Abstract
This paper reviews published knowledge on how to deal with invasive species during biological quality assessments in European river systems for water management and assessments of ecological quality required, for example, by the European Water Frame Work Directive. The papers studied included international papers and some standards for water assessment. An overview of the current state of neozoa research showed that many different topics are treated, comprising biogeography and fauna records, species replacements and effectiveness of colonisation, life cycles, competition between native and invasive species, habitat quality and pathways of migration. Additionally, some papers have been published recently on the integration of neozoa in index-based assessment systems. Although the decline or increase of alien species populations and the corresponding impacts on indigenous populations were frequently observed, the mechanisms behind the invasions often remain hypothetical. In the reviewed papers, issues such as possible reasons for coexistence, tolerances, quality of habitat or water, life history traits and introduction of diseases were rarely covered. Few neozoa are sufficiently investigated to be categorised as indicators. After discussing the advantages and disadvantages of inclusion or exclusion, inclusion of invaders in assessments of both biodiversity (all species) and human impact (only species classified in their specific tolerance) is suggested. Further research is required to (1) update and assign ecological profiles of the non-indigenous species currently and (2) assess the effects of new invaders on native communities.
Similar content being viewed by others
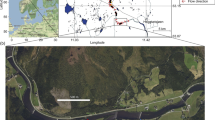
Avoid common mistakes on your manuscript.
Introduction
The aim of this paper is to determine whether aquatic neozoa should be included or neglected in the implementation of ecological quality assessments of aquatic systems (e.g. applying national standards for biological water assessments, which are frequently based on native communities). This question arises from the fact that increasing numbers of non-indigenous species are observed at present, frequently dominating the community and causing problems in the present day water quality assessment. “Neozoa” are “exotic”, “alien” or “non-indigenous species”, described as “invaders” or “invading species”, if they successfully intruded areas where they were not originally found. The local and original community free from invaders is mainly called “indigenous” or “native”. Species not known to be definitely an exotic or a native are named as “cryptogenic”. Recently, the term “biological invasion” has become widely accepted due to the increasing number of studies regarding the presence and dispersal of invasive species. Elliott (2003) suggested to consider such non-indigenous and invasive species and their geographical spread as “biological pollution” and “biological pollutants”, and Arbačiauskas et al. (2008) used the term “biocontamination”. “Biological invasion” is defined as the event in which a population is moved beyond its natural range or natural zone of potential dispersal through human-mediated transport. This term has to be separated from “colonisation” as this is often defined as natural range expansion. Ricciardi and Cohen (2007) stated that the terms indicating neozoa are used inconsistently in literature and among disciplines and further showed that the invasiveness and the impact of an invaded species on the native community or even human economics are not generally linked. This should be kept in mind when nature and effect of neozoa is discussed.
“Biological invasion” has increased in the last decades and is mainly caused by intentional introduction of exotic species, for example marine aquaculture purposes (Crassostrea gigas, Mya arenaria), unintentional co-introduction with oyster transfers (e.g. Crepidula fornicata, Cyclope neritea, Rhithropanopeus harrisii), but mainly increasing international ship traffic as a result of connecting rivers by canals (van der Velde and Platvoet 2007; Gollasch and Nehring 2006; Minchin et al. 2006; Devin et al. 2005; Diederich et al. 2005; Bij de Vaate et al. 2002; Kley and Maier 2003; Mueller et al. 2002; Wilson et al. 1999; and many national papers). Devin et al. (2005) consider this process of invasion as a re-colonization of the geographical area of fauna expelled by the environmental conditions of the glacial periods. Consequently, the structure of the aquatic life communities transformed dramatically in the last years. Nowadays, this transformation is still at a very dynamic state, far from equilibrium of the species composition (Simberloff and Gibbons 2004). From some invaders, the ecological profiles are well known, as they have been studied extensively for many years (e.g. Dreissena polymorpha; see Table 1; Padilla 2005; Bachmann et al. 2001; Piscart et al. 2006 and many others) or in specific studies (e.g. Dikerogammarus villosus; Brooks et al. 2008; Devin et al. 2004; Piscart et al. 2003; Kley and Maier 2003; Bruijs et al. 2001 and others). Also, interactions between different invader species were investigated (Gergs and Rothhaupt 2008; Dieterich et al. 2004; Burlakova et al. 2000; Baker and Hornbach 1997). For other exotic species, some records are known, but the impact on native communities is not clear (e.g. Craspedacusta sowerbyi, Menetus dilatatus, Dendrocoelum romanodanubiale, Obesogammarus crassus; Gollasch and Nehring 2006), making it difficult to estimate their contribution to the indicative power of a local community. There are initiatives to collect the scattered biological knowledge and make it available for the public in concise and practicable form, e.g. printed lists for a quick overview (Gollasch and Leppäkoski 2007; Kerckhof et al. 2007) or comprehensive approaches on online platforms (Aquatic aliens 2009; Baltic Sea Alien Species Database 2009; NOBANIS 2009; Nonindigenous Aquatic Species 2009, as output from EU-Projects DAISIE and ALARM). In DAISIE, a short table on the species requested is given for a first overview of its distribution and impact potential etc., depending on the information available from the various countries. In ALARM, however, the tools and/or their availability are still under construction. Facing the dynamic and occasionally complex situation in the data, it may seem advisable to neglect the neozoa or keep only few, at most, in the studies in order to eliminate uncertainties in data set and evaluation. However, as the impacts of the invaders are often disastrous for the native communities, an invader-dominated community can only be considered as representative for a site if these species are included in the biological assessment.
This review aims to (1) give an overview of the information on the knowledge of distribution, strategies and effects of species invasion and invading species and problems in water assessment and management published so far, (2) discuss the role of invasive species in the local community and their role as bio-indicators and (3) give arguments to include or exclude neozoa in biological quality assessment. The title and the conclusions are focused on European waters. However, inclusion or exclusion of invasive species in assessments is not only a typical European problem, but a generic one. Thus, some arguments and data refer to studies from other regions.
For this review, 159 papers from international journals and internet publications were evaluated. National papers and unpublished reports were not included, as they are not in English and are difficult to access for the international reader. However, significant amounts of data, reports of records and local information are provided in these resources in earlier years. Therefore, these data should not be neglected in more detailed studies. Recently, many papers have also been published in international journals on new records, the distribution, spreading and impact of neozoa on the native fauna. Moreover, the dynamics of the invasion of a single species or the interaction between several species and the many activities and papers on biological and ecological traits, effects and impacts made it impossible to give a complete overview in this review, but rather show trends and most important items. The search for the publications was performed using the search functions in the web of science and zoological records until December 2008. The search terms were “neozoa, invader, alien and macroinvertebrates, brackish, assessment” in various combinations for higher success rates. After viewing the list of titles and the abstracts, the articles containing substantial information were selected for further evaluation following the aims of the review. In few cases, special literature cited in a paper, but not appearing in the search results mentioned above was viewed. Except for the standards and comments on them, only websites and papers printed in scientific journals or books in English language were used. A total of 148 papers were evaluated for the overview in Tables 1 and 2, but using only the titles, key words and abstracts from the electronic search.
General overview
Subject areas and species considered
Most of the papers comprise studies from central European rivers or estuaries, while a smaller number deal with lakes (Gumuliauskaite and Arbaciauskas 2008; Pilollo et al. 2008; Gergs and Rothhaupt 2008; Frischer et al. 2005; Diggins et al. 2004; MacNeil et al. 2001a, b, 2003; Mueller 2001; Mueller et al. 2002; Stucki and Romer 2001). In the North American Great Lakes Area, the occurrence and influence of neozoic species was studied very intensively for Dreissenids, whereas detailed studies on other macrobenthic groups are less frequent (Palmer and Ricciardi 2005; Barbiero and Tuchman 2004; Budd et al. 2001; Dermott and Kerec 1997; Fahnenstiel et al. 1995; Haynes et al. 2005; Kuhns and Berg 1999; MacIsaac et al. 1999; Makarewicz et al. 2000; Ricciardi and Maclsaac 2000; Stewart et al. 1998; Zanatta et al. 2002; Zaranko et al. 1997). In the early 1990s, the number of papers published on neozoa in freshwater systems in Europe was relatively low and restricted to the rivers Rhine and Elbe (Kureck 1992; Paffen et al. 1994; Van den Brink et al. 1993). This situation is similar to the interest for neozoa in American Great Lakes and brackish waters, which also started in the 1990s, mainly because of their enormous impact on the ecosystem functioning and the possible negative effects of neozoic bivalves on aquaculture harvest (e.g. oyster banks) and because of problems in waterworks, power plants and fouling in cooling systems (Le Page 1992; Kovalak et al. 1992).
Of the literature reviewed, 12 studies were based exclusively on laboratory experiments (Brooks et al. 2008; van Riel et al. 2007; Aberle et al. 2005; Cherry et al. 2005; Cope and Winterbourn 2004; Kinzler and Maier 2003; Wijnhoven et al. 2003; Dick and Platvoet 2000; Dick et al. 2002; Bruijs et al. 2001; James et al. 1997; Baker and Hornbach 1997), another ten studies used both laboratory and field methods (Pilollo et al. 2008; Devin et al. 2005; Kelly and Dick 2005; Hakenkamp et al. 2001; Kley and Maier 2003; MacNeil et al. 2003; Montalto and de Drago 2003; Mueller 2001; Nel et al. 1996; Pillsbury et al. 2002), while all others gained their data purely from field studies, with the exception of some reviews (e.g. Gollasch and Nehring 2006; Kerckhof et al. 2007) and standards.
A series of neozooic species have successfully colonised wide areas of western European coastal waters (Potamopyrgus antipodarum, C. gigas, M. arenaria, Cordylophora caspia, Eriocheir sinensis, R. harrisii, D. polymorpha, Gammarus tigrinus, Ficopomatus enigmaticus, Ensis americanus, Balanus improvisus, Corbicula fluminea), while others are only abundant locally (Nereis succinea, Mytilopsis leucophaeata, Petricola pholadiformis). In Belgium, for example, 42 alien brackish and marine species are listed (Kerckhof et al. 2007). From the German North and Baltic Sea, 31 exotic species are recorded (Aquatic aliens 2009). Along the whole course of the middle European River Elbe, 31 non-indigenous macroinvertebrate species are known, including brackish and freshwater species. However, 21 of them were found in, or restricted to, the estuary. Nehring (2006) explained this with a combination of special estuarine adapations and geographical and physical conditions that are not valid for either marine or freshwater habitat conditions. Although several papers concerning bio-invasion were published for most of the neozoic species present in the brackish waters of north-western Europe, special attention should be paid to some freshwater populations of the estuarine colonial hydrozoan C. caspia in the Connecticut River (USA). Smith et al. (2002) observed that these populations have undergone physiological and ecological adaptations and can now survive in soft water with low alkalinity. This invasive species now fills the open niche for a benthic colonial predator. This filling of the open niches by invaders in estuarine habitats free of freshwater species is also hypothized by Nehring (2006).
