.5Bi2Nb2O9 [18], Pb(Mg1/3Nb2/3)O3 [19], KNN-SrTiO3 [20]. The diffuseness in the phase transition can be determined from the modified Curie–Weiss law 1/εr − 1/ε m = C−1(T − T m)γ [19], where ε m is the maximum value of relative permittivity at the phase transition temperature T m, γ is the degree of diffuseness and C is the Curie-like constant. The γ can have a value ranging from 1 for a normal ferroelectric to 2 for an ideal relaxor ferroelectric.
Based on the temperature plots of εr at 10 kHz, the graphs of ln(1/εr − 1/ε m) versus ln(T − T m) for the K0.5Na0.5NbO3 + 0.5 mol%MnO2 and K0.5Na0.5Nb0.92Sb0.08O3 + 0.5 mol%MnO2 ceramics were plotted, giving the results shown in Fig. 6. Both the samples exhibit a linear relationship. By least-squared fitting the experimental data to the modified Curie–Weiss law, γ was determined. The calculated γ for the K0.5Na0.5NbO3 + 0.5 mol%MnO2 ceramic is 1.17, revealing the normal ferroelectric characteristic. After the substitution of 8 mol%Sb+, γ increases significantly to 1.63, suggesting that the substitution of Sb5+ makes the K0.5Na0.5Nb0.92Sb0.08O3 + 0.5 mol%MnO2 ceramic become more relaxor, thus exhibiting a broadened transition peak as shown in Fig. 5b. The appearance of the diffuse phase transition may be ascribed to the increase in the disorder degree of B-site ions, resulting from the substitution of Sb5+ for Nb5+.
Except for the K0.5Na0.5Nb0.92Sb0.08O3 ceramic (i.e., without MnO2 doping), all the ceramics can be well sintered at 1,080–1,110 °C and have a dense structure, giving a high relative density (>95%), and so exhibit a well-saturated P-E hysteresis loop under an electric field of 5 kV/mm. Figure 7a shows the P-E hysteresis loops of the K0.5Na0.5Nb0.92Sb0.08O3 + xmol%MnO2 ceramics with x = 0.5, 1.5 and 2.5, while the variations of the remanent polarization P r and coercive field E c with x are shown in Fig. 7b. For the K0.5Na0.5Nb0.92Sb0.08O3 + xmol%MnO2 ceramics, P r increases slightly and then decreases as x increases from 0.5 to 3.0, giving a maximum value of 26.8 μC/cm2 at x = 1.5, while E c increases considerably from 0.65 to 0.97 kV/mm with x increasing from 0.5 to 2.0 and then decreases slightly to 0.94 kV/mm with x further increasing to 3.0.
Figure 8 shows the variations of d 33, k p, Q m, ε r and tanδ with x for the K0.5Na0.5Nb0.92Sb0.08O3 + xmol%MnO2 ceramics. After the use of the sintering aid, the density of the ceramics increases significantly from 4.12 to 4.48 (Fig. 3), thus yielding considerable enhancement in d 33, k p, and Q m and reduction in tanδ. The optimum content of MnO2 is 0.5 mol%. It can be seen that because of the poor densification, the ceramic without MnO2 doping exhibits the very high tanδ (65.2%) and relatively poor piezoelectricity (d 33 = 95 pC/N, k p = 22.6%, Q m = 6). After the addition of 0.5 mol%MnO2, the tanδ decrease significantly and reaches a minimum value of 2.71%. The great decrease in tanδ should be ascribed to the great enhancement in densification. For the ceramic with x = 0.5, the good piezoelectric and dielectric properties are obtained: d 33 = 187 pC/N, k p = 47.2%, and Q m = 123 (Fig. 8a), while ε r = 980 and tanδ = 2.71% (Fig. 8b).
Figure 9 shows the temperature dependence of k p for the K0.5Na0.5Nb0.92Sb0.08O3 + 0.5 mol%MnO2 ceramic. It can be seen that for the K0.5Na0.5Nb0.92Sb0.08O3 + 0.5 mol%MnO2 ceramic, there has no depolarization below 120 °C. Because the T O-T is relatively high (151 °C), the K0.5Na0.5Nb0.92Sb0.08O3 + 0.5 mol%MnO2 ceramic exhibits a very good thermal characteristic. It can be seen that as temperature increases above T O-T, the piezoelectricity of the ceramic become weak gradually.
Table 1 shows the electrical properties of K0.5Na0.5Nb0.92Sb0.08O3 + 0.5 mol%MnO2, K0.5Na0.5NbO3 + 0.5 mol%MnO2 and K0.5Na0.5Nb0.92Sb0.08O3 (i.e., without MnO2 doping) ceramics. It can be seen that compared with the K0.5Na0.5Nb0.92Sb0.08O3 + 0.5 mol%MnO2 ceramic, the K0.5Na0.5NbO3 + 0.5 mol%MnO2 ceramic exhibits typical “hardened” electrical characteristics (low d 33, k p, ε r, tanδ and P r; high Q m and E c). After the substitution of 8% Sb5+ for Nb5+, the observed d 33, k p, ε r, tanδ and P r increase by about 68, 8, 200, 145 and 13%, respectively, while the observed Q m and E c decrease by about 63 and 13%, respectively. This suggests that after the substitution of 8% Sb5+ for Nb5+, the ceramics become “softened”, thus giving rise to significant improvements in d 33, k p and ε r. The improvement of piezoelectric properties of the ceramics should be attributed to the strong covalency of Sb. For the K0.5Na0.5Nb0.92Sb0.08O3 + 0.5 mol%MnO2 ceramic, the partial substitution of Sb5+ for Nb5+ induces a much stronger covalency in the KNN-based perovskite, which favors the ferroelectricity and piezoelectricity. It can be also noted that because of the poor densification, the MnO2-undoped K0.5Na0.5Nb0.92Sb0.08O3 ceramic possesses the very poor piezoelectricity, indicating the effectiveness of MnO2 as a sintering aid for improving the sintering performance and electrical properties.
