Abstract
Bone morphogenetic proteins (BMPs) are known to be important in osteoblasts’ response to mechanical stimuli. BMPs/Smad signaling pathway has been demonstrated to play a regulatory role in the mechanical signal transduction in osteoblasts. However, little is currently known about the Smad independent pathway in osteoblasts differentiation in mechanical loading. In this study, MC3T3-E1 cells were subjected to mechanical stretch of 2000 micro-stain (με) at 0.5 Hz, in order to investigate the involvement of p38MAPK and NF-κB signaling pathways in mechanical response in osteoblasts. We found BMP-2/BMP-4 were up-regulated by mechanical stretch via the earlier activation of p38MAPK and NF-κB signaling pathways, which enhanced osteogenic gene expressions including alkaline phosphatase (ALP), collagen type I (Col I) and osteocalcin (OCN), and the expressions of these osteogenic genes were remarkably decreased with Noggin (an inhibitor for BMPs signals) pretreatment. Furthermore, BMP-2/BMP-4 expressions were suppressed by PDTC, an inhibitor of NF-κB pathway and SB203580, an inhibitor of p38MAPK pathway, respectively, leading to the declined levels of ALP, Col I and OCN. Interestingly, blocking in p38MAPK pathway can also cause the inactivation of NF-κB pathway in mechanical stretch. Collectively, the results indicate during mechanical stretch p38MAPK and NF-κB signaling pathways are activated first, and then up-regulate BMP-2/BMP-4 to enhance osteogenic gene expressions. Moreover, p38MAPK and NF-κB signals have cross-talk in regulation of BMP-2/BMP-4 in mechanical response.
Similar content being viewed by others
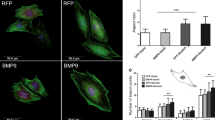
Avoid common mistakes on your manuscript.
Introduction
Bone tissue is a specialized form of connective tissue playing a critical load-bearing role in human body, and a dynamic organ with constant remodeling throughout life. Bone remodeling is a complex biological process depending on genetic, hormonal, metabolic, age-related factors and mechanical environment.24 Mechanical loading plays an essential role in bone remodeling, since suitable mechanical stimuli can maintain normal bone volume, while removal of mechanical stimuli can cause the loss of bone mass.31 Osteoblasts, originating from mesenchymal stem cells (MSCs), are well evidenced to be the crucial effector cells in the process of bone remodeling.16 Osteoblasts and their precursor cells are sensitive to mechanical stimuli, and have an ability to translate mechanical signals into biological responses to regulate the bone remodeling in intact bone and bone repair. The responses to mechanical force in osteoblasts have great influences on an interaction of various biological factors, such as prostaglandin e2 (PGE2), osteoprotegerin (OPG), receptor activator of nuclear factor ligand (RANKL) and bone morphogenetic proteins (BMPs) that are generally considered important in bone remodeling.
Bone morphogenetic proteins, identified as members of the transforming growth factor (TGF)-β super-families and a kind of secretary glycoprotein purified from bone matrix in human, are potent osteoblastic differentiation factors playing a pivotal regulatory role in remodeling and formation of bone.2 It has been reported that BMPs can induce the transformation of mesenchyme stem cells into osteoblasts and chondrocytes,20 and promote osteogenesis and new bone formation in vitro.1 Furthermore, the mechanical loading in osteoblasts has been demonstrated to increase the expressions of BMPs,15 suggesting BMPs and their related signaling pathways may be involved in the mechanical response in osteoblasts.
The intracellular signaling of BMPs is mediated primarily by Smad proteins. Smad proteins can be activated by BMPs through a complex serine-threonine receptor mechanism and then move into the nucleus to modulate the expression of osteoblasts related genes.21 Many reports have demonstrated that mechanical forces can influence the expressions of BMPs in osteoblasts,22,36 indicating the potent regulatory role of BMP/Smad pathway in mechanical signal transduction in osteoblast. Our previous study showed mechanical stretch can activate Smad1 and Smad5 signaling pathways by up-regulating BMP-2 expression.40 Although the involvement of Smad signaling pathway in osteoblasts differentiation has been well investigated, little is currently known about the possible involvement of Smad independent pathway in osteoblasts’ response to mechanical loading. As we know, p38 mitogen-activated protein kinase (p38MAPK) signaling pathway acts as the crucial signal transduction cascades contributing to neuronal differentiation, adipogenesis and chondrogenesis.8 Some reports indicate nuclear factor kappa-B (NF-κB) signaling is associated with bone metabolic diseases and inhibition of this pathway can suppress inflammatory bone loss by inhibiting osteoclastogenesis.11,34 It has been reported that p38MAPK and NF-κB signaling pathways are involved in the transmission of BMPs signals and the regulation of BMPs expressions.9,32 However, the interrelation between p38MAPK and NF-κB signaling pathways in mechanical responses and the detailed mechanism in modulating BMPs-induced osteoblasts differentiation have not been fully elucidated. Therefore, the purpose of this study was to examine the hypothesis that activation of p38MAPK and NF-κB pathways are required for regulating BMPs signals to enhance osteogenic gene expressions during mechanical stretch by investigating: (1) the effects of mechanical stretch on p38MAPK and NF-κB signaling pathways; (2) the mechanism of p38MAPK and NF-κB pathways to regulate the activation of BMP-2/BMP-4 in mechanical responses.