Amphipods and molluscs are the most frequently studied species group of freshwater taxa in the papers reviewed (Table 1). Of the crustaceans, studies on D. villosus, followed by Chelicorophium curvispinum and Echinogammarus spp., dominate. D. polymorpha is the most studied freshwater mollusc. This species is very well known and widely distributed with a long history of invasion in Europe and North America, and has been included in the list of “100 of the world’s worst invasive alien species” (European aliens 2009). The same is valid for E. sinensis, D. villosus and C. fluminea. There is no doubt that, at present, crustaceans are of greatest interest among freshwater macro-invertebrates in Europe, as some of them change the indigenous communities and food webs radically (van Riel et al. 2006a, b, 2007; Kelly and Dick 2005; Van den Brink et al. 1993; Paffen et al. 1994). For instance, crayfish occupy a keystone position in the trophic web of the invaded system and interact strongly with various trophic levels. Due to their predatory and grazing activities they often reduce food web complexity and structure, as their feeding on detritus opens the detritic food chains to higher trophic levels (Geiger et al. 2005).
Competition (especially predation), species replacement or effects of invasion and filtering impacts are the subjects most commonly addressed in the literature (see Table 2). The latter is included mainly due to the high number of papers dealing with the effects of Dreissena. Other questions, for example coexistence, tolerance, habitat or water quality, life history or traits and introduction of diseases were treated less frequently. Only few papers discussed the genetic consequences of their introduction (Therriault et al. 2005; Mueller 2001).
Invaders in water quality assessment
Assessments regarding the effect of exotic aquatic species follow different aims and definitions. Ashton et al. (2006), for example, understand the term as a survey of invading species recorded, while others focus on the ecological risks and their prediction (e.g. Ricciardi and Kipp 2008; Gollasch and Leppäkoski 2007; Claudi and Ravishankar 2006) and consequences for policy (Simberloff 2005). Further studies or procedures deal with the description of the ecological status of water bodies based on a standard or index and the problems of incorporation of invaders in assessments of national or EU level (e.g. Arbačiauskas et al. 2008; Gabriels et al. 2005; Friedrich and Herbst 2004; ECOPROF 2007; DIN 2004; ÖNORM 1997; Cardoso and Free 2008). Inclusion or exclusion of neozoa can have significant effect in assessments and ecological studies. Gabriels et al. (2005) found that inclusion or exclusion of Corbicula and Dreissena species led to different assessments applying the Belgian Sediment Index (BSI). Earlier, Gayraud et al. (2003) elaborated that, for large river systems, exclusion or inclusion of invaders caused significantly different results for community descriptions based on raw abundances. However, the exclusion had little or no effect when using ln-transformed or presence/absence data. The authors concluded that the inclusion of neozoa should improve the detection of human impacts on invertebrate communities and suggest a method for dealing with aliens similar to some fish indices (e.g. Belpaire et al. 2000) using the proportion of alien individuals or alien species in the community. Identifications on genus or even family level may be sufficient for the description of the functional structure of invertebrate communities of large rivers. Recently, Arbačiauskas et al. (2008) followed the same idea and tested a simple index correlated to BMWP (Biomonitoring Working Party), which is a metric frequently used for ecological quality assessment, using different taxonomic levels.
Gabriels et al. (2005) recommended to include the alien species in the standard indicator list of the Belgian Biotic Index (BBI), because they are part of the local community reflecting diversity, despite their danger for the indigenous fauna. However, no tolerance classes were aligned to the invaders. So, the species have effects on the BBI only through number of taxa, not for their ability to resist habitat or water quality deterioration. In present assessments, species of the non-indigenous families Cambaridae (Orconectes limosus or Procambarus clarkii) or Grapsidae (E. sinensis) are, for example, not specified with a tolerance level for a certain type of pollution, but are taken into account in the total number of taxa and are therefore incorporated into the BBI and BSI (Belgian Sediment Index) indices. Some (e.g. species of Dreissena and Potamopyrgus) are incorporated on species level with tolerance levels, while others are just counted as part of the taxonomic unit (e.g. on family level), to which a tolerance level is assigned (De Pauw and Heylen 2001). This is in part similar to the present method used in Germany, the Standard for Water Assessment (Saprobic Index) (DIN 2004), which works preferably on species level. There, 22 non-native species are included in the list of indicators for saproby. However, only ten of them are specified with an indicator value of tolerance, as only a defined tolerance is known and can contribute to the procedure for these species. The water assessment practice in Austria follows the same principle (ECOPROF 2007). However, in the Netherlands, invaders are excluded from water quality assessments highlighting the fact that various countries deal with invaders differently, mainly due to different assessment systems and concepts. The general problem is that most of the species recorded as non-indigenous in the last years are in the phase of invasion and do not yet show a clear saprobic tolerance. Friedrich and Herbst (2004) stated that at least 10 or more years would be necessary in order to detect the saprobic tolerance of a non-indigeniuos species after invasion. Later, when a certain tolerance level or range can be estimated from studies and observations, these species can be included in the assessment systems as classified organisms. Cardoso and Free (2008) suggested a way to consider the invaders in the implementation of the European water framework directive (WFD) by matching the abundances and distribution to the five quality levels following the classification in the WFD. However, the critical class values for each species still have to be defined by experts. This method should be performed separately from the other ecological assessments required by the WFD and shall supply the results from the latter. Arbačiauskas et al. (2008) classified invading species according to their levels of “biocontamination” and calculated the ratio of alien to native taxa. However, the approach aims to work on order level, which may lower the accuracy and robustness of the results, as some invading species are from the same order or even family as indigenous species (e.g. Dikerogammarus spp. and indigenous Gammarus spp. from family Gammaridae). The determination of a “biopollution level” is presented by Olenin et al. (2007) and is rather a sort of decision support system than an index-based assessment. This uses the value of typical local impact of each exotic species on the levels of community, habitat and ecological functioning. Although developed and tested in coastal waters, the application should also be possible in freshwaters. As this promising assessment tool is scaled in five classes, it conforms with the WFD.
An approach by Leung et al. (2005) gives a more theoretical mathematical frame, which is aimed to reduce biological and economical complexity to enable policy makers and managers to base their decisions of management and application of measures on rapid assessments. This approach can start, when more basic questions are solved (e.g. how to assess the invasion properly, estimate the value of an ecosystem after invasion etc,).
The examples given above show that the tolerance classification of invader species and their treatment in water management assessments is different in countries and conception. Some taxa are included on species level, others on family or genus level, whereas some are classified according to their tolerance and others not. There is no apparent consensus of simple and uniform “inclusion” or “exclusion” from the procedures at present.
Background, strategies and effect of invasion
Decrease or extinction of indigenous species populations
Significant decrease or extinction of indigenous species populations may be due to (1) a change of the habitat quality (mostly resulting from pollution) for native species, leaving an empty space for migrating tolerant species, (2) an invasion of a new species which takes over the niche of a native or preys on them successfully in some exceptional cases and (3) an exploitation of a ‘new’, previously unexploited food resource. For many estuaries of north-western Europe, invasion due to changes in habitat quality are most important. Faasse and van Moorsel (2003) state that the relatively high number of empty niches that exist in these brackish waters are the main reason why these waters are particularly susceptible to invasions of alien species. According to Wolff (1999), about twenty percent of the brackish macrobenthos species in Dutch estuaries were introduced by man.
After Amat et al. (2005), successful invasive species are usually characterised by their
-
high abundance in their original range or large native range
-
omnivorous nature (a wide feeding niche)
-
high genetic variability or phenotypic plasticity (rapid adaptation of ecology and life history characteristics)
-
short generation times and rapid population growth (r-strategists)
-
ability of fertilized females to colonise alone (single parent reproduction)
-
vegetative and asexual reproduction, self fertilization
-
larger size compared with most related species
-
high dispersal rate
-
association with human activities
-
ability to function in a wide range of physical conditions.
Statzner et al. (2008) showed in a statistical analysis that, for a series of biological traits that overlap in part with previous ones (e.g. reproduction cycles per year, reproduction strategy, aquatic dispersal), invasive taxa have significant advantages compared with natives. Invasions of closely related species often lead to niche competition between the invading and indigenous species. The outcome of this competition is partly determined by differences in physiological tolerance of the competing species to the environmental conditions of the colonised habitat (Wijnhoven et al. 2003).
Competition is, in general, a very important factor affecting the distribution and occurrence of indigenous species. For instance, D. polymorpha is known to attach itself to the top of other bivalves competing for food while filtration, which can lead to extirpation of the indigenous unionids (Burlakova et al. 2000; Nalepa 1994; Ricciardi et al. 1997). Thus, local food depletion and/or increased metabolic costs for the competing species may result in starvation of the native (Baker and Hornbach 1997). Filtration of the larval individuals of indigenous species by invasive species can also result in a reproductive threat (D. polymorpha, C. gigas and others). Mörtl and Rothhaupt (2003) demonstrated a lower recruitment of native macroinvertebrates in the presence of D. polymorpha, which could be explained by ingestion of larvae and competition for food by D. polymorpha. The introduction of neozoic species could therefore drastically alter life community structure and biodiversity by critically affecting the fitness of indigenous community members. In several cases, the occurrence of invasive species led to a domination of non-indigenous populations or a severe change in the macrobenthic community (Gumuliauskaite and Arbaciauskas 2008; Eckmann et al. 2008; Pilollo et al. 2008; Packalén et al. 2008; van Riel et al. 2006a, b; Paffen et al. 1994; van den Brink et al. 1993), frequently due to the lack of natural controls (Amat et al. 2005; Cinar et al. 2005; Haynes et al. 2005; Karatayev et al. 1997, 2003; Paffen et al. 1994; Ricciardi et al. 1996; Schloesser et al. 1998). Elminius modestus, for instance, became a dominating species at sites subject to freshwater influence, resulting in a complete replacement of all other autochthonous barnacles (Lawson et al. 2004). Occhipinti-Ambrogi and Savini (2003) stated that every healthy community has a natural impediment to bio-invasion. At sites lacking this natural contrasting force, the alien species have advantages to out-compete native ones successfully.