4 Conclusions
K0.5Na0.5Nb0.92Sb0.08O3 + xmol%MnO2 lead-free ceramics have been fabricated by an ordinary sintering technique. Our results show that MnO2 is an excellent sintering aid for improving the sintering performance. A small amount of MnO2 (0.5–1.0 mol%) improves effectively the densification. The high densification and strong covalence of Sb lead to the significant improvement in the ferroelectricity and piezoelectricity of the ceramics. The ceramic with x = 0.5 exhibits excellent ferroelectric and piezoelectric properties: d 33 = 187 pC/N, k P = 47.2%, ε r = 980, tanδ = 2.71%, P r = 24.7 μC/cm2, E c = 0.65 kV/mm and T C = 287 °C. Because of high T O-T, the ceramic with x = 0.5 exhibits a very good temperature stability of piezoelectric properties. Our results also show that the K0.5Na0.5Nb0.92Sb0.08O3 + xmol%MnO2 ceramics exhibit relaxor characteristic, which may be ascribed to the increase in the disorder degree of B-site ions resulting from the partial substitutions of Sb5+ for Nb5+. It is suggested that the K0.5Na0.5Nb0.92Sb0.08O3 + xmol%MnO2 (x = 0.5–1.0) ceramics are an attractive candidate for lead-free ceramics.
References
R.E. Jaeger, L. Egerton, J. Am. Ceram. Soc. 45(5), 209 (1962)
L. Egerton, D.M. Dillom, J. Am. Ceram. Soc. 42(9), 438 (1959)
Z.S. Ahn, W.A. Schulze, J. Am. Ceram. Soc. 70(1), C18 (1987)
E. Hollenstein, M. Davis, D. Damjanovic, N. Setter. Appl. Phys. Lett. 87, 182905 (2005)
G.Z. Zang, J.F. Wang, H.C. Chen, W.B. Su, C.M. Wang, P. Qi, B.Q. Ming, J. Du, L.M. Zheng, S. Zhang, R.T. Shrout, Appl. Phys. Lett. 88, 212908 (2006)
D. Lin, K.W. Kwok, K.H. Lam, H.L.W. Chan, J. Appl. Phys. 101, 074111 (2007)
H.Y. Park, C.W. Ahn, H.C. Song, J.H. Lee, S. Nahm, K. Uchino, H.G. Lee, H.J. Lee, Appl. Phys. Lett. 89, 062906 (2006)
R. Zuo, C. Ye, Appl. Phys. Lett. 91, 062916 (2007)
Y. Guo, K. Kakimoto, H. Ohsato, Appl. Phys. Lett. 85, 4121 (2004)
R. Zuo, X. Fang, C. Ye, Appl. Phys. Lett. 90, 092904 (2006)
Y. Saito, H. Takao, T. Tani, T. Nonoyama, K. Takatori, T. Homma, T. Nagaya, M. Nakamur, Nature 432, 84 (2004)
M. Matsubara, K. Kikuta, S. Hirano, J. Appl. Phys. 97, 114105 (2005)
H. Takao, Y. Saito, Y. Aoki, K. Horibuchi, J. Am. Ceram. Soc. 89, 1951 (2006)
D.J. Singh, M. Ghita, S.V. Halilov, M. Fornari, J. Phys. IV 128, 47 (2004)
C. Galassi, E. Roncari, C. Capiani, F. Craciun, J. Eur. Ceram. Soc. 19(6–7), 1237 (1999)
C.S. Yu, H.L. Hsieh, J. Eur. Ceram. Soc. 25(12), 2425 (2005)
Y. Li, W. Chen, Q. Xu, J. Zhou, X. Gu, S. Fang, Mater. Chem. Phys. 94, 328 (2005)
C. Karthik, N. Ravishankar, K.B.R. Varma, Appl. Phys. Lett. 89, 042905 (2006)
K. Uchino, S. Nomura, L.E. Cross, S.J. Tang, R.E. Newnham, J. Appl. Phys. 51, 1142 (1980)
Y. Guo, K. Kakimoto, H. Ohsato, Solid State Commun. 129, 279 (2004)
Acknowledgment
This work was supported by the project of Education Department of Sichuan Province (08ZA047), and Science and Technology Bureau of Sichuan Province (09ZQ026-059)
Author information
Authors and Affiliations
Corresponding author
Rights and permissions
About this article
Cite this article
Lin, D., Zheng, Q., Kwok, K.W. et al. Dielectric and piezoelectric properties of MnO2-doped K0.5Na0.5Nb0.92Sb0.08O3 lead-free ceramics. J Mater Sci: Mater Electron 21, 649–655 (2010). https://doi.org/10.1007/s10854-009-9971-7
Received:
Accepted:
Published:
Issue Date:
DOI: https://doi.org/10.1007/s10854-009-9971-7