Materials and Methods
Reagents
The Superscript III Reverse Transcriptase Kit was purchased from Invitrogen (Carlsbad, CA). ALP, Col I, OCN, BMP-2, BMP-4, p38, p-p38, p65, p-p65, IκBα and p-IκBα antibodies were obtained from Santa Cruz Biotechnology (Delaware Ave, CA). The proteases inhibitors, PDTC and SB203580 were purchased from Sigma (St. Louis, MO).
Cell Culture
MC3T3-E1 cells, a mouse monoclonal pre-osteoblastic cell line that has been demonstrated to differentiate into osteoblasts and osteocytes,33 were maintained in α-Minimum Essential Medium (α-MEM; Gibco-BRL, GrandIsland, NY) supplemented with 10% fetal bovine serum (FBS; Gibco-BRL, GrandIsland, NY) and 1% penicillin–streptomycin (Invitrogen, Carlsbad, CA) in 5% CO2 at 37 °C. The following pharmacological agents were used to evaluate the potential role of various signaling pathways in mechanical stretch: the proteases inhibitors Noggin (50 ng/mL), PDTC (4 μM) and SB203580 (10 μM). They were added to cell culture 1 h prior to mechanical stretch application and remained in the culture media throughout the experiment.
Application of Mechanical Stretch to Cultured Cells
Uniaxial and homogeneous mechanical tension was generated by a specially designed four-point bending device described previously.35 In the present study, cells were seeded at the density of 104/cm2 in culture dishes until they reached 80% confluence, and then were subjected to mechanical stretch of 2000 με at 0.5 Hz for the indicated time. Control cells were incubated under the same conditions except for application of mechanical stimuli.
Alkaline Phosphates (ALP) Activity Analysis
After mechanical stretch, MC3T3-E1 cells were harvested and lysed in the lysis buffer (10 mmol/L HEPES, 250 mmol/L sucrose, 5 mmol/L Tris–HCl, 0.1% Triton X-100, pH 7.5). ALP activities in each sample were examined by a fluorometric detection kit (Nanjing Jiancheng Biotechnology Co. Ltd, Nanjing, China). Values of ALP activity were normalized by protein concentration.
RT-PCR Analysis
For investigating the influence of mechanical stretch on mRNA expressions of the related genes, reverse transcription (RT)-polymerase chain reaction (PCR) was performed as previously described.12 Briefly, total RNA was extracted from MC3T3-E1 cells with Trizol reagent (Invitrogen, Carlsbad, CA) and the concentration was determined by the absorbance at 260 nm. A total of 2 μg RNA was reversely transcribed using the Superscript III Reverse Transcriptase Kit (Invitrogen, Carlsbad, CA). cDNA mixtures were subjected to PCR amplification and the primers used in this study were shown in Table 1. The PCR products were determined by 1.5% agarose gel and the relative intensities of gel bands were measured.
Western Blot Analysis
Cells were lysed in the lysis buffer containing inhibitors for proteases and phosphatases. The lysate was centrifuged at 14,000 rpm for 10 min (4 °C) and the supernatant was collected. The protein concentration was measured by Lowry’s method. Equal amounts of proteins were then separated by SDS-PAGE and electrotransferred onto PVDF membranes (Millipore, Bedford, MA). After transference, the membranes blocked with 5% skim milk were incubated with the desired primary antibody at 4 °C overnight. After washing, the membranes were then incubated with secondary antibody conjugated with horseradish peroxidase. The immunoreactive bands were visualized by enhanced chemiluminescence detection kit (Santa Cruz, Delaware Ave., CA). The densities of bands were quantified by image analysis system Gel Doc 2000 (Bio-Rad, Hercules, CA) and determined with a ratio to Tubulin.
Statistical Analysis
All data were presented as mean ± SD from at least three independent experiments. Statistical differences were evaluated by one-way analysis of variance (ANOVA) followed by the least significant difference (LSD) t test with p < 0.05 considered significant.