Predation is one of the most effective advantages in competing for species establishment in a colonised water (Dick and Platvoet 2000; Dick et al. 2002; Kinzler and Maier 2003). Therefore, some authors focus on the life history and biological traits of those species (Devin et al. 2004; Kley and Maier 2003; Piscart et al. 2003; Smith et al. 2002). However, establishment of a new dominating species in the community does not always result in a stable community structure. The macrobenthic community, which may be dominated by neozoic species, will most likely evolve to a new equilibrium state after initial peaks in abundance or biomass (Darrigran et al. 2003; Karatayev et al. 1997). Sometimes this could even result in a mass mortality of the neozoic species (Palacios et al. 2000) due to a variety of environmental or even biological events, leading to a co-existence with similar species from the same ecological guild. It has been demonstrated that, over the last 20 years in the lower Rhine, one dominating invading crustacean species followed the other, with each displaying a peak of mass development at a certain time (van Riel et al. 2006a; Bachmann et al. 2001; Ricciardi and Maclsaac 2000; Van den Brink et al. 1993; van der Velde et al. 1994). Out of these, D. villosus is considered to be one species that affects the community and the food web considerably more than the other species. Earlier, C. curvispinum was reported to have major impact (van Riel et al. 2006a; Van den Brink et al. 1993). Sometimes, the impact of neozoa is not only induced by competition or predation of living animals. Cherry et al. (2005) observed that Asian clam (C. fluminea) die-offs have the potential to exceed acute levels of NH3-N for at least some species of autochthonous unionid mussels, which could also result in community changes.
Co-invasion
Another result of neozoa invasion is their ability to introduce exotic diseases and parasites. During colonisation, the pressure of selection imposed on the parasites of the invader generally leads to their extinction, especially when the parasites need several successive hosts for completing their life cycle (e.g. trematodes). Consequently, only a few species of parasites succeed in adapting to the new environmental conditions. Gérard and Le Lannic (2003) discovered, for the first time in Europe, the occurrence of Sanguinicola sp. in the fresh and brackish water snail P. antipodarum, although this blood fluke of fish was previously never recorded in this prosobranch. Molloy et al. (1996) surveyed the parasites of zebra mussels and found mussels with trematode infections (Aspidogaster, Phyllodistomum and Bucephalus polymorphus) and ciliates (order Hymenostomatida). In this study, the authors did not describe whether these parasites could be transferred to populations of native bivalves, however, there are numerous cases described in which exotic species introduced new organisms such as diseases to sensitive native species. It is well known that the fungus Aphanomyces astaci, which is highly infective and disastrous to European crayfish populations, was introduced with the resistent American species Pacifastacus leniusculus, P. clarkii and O. limosus. P. clarkii could, apparently, transmit diseases even to humans, since it was identified as a mechanical transmitter in an outbreak of tularemia caused by the bacterium Franciscella tularensis (Geiger et al. 2005). The Chinese mitten crab (E. sinensis) is the second intermediate host of the oriental lung fluke (Paragonimus westermanii). Recently, a new reovirus was characterized in E. sinensis which may represent a new genus of Reoviridae, different from the other crab reoviruses (Zhang et al. 2004).
Coexistence
Invasion does not always result in extinction or domination of the native populations. Coexistence, or a balance either between colonizers and natives or among colonizers, was described in several papers (Bachmann et al. 2001; Cope and Winterbourn 2004; MacNeil et al. 2001a, b, 2003). The slipper limpet (C. fornicata), for instance, did not significantly affect oyster growth or even the zoobenthic community following colonization (de Montaudouin et al. 1999). Moreover, Schreiber et al. (2002) showed in a field experiment that the introduction and establishment of the non-indigenous P. antipodarum may even cause positive effects on the biomass of the native fauna. However, the studies of Kerans et al. (2005) could not confirm these findings in the field studies. It was shown by Aberle et al. (2005) that inter-specific competition between grazers was diminished by a shift in feeding preference and a potential divergence of trophic niches when species coexist. A similar effect of decreasing competition or balancing between invader and native is described by MacNeil et al. (2003). The authors showed, in Irish freshwaters, that infection of both the exotic Gammarus pulex and the indigenous G. duebeni celticus with the acanthocephalan Echinorynchus truttae reduced predation of the invader on the native and, thus, supports co-existence.
Co-existence, where native species from the same ecological guild are not outnumbered by a small niche overlap of invader species, is observed also in macroinvertebrates, as well as in higher trophic levels (Bachelet et al. 2004). Nevertheless, minor negative impacts on the macro-fauna could occur: It seems that the presence of C. fornicata could reduce survival and growth of blue mussels Mytilus edulis (Thieltges 2005; Thieltges et al. 2006). This underlines the need for a species-by-species approach in assessing impacts of introduced species. Although co-existence between guild-like macro-invertebrates can occur, it is still possible that the neozoon has a negative effect on other organisms. C. fornicata has such a negative impact on habitat suitability for juvenile sole because of some structural changes to the benthic habitat (Le Pape et al. 2004).
The establishment of a neozoon in a co-existence situation often depends not only on habitat quality, but also on the success of other competing invaders. Cope and Winterbourn (2004) reported about the mutual influence of two invasive snails, while Diggins et al. (2004) studied habitat selection of two invasive mussels. Kley and Maier (2003) investigated the balance between D. villosus and Echinogammarus ischnus. Fitness was addressed by Mueller (2001).
Amat et al. (2005) demonstrated that one invading species (Artemia sp.) can co-occur with the autochtonous species. However, in other regions, A. franciscana dominated and rapidly replaced A. salinus, most likely due to differences in environmental conditions. Although in some cases the difference between co-existence and complete domination is not very great, for other neozoa, the term ‘co-existence’ can not be used in such a dramatic context. Aside from those species which alter the structure of the native community by a successful invasion, there are species that are recorded in low abundances and are expected to have little or no effect on the native ecosystem and community, as far as it is known, for example occasional records of Dendrocoelum romanudanubiale (Turbellaria: Plathelminthes), Atyaephyra desmaresti (Decapoda: Crustacea) and Hemimysis anomala (Mysidacea: Crustacea) are described (Reeze et al. 2005). However, this may reflect a temporal situation, as exotic species can occur in small populations for a certain time period before exploding and causing heavy effect on the native community (Crooks 2005). Diederich et al. (2005) documented a low spread of the introduced Pacific oyster C. gigas in the German Wadden Sea for years, which can be interpreted as an intermediate co-existence, as the authors expect a much faster and more extensive growth and impact on indigenous species following higher water temperatures due to climate change. The authors report that this case was observed in New Zealand, when a marked increase of temperature was thought to be responsible for a rapid spread and growth superseding the native rock oyster Saccostrea glomerata after 7 years of the first wild record of C. gigas.
Effects on the food web
Phelps (1994) showed that the new establishment of C. fluminea had an impact on the entire ecosystem, resulting in an increase in submerged aquatic vegetation as well as increasing fish and even bird populations. Zebra mussel invasion changed the importance of the roles of benthic animals such as suspension feeders and sediment mixers (Strayer and Smith 2001), resulting in different impacts on higher trophic levels. Additionally, Branch and Steffani (2004) demonstrated effects on the food web, showing how a higher trophic level (birds) may profit from colonization of marine Mytilus mussels (as invaders), while others (crabs) were affected by mass mortality. Even on a more local scale, it is sometimes apparent that an invader can displace certain species from the same guild, while conditions for other benthic organisms (native as well as invasive) improve (Dermott and Kerec 1997; Ricciardi et al. 1997; Strayer and Smith 2001). Several authors demonstrated that the presence of Dreissena spp. initially resulted in increased population densities, biomasses and macro-invertebrate diversity, coinciding with an increased habitat complexity (Horvath et al. 1999; Kuhns and Berg 1999; Stewart et al. 1998) However, after a period of 3 years, the effects of Dreissena on other organisms generally changed, leading macro-benthos communities back towards pre-Dreissena conditions (Haynes et al. 1999).
The prediction of the effects of invaders on the indigenous community is challenging. If zebra mussels, for instance, provided a refuge for macro-zoobenthos against predation of fish (Dieterich et al. 2004), effects on a higher trophic level might be observed. Johannsson et al. (2000), for example, predicted that the benthic food chain could potentially support more biomass of fish than the pelagic food chain following introduction of Dreissena ssp. These predictions are in harmony with findings of Strayer et al. (2004); Karatayev and Burlakova (1995) and Karatayev et al. (1997), reporting an increase of the biomass of benthophage fish after zebra mussel invasion. The studies showed that the invasion of D. polymorpha had a negative impact on open water fish species, but populations of littoral fish species prospered from the invasion. The studies suggest a major shift in food web structure. Moreover, Kas’yanov and Izyumov (1995) showed that roach (Rutilus rutilus) even changed from a herbivorous feeding pattern towards consumption of molluscs after Dreissena introduction. In contrast, Trometer and Busch (1999) found no significant differences in age-0 growth for the fish species. They concluded that either the zooplankton abundance was still sufficient, or that fish may hatch earlier or rely more on other prey types, especially the more abundant benthic invertebrates (Kelleher et al. 1998, 2000). In general, assessing the predictive power of food web studies including the high dynamics of invasions, mass development and breakdown of an invader population, is difficult, as unknown key factors may not be considered. For example, the acanthocepalan Pomphorhynchus sp., which may be easily overlooked in food web studies, infected C. curvispinum from the River Rhine and may contribute to control and observed decrease the population of the crustacean (van Riel et al. 2003). At present, prediction of the invasiveness and impact of a single species may be more realistic through developing empirical models based on a well-studied invasion history (Ricciardi 2003).
Biological traits
D. villosus may be one of the best studied species in some regions (Piscart et al. 2003; Kley and Maier 2003; MacNeil et al. 2001a, b). Some papers also deal with the life cycle of invaders, such as C. fluminea (Rajagopal et al. 2000; Mouthon 2001a, b) and the golden mussel Xenostrobus securis (syn. Limnoperna fortunei) (Darrigran et al. 1999, 2003). Other papers deal with the biological traits of the brackish water mussel M. leucophaeata (Bamber and Taylor 2002), the life history and reproductive biology of C. curvispinum (Rajagopal et al. 1999), the longitudinal and temporal variations and demography of C. fluminea (Mouthon 2003) and the crab R. harrisii (Goncalves et al. 1995). Apparently, most attention was paid to D. polymorpha (an internet search yields a vast number of references). However, little is known of numerous other neozoa, apart from sole documentations of densities, individual numbers or biomass, distribution patterns in their new habitats and migration pathways.