Results
Expressions of Osteogenic Genes in Mechanical Response of Osteoblasts
ALP, Col I and OCN are believed to be the major characteristics of osteoblast activity. To see if mechanical stretch enhances osteogenic gene expression, we determined the expressions of these genes. The mRNA levels of these genes (Fig. 1a) were significantly increased after stretch for 4 h, and ALP started to decrease after 8 h, while Col I and OCN started to decrease after 12 h. The changes in protein levels of them were further confirmed by western blot (Fig. 1b) and ALP activity analysis (Fig. 1c). The results indicate that mechanical stretch can improve osteoblast differentiation.
Effect of mechanical stretch on osteoblast differentiation. MC3T3-E1 cells were subjected to mechanical stretch for 2–24 h. Both mRNA levels (a) and protein levels (b, c) of ALP, Col I and OCN were remarkably increased in the loaded cells after 4 h. Results are shown as mean ± SE (n = 3) from three independent experiments. *p < 0.05 vs. Control (0 h)
Regulation of BMP-2 and BMP-4 by Mechanical Stretch
In this study, we found mechanical loading up-regulated the expressions of BMP-2 and BMP-4 in the time dependence. Both mRNA (Fig. 2a) and protein levels (Fig. 2b) of BMP-2 and BMP-4 started to increase at 4 h and the maximum expression levels were observed at 8 h after initiating mechanical stimuli, and subsequently they returned to the basal level at 24 h. Thus, we found the activities of osteogenic related genes were enhanced following the same time dependent changes of BMP-2 and BMP-4. To confirm further the involvement of BMP-2/BMP-4 regulations in mechanical response, MC3T3-E1 cells were pretreated with 50 ng/mL Noggin (an inhibitor for BMPs signals) before application of stretch, and then subjected to stretch for 8 h. After loading, mRNA (Fig. 2c) and protein levels (Figs. 2d, 2e) of ALP, Col I and OCN expressions remarkably decreased with Noggin pretreatment in osteoblasts. The findings indicate that mechanical stretch can promote osteoblasts differentiation via up-regulation of BMPs.
Regulations of BMP-2 and BMP-4 in mechanical stretch. MC3T3-E1 cells were subjected to mechanical stretch for 2–24 h. Expressions of BMP-2/BMP-4 (a, b) were remarkably increased after 4 h, and the levels of ALP, Col I and OCN (c–e) were suppressed with Noggin pretreatment in the loaded cells. Results are shown as mean ± SE (n = 3) from three independent experiments. *p < 0.05 vs. Control (0 h)
Involvement of NF-κB Signaling Pathway in Mechanical Response
To determine whether osteoblasts response to mechanical stretch was mediated by NF-κB signaling pathway, we first investigated the influence of mechanical stretch on the expressions of IκBα, p-IκBα (phosphorylation of IκBα) and p-p65 (phosphorylation of p-p65) which were the signals in activation of NF-κB pathway. We found that the expressions of p-IκBα and p-p65 started to increase at 30 min and the maximum expression levels were observed at 60 min after initiating mechanical stimuli (Fig. 3). Meanwhile the expression of IκBα significantly decreased due to its degradation (Fig. 3). The data suggest NF-κB signals are activated earlier than BMP-2/BMP-4 in the time course of mechanical response. Next, the MC3T3-E1 cells were pretreated with 4 μM PDTC (an inhibitor for NF-κB pathway) for 1 h before application of stretch, and then subjected to mechanical stretch for 8 h. After mechanical loading, mRNA (Fig. 4a) and protein levels (Fig. 4b) of BMP-2 and BMP-4 expressions remarkably decreased with PDTC pretreatment in osteoblasts, finally resulting in the down-regulation of ALP, Col I and OCN expressions in mRNA (Fig. 4c) and protein levels (Figs. 4d, 4e). These results show that activation of NF-κB signaling pathway is required for modulating BMPs signals to enhance osteogenic gene expressions during mechanical stretch.