With respect to geographical distribution and directions of colonization, the Ponto-Caspian region presents itself as one of the main sources for regionally revolving invasions in central European waters. However, some species were also successfully imported from Northern America (e.g. G. tigrinus, Crangonyx pseudogracilis, Pectinatella magnifica, R. harrisii, Physella acuta, Dugesia tigrina, O. limosus). The central European species (G. pulex) presents itself as an invader in eastern and northern parts of Europe, causing changes of the native macro-invertebrate community, there (Kelly et al. 2003; MacNeil et al. 2001a, b, 2003).
Ecological studies on neozoa of central European waters were published irregularly and often in languages other than English, with many also out-of-date due to rapid distribution and new records. Fauna records were published either in lists of the agencies or in national papers. Despite the lack of availability and different languages, these studies may be of use for the reconstruction of the directions and speed of colonisation in the future. First steps to condense the scattered information have been made (DAISIE database and other platforms as mentioned in the Introduction), while some studies show that a powerful set of data enables use of the traits for an assessment of human impact (e.g. Gayraud et al. 2003) or a prediction of invasiveness (e.g. Statzner et al. 2008). Devin and Beisel (2007), however, conclude from their studies that not only biological but also ecological traits (e.g. salinity, flow velocity) are necessary for prediction of invasiveness.
Perspectives of application
Requirements for indicator species
In general, bio-indication must be based on “detailed analysis of the ecosystem components and their ecological functioning” (Bustos-Baez and Frid 2003). Consequently, species must fulfil several requirements to be useful indicators. In any case, the goal of the assessment influences the need to fulfill such requirements. Some are listed here
-
to be found in high abundance or frequency
-
to have an in situ response on changes of the ecosystem, with rapid changes in densities and/or biomasses
-
to cover a wide geographical area
-
to be determined accurately
-
to have an important and clear role in the trophic system
-
to be bound to the habitat, sedentary species with low dispersive capacities
-
to be allocated properly to a compartment of the ecosystem
-
to have a defined feeding strategy (e.g. no omnivory)
-
to have a continuous life cycle (e.g. no long diapause)
-
to have long or medium-long generations
-
to be one of the first phases in bio-accumulation.
Thus, the traits of the indicator organisms should be well known for a specific assessment (e.g. geographical distribution, feeding issues, reproduction, eco-physiology). However, frequently, only few species can fulfill the requirements, particularly for macro-invertebrates. In technical water assessments this problem is solved using life communities or species assemblages, in which different species compensate for the deficiencies of each other (with respect to the biological traits), resulting in a combination of the indicative traits.
Invaders as indicators?
Only the most dominating invasive species (e.g. D. villosus, D. polymorpha) fulfill the requirements to be an indicator for a certain aim of the assessment (e.g. pollution from industrial activities). Those are the only species, from which the life history and biological traits have been studied sufficiently at this stage. These abundant species are of high importance for understanding the main character and functioning of the community. Moreover, tolerances to certain pollutants are known. Thus, it does not seem advisable to base the indication of possible ecosystem changes on less known species, for which little more than, for example, mass development in an invaded habitat is recorded. However, any successful invasion by an exotic species indicates that something has changed in the use of system resources. Disturbed environments, for example strongly degraded or polluted environments, are especially susceptible to invasions (Occhipinti-Ambrogi and Savini 2003) and in these cases, each invading species is a good indicator species.
Of the species discussed in the papers reviewed, the abundant neozoan species in the Central European region generally fulfill some of the criteria above for use as indicators for pollution (e.g. D. villosus, Ch. curvispinum, D. tigrina, Jaera istri; see DIN 38410), although criteria on habitat bondage, defined feeding strategies and medium or long generations have still to be discussed. Other, more resilient species (e.g. O. limosus), would not fulfill the criteria from above. For example, they are weak indicators for special and rare habitat conditions, but better indicators for more widespread and common conditions (for example, after reduction of site-specific morphological diversity). However, a resilient species could also be sensitive for other conditions such as acidity or organic pollution. In general, a species can be used in assessments when it can be classified to certain tolerances according to its traits, no matter whether it is exotic or indigenous.
Alien species with presently or consistently small populations do not play the same role in the food web as the abundant ones and furthermore, even less detailed knowledge on their ecology is available. Therefore, the rare and less abundant neozoa do not seem to be appropriate as useful indicators for human impacts, but are still applicable for biodiversity indicators. Even small changes in taxonomic composition may indicate minor shifts of ecological settings and may serve as a sensitive early warning system, even if changes in the ecosystem functions cannot be detected at present. However, in future, neozoa with small populations may become dominant, for example due to climate change, as expected for C. gigas (Diederich et al. 2005).
Inclusion or exclusion from community assessments and analysis?
With regard to the question of how to deal with invaders in assessments, Reeze et al. (2005) discussed a list of options:
-
to exclude the neozoa (leads to an assessment based on native species only);
-
to move towards log-transformed data rather than normal scales (to diminish effect of invasive species);
-
to assign invasive species depending on their impact;
-
to exclude biotopes colonised mainly by invaders from the assessment;
-
to connect reliability of assessment to new metric indicating the impact of invasive species to introduce a metric based on other characteristics (i.e. diversity, functional groups).
Thus, there are two possible different points of view and arguments: pro exclusion and pro inclusion:
Pro exclusion
The communities affected by invaders may be not stable enough for establishing an indicator-based assessment system resulting from, for example, the dynamic replacement of natives by invading species. This includes the risk that, by only counting the remaining indigenous species and their abundances, only a too small part of the ecosystem is considered in order to infer any reliable changes in communities and functions caused by human impacts such as organic loads or xenobiotic contaminations.
Furthermore, in the case that the ecological traits of a dominant non-indigenous species are not known or imprecise, it would be difficult to achieve reasonable results in food web and community analysis, as well as in assessments based on ecological guilds. Van den Brink et al. (1993) stated that high individual numbers of an invader might change the food web, but whether this could also have happened with an indigenous species is unclear. The exclusion of neozoa, however, could reduce incalculable dynamics, (e.g. exponential increase of population and following breakdown within a short time period) and, thus, uncertainties of interpretation are diminished.
Pro inclusion
The inclusion of invasive species has the advantage of being able to analyse the functions of the whole ecosystem represented by the macroinvertebrates. Regarding ecological guilds of the community rather than species would be a first approach for an understanding of ecosystem functions. It can be expected that the guilds correspond to habitat conditions and changes to these conditions. This should also be indicated by invaders, as far as the traits of the occurring species are known or it is classified, although it cannot be excluded that the taxonomic community may change during the course of a study due to colonisation dynamics.
Even if little is known about the species ecology, enough is known about the most abundant neozoa (about the feeding type, dwelling type etc.,) to enable an allocation to ecological guilds and to be useful for ecological evaluation. The studies of Kinzler and Maier (2003) suggest that the function of the guild persists, while species may be replaced within the guild. Thus, for the assessment of ecological functioning, it would be of little importance, which species—native or invader—represents the life community. In this case, there would be no benefit to exclude an invader species, particularly if it developed a large population. Consequently, the German Water Assessment Standard “DIN 38410” (Water Assessment based on the Saprobic Index) includes neozoa, as they are dominant in communities of the Rivers Rhine, Danube and Elbe, where they are classified in their tolerance to saprobic conditions and contribute to the water quality assessment. As well as ecological functions, the taxonomic composition of a changing system may shift and thus, indicate changes where the functions of the system may not be affected. In this respect, the taxonomic community can serve as an early warning system. But replacement of an indigenous species by another, especially by a neozoan, does not necessarily mean a change of the settings, but may also be a consequence of the aggressive extension process of the invading species (as observed for D. villosus). Thus, the taxonomic community shows the dynamics and status of invasion and might also indicate an ending point of an invasion process. In this case, when biodiversity is assessed, the inclusion of the exotic species in community studies is clearly advisable.
Conclusions: adopting neozoan species as indicators
A clear tendency has been identified from the literature reviewed: neozoic macro-invertebrates are colonising brackish and freshwater water systems not only in central Europe but throughout the world, e.g. St. Lawrence river, Canada, rivers in the Greater Yellowstone Area, Lake Ontario, Lake George, USA, river systems in southern Spain (Ebro), Ukraine (Pripyat) or Iraq (Shatt Al Basrah Canal) or the Baltic Sea (Ricciardi and Whoriskey 2004; de Lafontaine et al. 2008; Hall et al. 2006; Haynes et al. 2005; Frischer et al. 2005; Lalaguna and Marco 2008; Semenchenko and Vezhnovetz 2008; Semenchenko and Laenko 2008; Alexandrov et al. 2007; Clark et al. 2006; Kube et al. 2007; Laine and Mattila 2006). This is a fact and this process cannot be halted. Invaders are expected to be established as a prominent part of the communities of European rivers and lake in the near future and “we should be aware of new species introductions” (Gollasch and Nehring 2006). Taking this into account, it appears that integration of these species into ecological studies and assessments is the only alternative. However, the approach used and extent of inclusion has to be linked to the aim of the assessment.
One feasible way to integrate the invaders in water management assessments is outlined in the following and illustrated in Fig. 1 with respect to the apparently complex situation.
Concept of integrated assessment with invaders. The elements contribute to the assessment of the ecological status. When “human impact” assessment is not possible, due to low number or abundance of tolerance-classified species, only “biodiversity” is available for the determination of the ecological status (see text for further explanations)
In most questions of water management or nature conservation, the ‘ecological status’ is the descriptor for measuring changes. ‘Ecological status’ can be described roughly by (1) biodiversity of a water type (“assessment of invaders or invasion”; indicated by e.g. species richness, dominance, metrics of ecological guilds and other traits) and/or (2) human impact on the system (“assessment with invaders”; frequently measured with species classified according their tolerances to certain stressors, e.g. saprobity, increased salinity, temperature, EC50 values of certain pollutants). In the first case (biodiversity) it appears advisable to include all species, native and non-indigenous, occurring at the sites of interest in the assessment. Analysis of the biodiversity of a water body can document and measure the effect of invading species on the local community and system functioning. A promising method in line with the requirements of the European Water Framework Directive is presented by Olenin et al. (2007), but other tools may also be appropriate (e.g. species richness measures). In the second case (measuring human impact), exotic species can be integrated in differentiated steps, at least for assessment systems that are based on the classification of indicators (e.g. index of saprobity, Belgian Biotic Index). In the first step of the procedure, only those invaders that are definitely classified with a certain tolerance level or value are integrated. Each taxon without classification is excluded from further processing, until a certain tolerance value is known and assigned to it so that it can be used in the index-based system. In this respect, alien species are treated in the same way as any other native species and the assessment should lead to a plausible result. This practice is applied presently in Austria, Germany and Belgium. However, if the abundance or the species number of all classified species from a study site is too low for a correct calculation, the assessment of human impact with macroinvertebrates is not possible, leaving only the method of biodiversity analysis (see above), including all invaders, for a description of the ‘ecological status’ (Fig. 1).