Effect of mechanical stretch on NF-κB signaling pathway. MC3T3-E1 cells were subjected to mechanical stretch for 15–120 min. Expressions of p-IκBα and p-p65 significantly increased, while IκBα expression decreased in the loaded cells. Results are shown as mean ± SE (n = 3) from three independent experiments. *p < 0.05 vs. Control (0 min)
Pretreatment with PDTC attenuated the effect of mechanical stretch. MC3T3-E1 cells were pretreated with PDTC (4 μM) for 1 h and then subjected to the strain for 8 h. Pretreatment with PDTC decreased the expressions of BMP-2 and BMP-4 in mRNA levels (a) and protein levels (b), leading to the declined expressions of ALP, Col I and OCN in mRNA levels (c) and protein levels (d, e). *p < 0.05 vs. Control or between the indicated groups (n = 3). Results represent three independent experiments
Involvement of P38MAPK Signaling Pathway in Mechanical Response
To confirm whether p38MAPK signaling pathway was involved in mechanical signal transduction in osteoblasts, we first determined the phosphorylation level of p38 (p-p38), the indicator of activation in p38MAPK pathway, in osteoblasts subjected to mechanical stretch for 12 h. We found that the expression of p-p38 started to increase at 15 min and the maximum expression level was observed at 90 min after initiating mechanical stimuli (Fig. 5). The data suggest p38MAPK signals are activated earlier than NF-κB and BMP-2/BMP-4 in the time course of mechanical response. To confirm further the relationship between p38MAPK and BMPs signals, levels of p38 phosphorylation (p-p38) were determined in osteoblasts pretreated with Noggin. After loading for 1 h, we found p38MAPK signals activated by stretch were not influenced by block of BMPs (Fig. 6), which indicate p38MAPK pathway is not the downstream signal of BMPs in mechanical response. Next, the MC3T3-E1 cells were pretreated with 10 μM SB203580 (an inhibitor for p38MAPK pathway) for 1 h before application of stretch, and then subjected to mechanical stretch for 8 h. After mechanical loading, mRNA (Fig. 7a) and protein levels (Fig. 7b) of BMP-2 and BMP-4 expressions were suppressed with SB203580 pretreatment in osteoblasts, which subsequently caused the down-regulation of ALP, Col I and OCN expressions in mRNA (Fig. 7c) and protein levels (Figs. 7d, 7e). The above findings reveal that activation of p38MAPK signaling pathway is required for modulating BMPs signals to enhance osteogenic gene expressions during mechanical stretch. In addition, to investigate the correlation between p38MAPK and NF-κB signaling pathways, we determined the effect of p38MAPK signaling inhibitor on NF-κB pathway. Interestingly, we found the expression of activated p65 (p-p65) was decreased with SB203580 pretreatment (Fig. 8), suggesting the block in p38MAPK pathway can intercept the activation of NF-κB pathway and they have cross-talk in regulation of BMPs to enhance osteogenic gene expression in mechanical response.
p38MAPK signals activated by stretch were not influenced with Noggin pretreatment in the loaded cells. MC3T3-E1 cells were pretreated with Noggin (50 ng/mL) for 1 h and then subjected to the strain for 1 h. Pretreatment with Noggin did not decrease the expression of p-p38. Results are shown as mean ± SE (n = 3) from three independent experiments. *p < 0.05 vs. Control
Pretreatment with SB203580 reduced the effect of mechanical stretch. MC3T3-E1 cells were pretreated with SB203580 (10 μM) for 1 h and then subjected to the stretch for 8 h. Pretreatment with SB203580 decreased the expressions of BMP-2 and BMP-4 in mRNA levels (a) and protein levels (b), inducing the declined expressions of ALP, Col I and OCN in mRNA levels (c) and protein levels (d, e). *p < 0.05 vs. Control or between the indicated groups (n = 3). Results represent three independent experiments
Pretreatment with SB203580 inhibited the activation of NF-κB signaling pathway. MC3T3-E1 cells were pretreated with SB203580 (10 μM) for 1 h and then subjected to the stretch for 8 h. Pretreatment with SB203580 intercepted the phosphorylation of p65 protein. *p < 0.05 vs. Control or between the indicated groups (n = 3). Results represent three independent experiments
Discussion
It is generally considered that mechanical loading is a fundamental physiological factor regulating the structure and function of bones. Absence of mechanical loading can lead to the loss of bone mass, while suitable mechanical force can stimulate bone formation.29 It has been confirmed that the dynamic load is associated with the increased bone mass, whereas the similar static load has no effect on bone remodeling.17 Moreover, a suitable dynamic load not only induces the periosteal surface to transform directly from quiescence to active bone formation,27 but also modulates the bone loss caused by calcium insufficiency.18 During evolution, bone tissue acts as the load-bearing role by adapting its architecture and function to mechanical stimuli. The ability of bones to adapt to mechanical environment mainly depends on the bone cells, including osteoblasts, osteoclasts and osteocytes.