The need for further knowledge is obvious. It is necessary to elaborate and indentify the power of all important invaders for indication more precisely, in order to keep indicator systems (e.g. standards for human impact assessment) valid for the future. Careful studies of the recent knowledge on invader ecology derived from field or laboratory studies and literature is required in order to (1) adjust and assign ecological profiles (biological traits) of the non-indigenous species currently, and (2) to assess their effects on native communities, especially at the early phase of colonisation.
References
Aberle N, Hillebrand H, Grey J, Wiltshire KH (2005) Selectivity and competitive interactions between two benthic invertebrate grazers (Asellus aquaticus and Potamopyrgus antipodarum): an experimental study using C-13- and N-15-labelled diatoms. Freshw Biol 50(2):369–379. doi:10.1007/b95175
Alexandrov B, Boltachev A, Kharchenko T, Lyashenko A, Son M, Tsarenko P, Zhukinsky V (2007) Trends of aquatic alien species invasions in Ukraine. Aquat Invasions 2(3):215–242. doi:10.3391/ai.2007.2.3.8
Amat F, Hontoria F, Ruiz O, Green AJ, Hortas F, Figuerola J, Hortas F (2005) The American brine shrimp as an exotic invasive species in the western Mediterranean. Biol Invasions 7(1):37–47. doi:10.1007/s10530-004-9634-9
Aquatic aliens (2009) Aquatic alien species in German inland and coastal waters. www.aquatic-aliens.de. Cited 05 Jan 2009
Arbačiauskas K, Semenchenko V, Grabowski M, Leuven RSEW, Paunović M, Son MO, Csányi B, Gumuliauskaitė S, Konopacka A, Nehring S, van der Velde G, Vezhnovetz V, Panov VE (2008) Assessment of biocontamination of benthic macroinvertebrate communities in European inland waterways. Aquat Invasions 3(2):211–230. doi:10.3391/ai.2008.3.2.12
Ashton G, Boos K, Shucksmith R, Cook E (2006) Rapid assessment of the distribution of marine non-native species in marinas in Scotland. Aquat Invasions 1(4):209–213. doi:10.1007/3-540-30023-6
Bachelet G, Simon-Bouhet B, Desclaux C, Garcia-Meunier P, Mairesse G, de Montaudouin X, Raigne H, Randriambao K, Sauriau PG, Viard F (2004) Invasion of the eastern Bay of Biscay by the nassariid gastropod Cyclope neritea: origin and effects on resident fauna. Mar Ecol Prog Ser 276:147–159. doi:10.3354/meps276147
Bachmann V, Beisel JN, Usseglio-Polatera P, Moreteau JC (2001) Decline of Dreissena polymorpha in the River Moselle: biotic and abiotic key factors involved in dynamics of invasive species. Arch Hydrobiol 151(2):263–281
Baker SM, Hornbach DJ (1997) Acute physiological effects of zebra mussel (Dreissena polymorpha) infestation on two unionid mussels, Actinonaias ligamentina and Amblema plicata. Can J Fish Aquat Sci 54(3):512–519. doi:10.1139/cjfas-54-3-512
Baltic Sea Alien Species Database (2009) http://www.corpi.ku.lt/nemo/. Cited 05 Jan 2009)
Bamber RN, Taylor JD (2002) The brackish water mussel Mytilopsis leucophaeata (Conrad, 1831) (Bivalvia: Dreissenidae) in the River Thames. J Conchol 37:403–404
Barbiero RP, Tuchman ML (2004) Long-term dreissenid impacts on water clarity in Lake Erie. J Gt Lakes Res 30(4):557–565
Belpaire C, Smolders R, Auweele IV, Ercken D, Breine J, Van Thuyne G, Ollevier F (2000) An index of biotic integrity characterizing fish populations and the ecological quality of Flandrian water bodies. Hydrobiologia 434(1–3):17–33. doi:10.1023/A:1004026121254
Bij de Vaate A, Jazdzewski K, Ketelaars HAM, Gollasch S, Van der Velde G (2002) Geographical patterns in range extension of Ponto-Caspian macroinvertebrate species in Europe. Can J Fish Aquat Sci 59(7):1159–1174. doi:10.1139/f02-098
Branch GM, Steffani CN (2004) Can we predict the effects of alien species? A case-history of the invasion of South Africa by Mytilus galloprovincialis (Lamarck). J Exp Mar Biol Ecol 300(1–2):189–215. doi:10.1016/j.jembe.2003.12.007
Brooks SJ, Platvoet D, Mills CL (2008) Cation regulation and alteration of water permeability in the amphipod Dikerogammarus villosus: an indicator of invasion potential. Fundam Appl Limnol 172(3):183–189. doi:10.1127/1863-9135/2008/0172-0183
Bruijs MCM, Kelleher B, van der Velde G, de Vaate AB (2001) Oxygen consumption, temperature and salinity tolerance of the invasive amphipod Dikerogammarus villosus: indicators of further dispersal via ballast water transport. Arch Hydrobiol 152(4):633–646
Budd JW, Drummer TD, Nalepa TF, Fahnenstiel GL (2001) Remote sensing of biotic effects: zebra mussels (Dreissena polymorpha) influence on water clarity in Saginaw Bay, Lake Huron. Limnol Oceanogr 46(2):213–223
Burlakova LE, Karatayev AY, Padilla DK (2000) The impact of Dreissena polymorpha (PALLAS) invasion on unionid bivalves. Int Rev Hydrobiol 85(5–6):529–541
Bustos-Baez S, Frid C (2003) Using indicator species to assess the state of macrobenthic communities. Hydrobiologia 496(1–3):299–309. doi:10.1023/A:1026169520547
Cardoso AC, Free G (2008) Incorporating invasive alien species into ecological assessment in the context of the water framework directive. Aquat Invasions 3(4):361–366. doi:10.3391/ai.2008.3.4.1
Cherry DS, Scheller JL, Cooper NL, Bidwell JR (2005) Potential effects of Asian clam (Corbicula fluminea) die-offs on native freshwater mussels (Unionidae) I: water-column ammonia levels and ammonia toxicity. J N Am Benthol Soc 24(2):369–380. doi:10.1899/04-073.1
Cinar ME, Ergen Z, Dagli E, Petersen ME (2005) Alien species of spionid polychaetcs (Streblospio gynobranchiata and Polydora cornuta) in Izmir Bay, eastern Mediterranean. J Mar Biol Assoc UK 85(4):821–827. doi:10.1017/S0025315405011768
Clark PF, Abdul-Sahib IM, Al-Asadi MS (2006) The first record of Eriocheir sinensis H. Milne Edwards, 1853 (Crustacea: Brachyura: Varunidae) from the Basrah Area of Southern Iraq. Aquat Invasions 1(2):51–54. doi:10.1007/3-540-30023-6
Claudi R, Ravishankar TJ (2006) Quantification of risks of alien species introductions associated with ballast water discharge in the Gulf of St. Lawrence. Biol Invasions 8(1):25–44. doi:10.1007/s10530-005-0234-0
Cope NJ, Winterbourn MJ (2004) Competitive interactions between two successful molluscan invaders of freshwaters: an experimental study. Aquat Ecol 38(1):83–91. doi:10.1023/B:AECO.0000021018.20945.9d
Crooks JA (2005) Lag times and exotic species: the ecology and management of biological invasions in slow-motion. Ecoscience 12(3):316–329. doi:10.2980/i1195-6860-12-3-316.1
Darrigran G, Penchaszadeh P, Damborenea MC (1999) The reproductive cycle of Limnoperna fortunei (Dunker, 1857) (Mytilidae) from a neotropical temperate locality. J Shellfish Res 18(2):361–365
Darrigran G, Damborenea C, Penchaszadeh P, Taraborelli C (2003) Adjustments of Limnoperna fortunei (Bivalvia: Mytilidae) after ten years of invasion in the Americas. J Shellfish Res 22(1):141–146
de Lafontaine Y, Sévigny JM, Calvé R, Verreault G, Despatie SP, Veilleux É (2008) Chinese mitten crabs (Eriocheir sinensis) in the St. Lawrence River and estuary, Canada: new records and risk of invasion. Aquat Invasions 3(2):153–163. doi:10.3391/ai.2008.3.2.5
de Montaudouin X, Audemard C, Labourg PJ (1999) Does the slipper limpet (Crepidula fornicata, L.) impair oyster growth and zoobenthos biodiversity? A revisited hypothesis. J Exp Mar Biol Ecol 235(1):105–124. doi:10.1016/S0022-0981(98)00167-1
De Pauw N, Heylen S (2001) Biotic index for sediment quality assessment of watercourses in Flanders, Belgium. Aquat Ecol 35(2):121–133. doi:10.1023/A:1011478427152
Dermott R, Kerec D (1997) Changes to the deepwater benthos of eastern Lake Erie since the invasion of Dreissena: 1979–1993. Can J Fish Aquat Sci 54(4):922–930. doi:10.1139/cjfas-54-4-922
Devin S, Beisel JN (2007) Biological and ecological characteristics of invasive species: a gammarid study. Biol Invasions 9(1):13–24. doi:10.1007/s10530-006-9001-0
Devin S, Piscart C, Beisel JN, Moreteau JC (2004) Life history traits of the invader Dikerogammarus villosus (Crustacea: Amphipoda) in the Moselle River, France. Int Rev Hydrobiol 89(1):21–34. doi:10.1002/iroh.200310667
Devin S, Beisel JN, Usseglio-Polatera P, Moreteau JC (2005) Changes in functional biodiversity in an invaded freshwater ecosystem: the Moselle River. Hydrobiologia 542(1):113–120. doi:10.1007/s10750-004-8771-6
Dick JTA, Platvoet D (2000) Invading predatory crustacean Dikerogammarus villosus eliminates bath native and exotic species. Proc R Soc Lond B Biol Sci 267(1447):977–983. doi:10.1098/rspb.2000.1099
Dick JTA, Platvoet D, Kelly DW (2002) Predatory impact of the freshwater invader Dikerogammarus villosus (Crustacea: Amphipoda). Can J Fish Aquat Sci 59(6):1078–1084. doi:10.1139/f02-074
Diederich S, Nehls G, van Beusekom JEE, Reise K (2005) Introduced Pacific oysters (Crassostrea gigas) in the northern Wadden Sea: invasion accelerated by warm summers? Helgol Mar Res 59(2):97–106. doi:10.1007/s10152-004-0195-1
Dieterich A, Mörtl M, Eckmann R (2004) The effects of zebra mussels (Dreissena polymorpha) on the foraging success of Eurasian perch (Perca fluviatilis) and ruffe (Gymnocephalus cernuus). Int Rev Hydrobiol 89(3):229–237. doi:10.1002/iroh.200310693
Diggins TP, Weimer M, Stewart KM, Baier RE, Meyer AE, Forsberg RF, Goehle MA (2004) Epiphytic refugium: are two species of invading freshwater bivalves partitioning spatial resources? Biol Invasions 6(1):83–88. doi:10.1023/B:BINV.0000010124.00582.d3
DIN (2004) DIN 38410-1 Deutsche Einheitsverfahren zur Wasser-, Abwasser- und Schlammuntersuchung (Biologisch-oekologische Gewaesseruntersuchung (Gruppe M). Bestimmung des Saprobieindex in Fliessgewsessern (M1). [German standard methods for the examination of water, waste water and sludge—Biological-ecological analysis of water (group M)—Part 1: determination of the saprobic index in running waters (M 1)] Beuth-Verlag Berlin 2004
Eckmann R, Mörtl M, Baumgaertner D, Berron C, Fischer P, Schleuter D, Weber A (2008) Consumption of amphipods by littoral fish after the replacement of native Gammarus roeseli by invasive Dikerogammarus villosus in Lake Constance. Aquat Invasions 3(2):187–191. doi:10.3391/ai.2008.3.2.9
ECOPROF (2007) Evaluation of aquatic biological data. Version 3.0. www.ecoprof.at. Cited 01 Feb 2009
Elliott M (2003) Biological pollutants and biological pollution—an increasing cause for concern. Mar Pollut Bull 46(3):275–280. doi:10.1016/S0025-326X(02)00423-X
European aliens (2009) http://www.europe-aliens.org/speciesTheWorst.do. Cited 05 Jan 2009
Faasse M, van Moorsel G (2003) The North-American amphipods, Melita nitida (Smith, 1873) and Incisocalliope aestuarius (Watling and Maurer, 1973) (Crustacea: Amphipoda: Gammaridae), introduced to the Western Scheldt estuary (The Netherlands). Aquat Ecol 37(1):13–22. doi:10.1023/A:1022120729031
Fahnenstiel GL, Lang GA, Nalepa TF, Johengen TH (1995) Effects of zebra mussel (Dreissena polymorpha) colonization on water quality parameters in Saginaw Bay, Lake Huron. J Gt Lakes Res 21(4):435–448
Friedrich G, Herbst V (2004) Eine erneute Revision des Saprobiensystems—weshalb und wozu? [Another revision of the saprobic index—why and what for?]. Acta Hydrochim Hydrobiol 32(1):61–74. doi:10.1002/aheh.200300518
Frischer ME, McGrath BR, Hansen AS, Vescio PA, Wyllie JA, Wimbush J, Nierzwicki-Bauer SA (2005) Introduction pathways, differential survival of adult and larval zebra mussels (Dreissena polymorpha), and possible management strategies, in an adirondack lake, Lake George, NY. Lake Reservior Manag 21(4):391–402
Gabriels W, Goethals PLM, De Pauw N (2005) Implications of taxonomic modifications and alien species on biological water quality assessment as exemplified by the Belgian Biotic Index method. Hydrobiologia 542:137–150. doi:10.1007/s10750-004-1452-7
Gayraud S, Statzner B, Bady P, Haybachp A, Scholl F, Usseglio-Polatera P, Bacchi M (2003) Invertebrate traits for the biomonitoring of large European rivers: an initial assessment of alternative metrics. Freshw Biol 48(11):2045–2064. doi:10.1046/j.1365-2427.2003.01139.x
Geiger W, Alcorlo P, Baltanas A, Montes C (2005) Impact of an introduced Crustacean on the trophic webs of Mediterranean wetlands. Biol Invasions 7(1):49–73. doi:10.1007/s10530-004-9635-8
Gérard C, Le Lannic J (2003) Establishment of a new host-parasite association between the introduced invasive species Potamopyrgus antipodarum (Smith) (Gastropoda) and Sanguinicola sp. Plehn (Trematoda) in Europe. J Zool (Lond) 261:213–216. doi:10.1017/S0952836903004084
Gergs R, Rothhaupt KO (2008) Effects of zebra mussels on a native amphipod and the invasive Dikerogammarus villosus: the influence of biodeposition and structural complexity. J N Am Benthol Soc 27(3):541–548. doi:10.1899/07-151.1
Gollasch S, Leppäkoski E (2007) Risk assessment and management scenarios for ballast water mediated species introductions into the Baltic Sea. Aquat Invasions 2(4):313–340. doi:10.3391/ai.2007.2.4.3
Gollasch S, Nehring S (2006) National checklist for aquatic alien species in Germany. Aquat Invasions 1(4):245–269. doi:10.1007/3-540-30023-6
Goncalves F, Ribeiro R, Soares A (1995) Rhithropanopeus harrisii (Gould), an American crab in the estuary of the Mondego-River, Portugal. J Crustac Biol 15(4):756–762. doi:10.2307/1548824
Gumuliauskaite S, Arbaciauskas K (2008) The impact of the invasive Ponto-Caspian amphipod Pontogammarus robustoides on littoral communities in Lithuanian lakes. Hydrobiologia 599:127–134. doi:10.1007/s10750-007-9209-8
Hakenkamp CC, Ribblett SG, Palmer MA, Swan CM, Reid JW, Goodison MR (2001) The impact of an introduced bivalve (Corbicula fluminea) on the benthos of a sandy stream. Freshw Biol 46(4):491–501. doi:10.1046/j.1365-2427.2001.00700.x
Hall RO, Dybdahl MF, Van der Loop MC (2006) Extremely high secondary production of introduced snails in rivers. Ecol Appl 16(3):1121–1131. doi:10.1890/1051-0761(2006)016[1121:EHSPOI]2.0.CO;2
Haynes JM, Stewart TW, Cook GE (1999) Benthic macroinvertebrate communities in southwestern Lake Ontario following invasion of Dreissena: continuing change. J Gt Lakes Res 25(4):828–838
Haynes JM, Tisch NA, Mayer CM, Rhyne RS (2005) Benthic macroinvertebrate communities in southwestern Lake Ontario following invasion of Dreissena and Echinogammarus: 1983 to 2000. J N Am Benthol Soc 24(1):148–167. doi:10.1899/0887-3593(2005)024<0148:BMCISL>2.0.CO;2
Horvath TG, Martin KM, Lamberti GA (1999) Effect of zebra mussels, Dreissena polymorpha, on macroinvertebrates in a lake-outlet stream. Am Midl Nat 142(2):340–347. doi:10.1674/0003-0031(1999)142[0340:EOZMDP]2.0.CO;2
James WF, Barko JW, Eakin HL (1997) Nutrient regeneration by the zebra mussel (Dreissena polymorpha). J Freshwat Ecol 12(2):209–216
Johannsson OE, Dermott R, Graham DM, Dahl JA, Millard ES, Myles DD, LeBlanc J (2000) Benthic and pelagic secondary production in Lake Erie after the invasion of Dreissena spp. with implications for fish production. J Gt Lakes Res 26(1):31–54
Karatayev AY, Burlakova LE (1995) The role of Dreissena in lake ecosystems. Russ J Ecol 26(3):207–211. doi:10.1596/0-8213-3187-6
Karatayev AY, Burlakova LE, Padilla DK (1997) The effects of Dreissena polymorpha (Pallas) invasion on aquatic communities in eastern Europe. J Shellfish Res 16(1):187–203
Karatayev AY, Burlakova LE, Kesterson T, Padilla DK (2003) Dominance of the Asiatic clam, Corbicula fluminea (Muller), in the benthic community of a reservoir. J Shellfish Res 22(2):487–493
Kas’yanov AN, Izyumov YG (1995) Growth and morphology of roach, Rutilus rutilus, from Lake Pleshcheyevo, after introduction of Dreissena polymorpha. J Ichthyol 35(8):253–256
Kelleher B, Bergers PJM, van den Brink FWB, Giller PS, van der Velde G, de Vaate AB (1998) Effects of exotic amphipod invasions on fish diet in the Lower Rhine. Arch Hydrobiol 143(3):363–382
Kelleher B, van der Velde G, Giller PS, Bij de Vaate A (2000) Dominant role of exotic invertebrates, mainly Crustacea, in diets of fish in the lower Rhine River. Crustac Issues 12:35–46
Kelly DW, Dick JTA (2005) Effects of environment and an introduced invertebrate species on the structure of benthic macroinvertebrate species at the catchment level. Arch Hydrobiol 164(1):69–88. doi:10.1127/0003-9136/2005/0164-0069
Kelly DW, Dick JTA, Montgomery WI, MacNeil C (2003) Differences in composition of macroinvertebrate communities with invasive and native Gammarus spp. (Crustacea: Amphipoda). Freshw Biol 48(2):306–315. doi:10.1046/j.1365-2427.2003.00977.x
Kerans BL, Dybdahl MF, Gangloff MM, Jannot JE (2005) Potamopyrgus antipodarum: distribution, density, and effects on native macroinvertebrate assemblages in the greater Yellowstone ecosystem. J N Am Benthol Soc 24(1):123–138. doi:10.1899/0887-3593(2005)024<0123:PADDAE>2.0.CO;2
Kerckhof F, Haelters J, Gollasch S (2007) Alien species in the marine and brackish ecosystem: the situation in Belgian waters. Aquat Invasions 2(3):243–257. doi:10.3391/ai.2007.2.3.9
Kinzler W, Maier G (2003) Asymmetry in mutual predation: possible reason for the replacement of native gammarids by invasives. Arch Hydrobiol 157(4):473–481. doi:10.1127/0003-9136/2003/0157-0473
Kley A, Maier G (2003) Life history characteristics of the invasive freshwater gammarids Dikerogammarus villosus and Echinogammarus ischnus in the river Main and the Main-Donau canal. Arch Hydrobiol 156(4):457–469. doi:10.1127/0003-9136/2003/0156-0457
Kovalak WP, Longton GT, Smithee RD (1992) Infestation of power plant water systems by the Zebra mussel (Dreissena polymorpha). In: Nalepa FT, Schloesser D (eds) Zebra mussels biology, impacts, and control. CRC, Boca Raton, p 832