Osteoblasts, deriving from the MSCs, locate on the surface of cancellous bone and cortical bone. Osteoblasts, the crucial effector cells sensitive to mechanical stimuli, can be activated to promote bone formation. In our experiments, mouse osteoblastic MC3T3-E1 cells were seeded in a flexible cell culture system and were exposed to mechanical stretch of 2000 με at 0.5 Hz. As described in Tang’s study,28 the four-point bending system can supply uniaxial and homogeneous mechanical stretch to the cultured cells. The load magnitude of 2000 με has been shown to induce the osteogenic differentiation of marrow stroma cells (MSCs).41 ALP, Col I and OCN are the major biological markers in osteoblasts differentiation and their expressions were significantly increased in mechanical stretch-loaded cells for 4–12 h in our study. Thus, mechanical stretch can enhance osteogenic gene expressions.
BMPs, potent osteoblastic differentiation factors, play a pivotal role in the regulatory signaling network of bone formation and remodeling. Until now, more than 15 BMP genes have been identified in vertebrates,39 and several BMPs have been demonstrated to induce ectopic bone formation.37 BMP-2 and BMP-4, with 83% similarity in the sequences of amino acids, are the vital members of BMPs families. BMP-2 and BMP-4 have the ability to promote chondrogenesis and osteogenesis and have gained much interest in the field of biomechanics.23 In the present study, both mRNA and protein levels of BMP-2 and BMP-4 were determined and their expressions were remarkably enhanced by mechanical stretch for 4–12 h, while the expressions of BMPs target genes were significantly decreased with Noggin (an inhibitor for BMPs signals) pretreatment in osteoblasts after mechanical loading, which suggest activations of BMP-2 and BMP-4 participate in regulations of osteoblast differentiation in mechanical response. Additionally, we observed an interesting phenomenon: although BMP-2 and BMP-4 expressions started to increase at 4 h after stretch initiation, the elevated levels were not maintained and returned to the basal level at 24 h. Thus, the increased secretion of BMPs after mechanical stretch is not stable. We assumed that the reason for transient expressions of BMPs may be correlated with the duration of mechanical stretch. The loading time, an important parameter of mechanical stretch, can determine the response of osteoblasts. Data suggest the stretch of moderate duration can induce the differentiation of osteoblasts,13 while too short or too long exposure might have the opposite effect.26
The actions of BMPs are well known to be mediated by their receptors and related signaling pathways.10 BMP signals are generally transduced from plasma membrane receptors to the nucleus through both Smad and non-Smad signaling pathways, and are affected by various extracellular and intracellular molecules. BMPs act through a complex serine-threonine receptor mechanism to activate the signal transduction molecules, Smad proteins. The activated Smad proteins then move into the nucleus to modulate the expression of osteoblasts related genes.25 Smad1 and Smad5 are considered to be the major signaling molecules to induce osteoblasts differentiation. Our previous study has reported mechanical stretch activated Smad1 and Smad5 signaling pathways by up-regulating BMP-2 expression.40 Though the role of Smad signaling pathway in osteoblasts differentiation has been well investigated, it is not clear whether the non-Smad pathway is involved in osteoblasts’ mechanical response.
NF-κB signaling is associated with bone metabolism and considered to be the important signal transduction pathway in osteoblasts differentiation.30 It has been demonstrated that NF-κB pathway is involved in pressure-induced BMP-2 expression in endothelial cells.4 NF-κB mainly locates in the cytoplasm in a complex of some inhibitory IκB proteins, including IκBα, IκBβ, IκBγ and IκBε.6 The phosphorylation of IκB proteins is the key point in the regulation of NF-κB pathway in mechanical responses, which enables NF-κB to translocate to the nucleus, where it can activate the target genes. Moreover, p65 protein, one of transcription factors in the NF-κB/Rel family, contains a C-terminal activation domain that is crucial to induce target genes expression in NF-κB pathway. In this study, we found that the expressions of p-IκBα and p-p65 significantly increased in mechanical stretch for 30–90 min, while the expression of IκBα decreased due to its degradation. The data indicate NF-κB signals are activated earlier than BMP-2/BMP-4 in the time course of mechanical response. In addition, we observed that expressions of BMP-2 and BMP-4 remarkably decreased with PDTC (an inhibitor for NF-κB pathway) in osteoblasts after mechanical loading. Hence, we conclude that activation of NF-κB signaling pathway is required for modulating BMPs signals to enhance osteogenic gene expressions during mechanical stretch.
p38MAPK signaling pathway acts as the crucial signal transduction cascades contributing to inflammation, cell-cycle regulation and cell differentiation,3 and the data from some reports indicate that p38 is activated in chondrogenesis and osteoblasts differentiation.14,38 However, the importance of stimulation of p38 activity in osteoblastic maturation in response to load is not clear. To investigate whether p38MAPK pathway is involved in mechanical signal transduction in osteoblasts and its role in regulation of BMP-2 and BMP-4, we first determined the phosphorylation level of p38 (p-p38), the marker in activation of p38MAPK pathway. The results showed that p-p38 expression significantly increased in mechanical stretch for 15–90 min, which suggest p38MAPK signals are activated earlier than NF-κB and BMP-2/BMP-4 in the time course of mechanical response. Furthermore, we found that p38MAPK signals activated by stretch were not influenced by Noggin (an inhibitor for BMPs signals), which indicate p38MAPK pathway is not the downstream signal of BMPs in mechanical response. In addition, we found that expressions of BMP-2 and BMP-4 remarkably decreased with SB203580 (an inhibitor for p38MAPK pathway) in osteoblasts after mechanical loading. Thus, activation of p38MAPK signaling pathway is required for modulating BMPs signals to enhance osteogenic gene expressions during mechanical stretch. Interestingly, we observed the inhibition of p38MAPK pathway can cause the block of NF-κB pathway, which indicate that p38MAPK and NF-κB pathways have cross-talk in regulation of BMPs in mechanical responses. It has been known that p38MAPK and NF-κB both belong to the members of non-Smad signaling of BMPs, and some data suggest p38MAPK could control NF-kB inductions by changes in mechanical environment.7 Findings have revealed p38MAPK pathway was mainly mediated by TGFβ1 activated tyrosine kinase 1 (TAK1), a MAPKKK tyrosine kinase.5 Moreover, TAK1 has been reported to also activate the Jun N-terminal kinase (JNK) and NF-κB signaling pathway.19 Thus, we assumed the interaction between p38MAPK and NF-κB pathways could be mediated by TAK1.
As for the downstream signals of BMP-2/BMP-4 during mechanical stretch, we feel it important to investigate the time course of Smad signals. In our previous study,40 we found Smad1/5 proteins were increased in cells stretched for 4–12 h, and a increase in activated Smad1 (p-Smad1) and Smad5 (p-Smad5), both in whole cell and in nucleus, was observed after mechanical strain for 4 h, which was consistent with changes of BMP-2/BMP-4 expressions in the time course. In addition, protein levels of p-Smad1/5 were attenuated by Noggin. Thus, we conclude that p38 and NF-kB signals are activated first by stretch, and then up-regulate BMP-2/BMP-4 to enhance osteogenic gene expressions through BMPs/Smad signaling pathway (Fig. 9).
In conclusion, our study indicate that activations of p38MAPK and NF-kB signals are both required for up-regulating BMP-2/BMP-4 to enhance osteogenic gene expressions during mechanical stretch, and p38MAPK and NF-kB pathways have cross-talk in regulation of BMPs in mechanical response.
References
Boden, S. D., J. Kang, H. Sandhu, and J. G. Heller. Use of recombinant human bone morphogenetic protein-2 to achieve posterolateral lumbar spine fusion in humans: a prospective, randomized clinical pilot trial: 2002 Volvo Award in clinical studies. Spine 27:2662–2673, 2002.
Canalis, E., A. N. Economides, and E. Gazzerro. Bone morphogenetic proteins, their antagonists, and the skeleton. Endocr. Rev. 24:218–235, 2003.
Coulthard, L. R., D. E. White, D. L. Jones, M. F. McDermott, and S. A. Burchill. p38 (MAPK): stress responses from molecular mechanisms to therapeutics. Trends Mol. Med. 15:369–379, 2009.
Csiszar, A., K. E. Smith, A. Koller, G. Kaley, J. G. Edwards, and Z. Ungvari. Regulation of bone morphogenetic protein-2 expression in endothelial cells: role of nuclear factor-kappaB activation by tumor necrosis factor-alpha, H2O2, and high intravascular pressure. Circulation 111:2364–2372, 2005.
Derynck, R., and Y. E. Zhang. Smad-dependent and Smad-independent pathways in TGF-beta family signalling. Nature 425:577–584, 2003.
Ghosh, S., and M. S. Hayden. New regulators of NF-kB in inflammation. Nat. Rev. Immunol. 8:837–848, 2008.
Granet, C., N. Boutahar, L. Vico, C. Alexandre, and M. H. Lafage-Proust. MAPK and SRC-Kinases control EGR-1 and NF-kB Inductions by changes in mechanical environment in osteoblasts. Biochem. Biophys. Res. Commun. 284:622–631, 2001.
Hamamura, K., M. B. Goldring, and H. Yokota. Involvement of p38 MAPK in regulation of MMP13 mRNA in chondrocytes in response to surviving stress to endoplasmic reticulum. Arch. Oral Biol. 54:279–286, 2009.