Kube S, Postel L, Honnef C, Augustin CB (2007) Mnemiopsis leidyi in the Baltic Sea—distribution and overwintering between autumn 2006 and spring 2007. Aquat Invasions 2(2):137–145. doi:10.1007/978-3-540-36920-2
Kuhns LA, Berg MB (1999) Benthic invertebrate community responses to round goby (Neogobius melanostomus) and zebra mussel (Dreissena polymorpha) invasion in southern Lake Michigan. J Gt Lakes Res 25(4):910–917
Kureck A (1992) New fauna in the Rhine River—Resettlement of the current and propagation of neozoa. Naturwissenschaften 79(12):533–540. doi:10.1007/BF01131409
Laine AO, Mattila JAL (2006) First record of the brackish water dreissenid bivalve Mytilopsis leucophaeata in the northern Baltic Sea. Aquat Invasions 1(1):38–41. doi:10.1007/3-540-30023-6
Lalaguna CD, Marco AA (2008) The zebra mussel invasion in Spain and navigation rules. Aquat Invasions 3(3):315–324. doi:10.3391/ai.2008.3.3.6
Lawson J, Davenport J, Whitaker A (2004) Barnacle distribution in Lough Hyne Marine Nature Reserve: a new baseline and an account of invasion by the introduced Australasian species Elminius modestus Darwin. Estuar Coast Shelf Sci 60(4):729–735. doi:10.1016/j.ecss.2004.03.011
Le Page WL (1992) The impact of Dreissena polymorpha on waterworks operations at Monroe, Michigan: a case history. In: Nalepa FT, Schloesser D (eds) Zebra mussels biology, impacts, and control. CRC, Boca Raton 832 pp
Le Pape O, Guerault D, Desaunay Y (2004) Effect of an invasive mollusc, American slipper limpet Crepidula fornicata, on habitat suitability for juvenile common sole Solea solea in the Bay of Biscay. Mar Ecol Prog Ser 277:107–115. doi:10.3354/meps277107
Leung B, Finnoff D, Shogren JF, Lodge D (2005) Managing invasive species: rules of thumb for rapid assessment. Ecol Econ 55(1):24–36. doi:10.1016/j.ecolecon.2005.04.017
MacIsaac HJ, Johannsson OE, Ye J, Sprules WG, Leach JH, McCorquodale JA, Grigorovich IA (1999) Filtering impacts of an introduced bivalve (Dreissena polymorpha) in a shallow lake: application of a hydrodynamic model. Ecosystems (NY, Print) 2(4):338–350. doi:10.1007/s100219900084
MacNeil C, Dick JTA, Elwood RW, Montgomery WI (2001a) Coexistence among native and introduced freshwater amphipods (Crustacea); habitat utilization patterns in littoral habitats. Arch Hydrobiol 151(4):591–607
MacNeil C, Montgomery WI, Dick JTA, Elwood RW (2001b) Factors influencing the distribution of native and introduced Gammarus spp. in Irish river systems. Arch Hydrobiol 151(3):353–368
MacNeil C, Bigsby E, Dick JTA, Hynes HBN, Hatcher MJ, Dunn AM (2003) Temporal changes in the distribution of native and introduced freshwater amphipods in Lough Neagh, Northern Ireland. Arch Hydrobiol 157(3):379–395. doi:10.1127/0003-9136/2003/0157-0379
Makarewicz JC, Bertram P, Lewis TW (2000) Chemistry of the offshore surface waters of Lake Erie: pre- and post- Dreissena introduction (1983–1993). J Gt Lakes Res 26(1):82–93
Minchin D, David M, Olenin S, Gollasch S (2006) In: ‘Bridging divides’—man-made shipping canals as migration pathways of non-indigenous species. Kluwer Academic Press, New York
Molloy DP, Roitman VA, Shields JD (1996) Survey of the parasites of zebra mussels (Bivalvia: Dreissenidae) in northwestern Russia, with comments on records of parasitism in Europe and North America. J Helm Soc Wash 63(2):251–256
Montalto L, de Drago IE (2003) Tolerance to desiccation of an invasive mussel, Limnoperna fortunei (Dunker, 1857) (Bivalvia, Mytilidae), under experimental conditions. Hydrobiologia 498(1–3):161–167. doi:10.1023/A:1026222414881
Mörtl M, Rothhaupt KO (2003) Effects of adult Dreissena polymorpha on settling juveniles and associated macroinvertebrates. Int Rev Hydrobiol 88(6):561–569. doi:10.1002/iroh.200310640
Mouthon J (2001a) Life cycle and population dynamics of the Asian clam Corbicula fluminea (Bivalvia: Corbiculidae) in the Saone river at Lyon (France). Hydrobiologia 452(1–3):109–119. doi:10.1023/A:1011980011889
Mouthon J (2001b) Life cycle and population dynamics of the Asian clam Corbicula fluminea (Bivalvia: Corbiculidae) in the Rhone River at Creys-Malville (France). Arch Hydrobiol 151(4):571–589
Mouthon J (2003) Longitudinal and temporal variations of density and size structure of Corbicula fluminea (Bivalvia) populations in the Saone and Rhone rivers (France). Ann Limnol 39(1):15–25
Mueller J (2001) Invasion history and genetic population structure of riverine macro invertebrates. Zool-Anal Complex Sy 104(3–4):346–355
Mueller JC, Hidde D, Seitz A (2002) Canal construction destroys the barrier between major European invasion lineages of the zebra mussel. Proc R Soc Lond B Biol Sci 269(1496):1139–1142. doi:10.1098/rspb.2002.1994
Nalepa TF (1994) Decline of native unionid bivalves in lake st clair after infestation by the zebra mussel, Dreissena polymorpha. Can J Fish Aquat Sci 51(10):2227–2233. doi:10.1139/f94-225
Nehring S (2006) Four arguments why so many alien species settle into estuaries, with special reference to the German river Elbe. Helgol Mar Res 60(2):127–134. doi:10.1007/s10152-006-0031-x
Nel R, Coetzee PS, Van Niekerk G (1996) The evaluation of two treatments to reduce mud worm (Polydora hoplura Claparede) infestation in commercially reared oysters (Crassostrea gigas Thunberg). Aquaculture 141(1–2):31–39. doi:10.1016/0044-8486(95)01212-5
NOBANIS (2009) North European and Baltic network on invasive alien species. www.nobanis.org. Cited 01 May 2009
Nonindigenous Aquatic Species (2009) http://nas.er.usgs.gov/. Cited 31 Jan 2009
Occhipinti-Ambrogi A, Savini D (2003) Biological invasions as a component of global change in stressed marine ecosystems. Mar Pollut Bull 46(5):542–551. doi:10.1016/S0025-326X(02)00363-6
Olenin S, Minchin D, Daunys D (2007) Assessment of biopollution in aquatic ecosystems. Mar Pollut Bull 55(7–9):379–394. doi:10.1016/j.marpolbul.2007.01.010
ÖNORM (1997) ÖNORM M 6232:1997 05 01. Guidelines for the ecological study and assessment of rivers (bilingual edition)
Packalén A, Korpinen S, Lehtonen KK (2008) The invasive amphipod species Gammarus tigrinus (Sexton 1939) can rapidly change littoral communities in the Gulf of Finland (Baltic Sea). Aquat Invasions 3(4):405–412. doi:10.3391/ai.2008.3.4.5
Padilla DK (2005) The potential of zebra mussels as a model for invasion ecology. Am Malacol Bull 20(1–2):123–131
Paffen BGP, Van den Brink FWB, Van der Velde G, De Vaate AB (1994) The population explosion of the amphipod Corophium curvispinum in the Dutch Lower Rhine. Water Sci Technol 29(3):53–55
Palacios R, Armstrong DA, Orensanz JL (2000) Fate and legacy of an invasion: extinct and extant populations of the soft-shell clam (Mya arenaria) in Grays Harbor (Washington). Aquat Conserv 10(4):279–303. doi:10.1002/9780470999356
Palmer ME, Ricciardi A (2005) Community interactions affecting the relative abundance of native and invasive amphipods in the St. Lawrence River. Can J Fish Aquat Sci 62:1111–1118
Phelps HL (1994) The Asiatic clam (Corbicula fluminea) Invasion and system-level ecological change in the Potomac River estuary near Washington, Dc. Estuaries 17(3):614–621. doi:10.2307/1352409
Pillsbury RW, Lowe RL, Pan YD, Greenwood JL (2002) Changes in the benthic algal community and nutrient limitation in Saginaw Bay, Lake Huron, during the invasion of the zebra mussel (Dreissena polymorpha). J N Am Benthol Soc 21(2):238–252. doi:10.2307/1468412
Pilollo F, Free G, Crosa G, Sena F, Ghiani M, Cardoso AC (2008) The invasive crayfish Orconectes limosus in Lake Varese: estimating abundance and population size structure in the context of habitat and methodological constraints. J Crustac Biol 28(4):633–640. doi:10.1651/07-2967.1
Piscart C, Devin S, Beisel JN, Moreteau JC (2003) Growth-related life-history traits of an invasive gammarid species: evaluation with a Laird-Gompertz model. Can J Zool/Rev Can Zool 81(12):2006–2014
Piscart C, Usseglio-Polatera P, Moreteau JC, Beisel JN (2006) The role of salinity in the selection of biological traits of freshwater invertebrates. Arch Hydrobiol 166(2):185–198. doi:10.1127/0003-9136/2006/0166-0185
Rajagopal S, van der Velde G, Paffen BGP, van den Brink FWB, de Vaate AB (1999) Life history and reproductive biology of the invasive amphipod Corophium curvispinum (Crustacea: Amphipoda) in the Lower Rhine. Arch Hydrobiol 144(3):305–325
Rajagopal S, van der Velde G, de Vaate AB (2000) Reproductive biology of the Asiatic clams Corbicula fluminalis and Corbicula fluminea in the River Rhine. Arch Hydrobiol 149(3):403–420
Reeze B, Greijdanus M, Bij de Vaate A (2005) Invasive macroinvertebrates and the ecological quality of large rivers. University of Florence, Italy. Workshop “Biological Invasions of Inland Waters” http://www.dbag.unifi.it/inwat/presentations/reeze.pdf. Cited 08 Feb 2008