Hu, Y., E. Chan, S. X. Wang, and B. Li. Activation of p38 mitogen-activated protein kinase is required for osteoblast differentiation. Endocrinology 144:2068–2074, 2003.
Huang, X. F., and Y. Chai. TGF-β signalling and tooth development. Chin. J Dent. Res. 13:7–15, 2010.
Jimi, E., K. Aoki, H. Saito, F. D. Acquisto, M. J. May, I. Nakamura, T. Sudo, T. Kojima, F. Okamoto, H. Fukushima, K. Okabe, K. Ohya, and S. Ghosh. Selective inhibition of NF-kappa B blocks osteoclastogenesis and prevents inflammatory bone destruction in vivo. Nat. Med. 10:617–624, 2004.
Kanazawa, I., T. Yamaguchi, S. Yano, M. Yamauchi, M. Yamamoto, and T. Suqimoto. Adiponectin and AMP kinase activator stimulate proliferation, differentiation, and mineralization of osteoblastic MC3T3-E1 cells. BMC Cell Biol. 8:51, 2007.
Kaspar, D., W. Seidl, C. Neidlinger-Wilke, A. Ignatius, and L. Claes. Dynamic cell stretching increases human osteoblast proliferation and CICP synthesis but decreases osteocalcin synthesis and alkaline phosphatase activity. J. Biomech. 33:45–51, 2000.
Kawaki, H., S. Kubota, A. Suzuki, M. Suzuki, K. Kohsaka, K. Hoshi, T. Fujii, N. Lazar, T. Ohgawara, T. Maeda, B. Perbal, T. Takano-Yamamoto, and M. Takigawa. Differential roles of CCN family proteins during osteoblast differentiation: Involvement of Smad and MAPK signaling pathways. Bone 49:975–989, 2011.
Khanal, A., I. Yoshioka, K. Tominaga, N. Furuta, M. Habu, and J. Fukuda. The BMP signaling and its Smads in mandibular distraction osteogenesis. Oral Dis. 14:347–355, 2008.
Kohli, S. S., and V. S. Kohli. Role of RANKL-RANK/osteoprotegerin molecular complex in bone remodeling and its immunopathologic implications. Indian J Endocrinol. Metab. 15:175–181, 2011.
Lanyon, L. E., and C. T. Rubin. Static vs dynamic loads as an influence on bone remodeling. J. Biomech. 17:897–905, 1984.
Lanyon, L. E., C. T. Rubin, and G. Baust. Modulation of bone loss during calcium insufficiency by controlled dynamic loading. Calcif. Tissue Int. 38:209–216, 1986.
Lee, S. W., S. I. Han, H. H. Kim, and Z. H. Lee. TAK1-dependent activation of AP-1 and c-Jun N-terminal kinase by receptor activator of NF-kappaB. J. Biochem. Mol. Biol. 35:371–376, 2002.
Lieberman, J. R., A. Daluiski, and T. A. Einhorn. The role of growth factors in the repair of bone. Biology and clinical applications. J. Bone Joint Surg. Am. 84-A:1032–1044, 2002.
Liu, A., and L. A. Niswander. Bone morphogenetic protein signaling and vertebrate nervous system development. Nat. Rev. Neurosci. 6:945–954, 2005.
Mitsui, N., N. Suzuki, M. Maeno, M. Yanagisawa, Y. Koyama, K. Otsuka, and N. Shimizu. Optimal compressive force induces bone formation via increasing bone morphogenetic proteins production and decreasing their antagonists production by Saos-2 cells. Life Sci. 78:2697–2706, 2006.
Miyazono, K., S. Maeda, M. Shin, and T. Imamura. BMP receptor signaling: transcriptional targets, regulation of signals, and signaling cross-talk. Cytokine Growth Factor Rev. 16:251–263, 2005.
Mullender, M., A. J. El Haj, Y. Yang, M. A. van Duin, E. H. Burger, and J. Klein-Nulend. Mechanotransduction of bone cells in vitro: mechanobiology of bone tissue. Med. Biol. Eng. Comput. 42:14–21, 2004.
Noth, U., R. Tuli, R. Seghatoleslami, M. Howard, A. Shah, D. J. Hall, N. J. Hickok, and R. S. Tuan. Activation of p38 and Smads mediates BMP-2 effects on human trabecular bone-derived osteoblasts. Exp. Cell Res. 291:201–211, 2003.
Palma Di, F., M. Douet, C. Boachon, A. Guignandon, S. Peyroche, B. Forest, C. Alexandre, A. Chamson, and A. Rattner. Physiological strains induce differentiation in human osteoblasts cultured on orthopaedic biomaterial. Biomaterials 24:3139–3151, 2003.