Ricciardi A (2003) Predicting the impacts of an introduced species from its invasion history: an empirical approach applied to zebra mussel invasions. Freshw Biol 48(6):972–981. doi:10.1046/j.1365-2427.2003.01071.x
Ricciardi A, Cohen J (2007) The invasiveness of an introduced species does not predict its impact. Biol Invasions 9(3):309–315. doi:10.1007/s10530-006-9034-4
Ricciardi A, Kipp R (2008) Predicting the number of ecologically harmful exotic species in an aquatic system. Divers Distrib 14(2):374–380. doi:10.1111/j.1472-4642.2007.00451.x
Ricciardi A, Maclsaac HJ (2000) Recent mass invasion of the North American Great Lakes by Ponto-Caspian species. Trends Ecol Evol 15(2):62–65. doi:10.1016/S0169-5347(99)01745-0
Ricciardi A, Whoriskey FG (2004) Exotic species replacement: shifting dominance of dreissenid mussels in the Soulanges Canal, upper St. Lawrence River, Canada. J N Am Benthol Soc 23(3):507–514. doi:10.1899/0887-3593(2004)023<0507:ESRSDO>2.0.CO;2
Ricciardi A, Whoriskey FG, Rasmussen JB (1996) Impact of the Dreissena invasion on native unionid bivalves in the upper St Lawrence River. Can J Fish Aquat Sci 53(6):1434–1444. doi:10.1139/cjfas-53-6-1434
Ricciardi A, Whoriskey FG, Rasmussen JB (1997) The role of the zebra mussel (Dreissena polymorpha) in structuring macroinvertebrate communities on hard substrata. Can J Fish Aquat Sci 54(11):2596–2608. doi:10.1139/cjfas-54-11-2596
Schloesser DW, Kovalak WP, Longton GD, Ohnesorg KL, Smithee RD (1998) Impact of zebra and quagga mussels (Dreissena spp.) on freshwater unionids (Bivalvia: Unionidae) in the Detroit River of the Great Lakes. Am Midl Nat 140(2):299–313. doi:10.1674/0003-0031(1998)140[0299:IOZAQM]2.0.CO;2
Schreiber ESG, Lake PS, Quinn GP (2002) Facilitation of native stream fauna by an invading species? Experimental investigations of the interaction of the snail, Potamopyrgus antipodarum (Hydrobiidae) with native benthic fauna. Biol Invasions 4(3):317–325. doi:10.1023/A:1020925022843
Semenchenko V, Laenko T (2008) First record of the invasive North American gastropod Ferrissia fragilis (Tryon, 1863) from the Pripyat River basin, Belarus. Aquat Invasions 3(1):80–82. doi:10.3391/ai.2008.3.1.12
Semenchenko V, Vezhnovetz V (2008) Two new invasive Ponto-Caspian amphipods reached the Pripyat River, Belarus. Aquat Invasions 3(4):445–447. doi:10.3391/ai.2008.3.4.14
Simberloff D (2005) The politics of assessing risk for biological invasions: the USA as a case study. Trends Ecol Evol 20(5):216–222. doi:10.1016/j.tree.2005.02.008
Simberloff D, Gibbons L (2004) Now you see them, now you don’t—population crashes of established introduced species. Biol Invasions 6(2):161–172. doi:10.1023/B:BINV.0000022133.49752.46
Smith DG, Werle SF, Klekowski E (2002) The rapid colonization and emerging biology of Cordylophora caspia (Pallas, 1771) (Cnidaria: Clavidae) in the Connecticut River. J Freshwat Ecol 17(3):423–430
Statzner B, Bonada N, Doledec S (2008) Biological attributes discriminating invasive from native European stream macroinvertebrates. Biol Invasions 10(4):517–530. doi:10.1007/s10530-007-9148-3
Stewart TW, Miner JG, Lowe RL (1998) Macroinvertebrate communities on hard substrates in western Lake Erie: structuring effects of Dreissena. J Gt Lakes Res 24(4):868–879
Strayer DL, Smith LC (2001) The zoobenthos of the freshwater tidal Hudson River and its response to the zebra mussel (Dreissena polymorpha) invasion. Arch Hydrobiol 139:1–52
Strayer DL, Hattala KA, Kahnle AW (2004) Effects of an invasive bivalve (Dreissena polymorpha) on fish in the Hudson River estuary. Can J Fish Aquat Sci 61(6):924–941. doi:10.1139/f04-043
Stucki TP, Romer J (2001) Will Astacus leptodactylus displace Astacus astacus and Austropotamobius torrentium in Lake Ageri, Switzerland? Aquat Sci 63(4):477–489. doi:10.1007/s00027-001-8046-5
Therriault TW, Orlova MI, Docker MF, MacIsaac HJ, Heath DD (2005) Invasion genetics of a freshwater mussel (Dreissena rostriformis bugensis) in eastern Europe: high gene flow and multiple introductions. Heredity 95(1):16–23. doi:10.1038/sj.hdy.6800691
Thieltges DW (2005) Impact of an invader: epizootic American slipper limpet Crepidula fornicata reduces survival and growth in European mussels. Mar Ecol Prog Ser 286:13–19. doi:10.3354/meps286013
Thieltges DW, Strasser M, Reise K (2006) How bad are invaders in coastal waters? The case of the American slipper limpet Crepidula fornicata in western Europe. Biol Invasions 8(8):1673–1680. doi:10.1007/s10530-005-5279-6
Trometer ES, Busch WDN (1999) Changes in age-0 fish growth and abundance following the introduction of zebra mussels Dreissena polymorpha in the western basin of Lake Erie. N Am J Fish Manag 19(2):604–609. doi:10.1577/1548-8675(1999)019<0604:CIAFGA>2.0.CO;2
van den Brink FWB, van der Velde G, Devaate AB (1993) Ecological Aspects, Explosive Range Extension and Impact of a Mass Invader, Corophium curvispinum Sars, 1895 (Crustacea, Amphipoda), in the Lower Rhine (the Netherlands). Oecologia 93(2):224–232. doi:10.1007/BF00317675
van der Velde G, Platvoet D (2007) Quagga mussels Dreissena rostriformis bugensis (Andrusov, 1897) in the Main River (Germany). Aquat Invasions 2(3):261–264. doi:10.3391/ai.2007.2.3.11
van der Velde G, Paffen BGP, van den Brink FWB, Bij de Vaate A, Jenner HA (1994) Decline of Zebra mussel populations in the Rhine. Competition between two mass invaders (Dreissena polymorpha and Corophium curvispinum). Naturwissenschaften 81:32–34. doi:10.1007/BF01138559
van Riel MC, van der Velde G, De Vaate AB (2003) Pomphorhynchus spec. (Acanthocephala) uses the invasive amphipod Chelicorophium curvispinum (G. O. SARS, 1895) as an intermediate host in the River Rhine. Crustaceana 76(2):241–246. doi:10.1163/156854003321824585
van Riel MC, van der Velde G, de Vaate AB (2006a) To conquer and persist: colonization and population development of the Ponto-Caspian amphipods Dikerogammarus villosus and Chelicorophium curvispinum on bare stone substrate in the main channel of the River Rhine. Arch Hydrobiol 166(1):23–39. doi:10.1127/0003-9136/2006/0166-0023
van Riel MC, van der Velde G, Rajagopal S, Marguillier S, Dehairs F, de Vaate AB (2006b) Trophic relationships in the Rhine food web during invasion and after establishment of the Ponto-Caspian invader Dikerogammarus villosus. Hydrobiologia 565:39–58. doi:10.1007/s10750-005-1904-8
van Riel MC, Healy EP, van der Velde G, de Vaate AB (2007) Interference competition among native and invader amphipods. Acta Oecologica-International. J Ecol 31(3):282–289
Wijnhoven S, van Riel MC, van der Velde G (2003) Exotic and indigenous freshwater gammarid species: physiological tolerance to water temperature in relation to ionic content of the water. Aquat Ecol 37(2):151–158. doi:10.1023/A:1023982200529
Wilson AB, Naish KA, Boulding EG (1999) Multiple dispersal strategies of the invasive quagga mussel (Dreissena bugensis) as revealed by microsatellite analysis. Can J Fish Aquat Sci 56(12):2248–2261. doi:10.1139/cjfas-56-12-2248
Wolff WJ (1999) Exotic invaders of the meso-oligohaline zone of estuaries in the Netherlands: why are there so many? Helgol Meersunters 52:393–400. doi:10.1007/BF02908913
Zanatta DT, Mackie GL, Metcalfe-Smith JL, Woolnough DA (2002) A refuge for native freshwater mussels (Bivalvia: Unionidae) from impacts of the exotic zebra mussel (Dreissena polymorpha) in Lake St. Clair. J Gt Lakes Res 28(3):479–489
Zaranko DT, Farara DG, Thompson FG (1997) Another exotic mollusc in the Laurentian Great Lakes: the New Zealand native Potamopyrgus antipodarum (Gray 1843) (Gastropoda, Hydrobiidae). Can J Fish Aquat Sci 54(4):809–814. doi:10.1139/cjfas-54-4-809
Zhang S, Shi Z, Zhang J, Bonami JR (2004) Purification and characterization of a new reovirus from the Chinese mitten crab, Eriocheir sinensis. J Fish Dis 27(12):687–692. doi:10.1111/j.1365-2761.2004.00587.x
Acknowledgments
The study was funded by the European Commission within the project MODELKEY (FP 6, 511237-GOCE). We would like to thank Emma Schymanski for reviewing the manuscript linguistically and two anonymous reviewers for useful comments.
Author information
Authors and Affiliations
Corresponding author
Rights and permissions
About this article
Cite this article
Orendt, C., Schmitt, C., van Liefferinge, C. et al. Include or exclude? A review on the role and suitability of aquatic invertebrate neozoa as indicators in biological assessment with special respect to fresh and brackish European waters. Biol Invasions 12, 265–283 (2010). https://doi.org/10.1007/s10530-009-9448-x
Received:
Accepted:
Published:
Issue Date:
DOI: https://doi.org/10.1007/s10530-009-9448-x