Pead, M. J., T. M. Skerry, and J. E. Lanyon. Direct transformation from quiescence to bone formation in the adult periosteum following a single brief period of bone loading. J. Bone Miner. Res. 3:647–656, 1988.
Qi, M. C., J. Hu, S. J. Zou, H. Q. Chen, H. X. Zhou, and L. C. Han. Mechanical strain induces osteogenic differentiation: Cbfa1 and Ets-1 expression in stretched rat mesenchymal stem cells. Int. J. Oral Maxillofac. Surg. 37:453–458, 2008.
Rubin, C. T., and L. E. Lanyon. Regulation of bone formation by applied dynamic loads. J. Bone Joint Surg. Am. 66:397–402, 1984.
Ryu, B., Z. J. Qian, and S. K. Kim. Purification of a peptide from seahorse, that inhibits TPA-induced MMP, iNOS and COX-2 expression through MAPK and NF-kappaB activation, and induces human osteoblastic and chondrocytic differentiation. Chem. Biol. Interact. 184:413–422, 2010.
Schriefer, J. L., S. J. Warden, L. K. Saxon, A. G. Robling, and C. H. Turner. Cellular accommodation and the response of bone to mechanical loading. J. Biomech. 38:1838–1845, 2005.
Sieber, C., J. Kopf, C. Hiepen, and P. Knaus. Recent advances in BMP receptor signaling. Cytokine Growth Factor Rev. 20:343–355, 2009.
Sudo, H., H. A. Kodama, Y. Amagai, S. Yamamoto, and S. Kasai. In vitro differentiation and calcification in a new clonal osteogenic cell line derived from newborn mouse calvaria. J. Cell Biol. 96:191–198, 1983.
Suzuki, J., M. Ogawa, S. Muto, A. Itai, M. Isobe, Y. Hirata, and R. Nagai. Novel IkB kinase inhibitors for treatment of nuclear factor-kB-related diseases. Expert Opin. Investig. Drugs 20:395–405, 2011.
Tang, L. L., Y. L. Wang, J. Pan, and S. X. Cai. The effect of step-wise increased stretching on rat calvarial osteoblast collagen production. J. Biomech. 37:157–161, 2004.
Tanno, M., K. I. Furukawa, K. Ueyama, S. Harata, and S. Motomura. Uniaxial cyclic stretch induces osteogenic differentiation and synthesis of bone morphogenetic proteins of spinal ligament cells derived from patients with ossification of the posterior longitudinal ligaments. Bone 33:475–484, 2003.
Urist, M. R. Osteoinduction in undemineralized bone implants modified by chemical inhibitors of endogenous matrix enzymes. A preliminary report. Clin. Orthop. Relat. Res. 87:132–137, 1972.
Vinals, F., T. Lopez-Rovira, J. L. Rosa, and F. Ventura. Inhibition of PI3 K/p70 S6 K and p38 MAPK cascades increases osteoblastic differentiation induced by BMP-2. FEBS Let. 510:99–104, 2002.
Wan, M., and X. Cao. BMP signaling in skeletal development. Biochem. Biophys. Res. Commun. 328:651–657, 2005.
Wang, L., X. Z. Zhang, Y. Guo, X. Z. Chen, R. X. Li, L. Liu, C. H. Shi, C. Guo, and Y. Zhang. Involvement of BMPs/Smad signaling pathway in mechanical response in osteoblasts. Cell. Physiol. Biochem. 26:1093–1102, 2010.
Wozney, J. M., V. Rosen, A. J. Celeste, L. M. Mitsock, M. J. Whitters, R. W. Kriz, R. M. Hewick, and E. A. Wang. Novel regulators of bone formation: molecular clones and activities. Science 242:1528–1534, 1988.
Acknowledgments
This work was supported by grants from the National Nature Science Foundation of China (No. 10832012).
Conflict of interest
All the authors certify that they have no financial and personal relationship that could inappropriately influence or bias this work.
Author information
Authors and Affiliations
Corresponding author
Additional information
Associate Editor Kent Leach oversaw the review of this article.
Liang Wang and Jian-yu Li contributed equally to this work.
Rights and permissions
About this article
Cite this article
Wang, L., Li, Jy., Zhang, Xz. et al. Involvement of p38MAPK/NF-κB Signaling Pathways in Osteoblasts Differentiation in Response to Mechanical Stretch. Ann Biomed Eng 40, 1884–1894 (2012). https://doi.org/10.1007/s10439-012-0548-x
Received:
Accepted:
Published:
Issue Date:
DOI: https://doi.org/10.1007/s10439-012-0548-x