Abstract
Water is one of the fundamental needs for human life on earth. Different kinds of contaminants that exist in the drinking water may cause serious health issues, affect body organs, or may cause mortality. One of the main sources of biological contaminants in the water is protozoan parasites, which are normally transmitted through the oral-fecal route. Two frequently water born protozoan parasites are C. parvum and G. lamblia. Both of these parasites have different values of refractive index and size. Using these biophysical parameters, the new detection system may be developed for real-time and on-site measurement. In this paper surface plasmon resonance (SPR) technique is utilized for theoretical investigation of biological contamination protozoa in drinking. Based on angle interrogation, the Kretschmann configuration is used to elaborate the concept of the setup. The performance is analysed on the basis of incident light wavelength in visible region and radiative properties of a multi-layered structure are studied using the transfer Metrix method.
Similar content being viewed by others
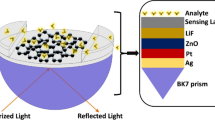
Avoid common mistakes on your manuscript.
1 Introduction
Water is a fundamental need of all living organism on earth. As it's a basic need of life so it should be pure, non-contaminated, and hygienic [1]. Many commercial companies are offering different types of water purification systems, government and non-government oranizations are continuously awaring contryment to use safe drinking water.. Water contamination is of two types biological and non-biological. Numerous non-biological contaminants like silica, sodium, sulfur, ammonia, and chlorine existed in the water. Heavy metals such as cadmium (Cd), lead (Pb), arsenic (As), mercury (Hg), and nickel (Ni) are also found in water as contaminant agents [2]. Benzene, toluene, ethylbenzene, and xylenes are found in water as chemical contamination so they need to be investigated. Boiling, filtering, disinfection with iodine, chlorine, and chlorine dioxide are some common processes to remove bacteria from water [3]. Unfortunately, these processes are not in practice in large section of population. There are a lot of chances for spreading various kinds of contaminated water-generated diseases like asymptomatic infections, nausea, diarrhea, anorexia, uneasiness in the upper intestine, malaise, occasionally low-grade fever and Jaundice, etc. An on-chip immersion refractometer used by Chin et.al. [4] in the year 2011 for biophysical measurement of protozoa. He observed that Cryptosporidium parvum oocysts and Giardia lamblia are two commonly found waterborne protozoan parasites. It was found that both types differ from each other by size, shape, and by their refractive index. C. parvum oocyst is spherical in shape with an ovality of 3–7 μm and refractive index of 1.418 while G. lamblia cyst has ovality of 8–12 μm and refractive index of 1.433 [5]. The optical sensor detect light in specific range and convert it into electronic signals. These sensors detect the change in wavelength, frequency, polarization, and intensity of light [6]. In this paper, the author gives a theoretical investigation of biological contamination protozoa in drinking water using a surface plasmon resonance (SPR) based sensor. SPR sensors can identify the change in the refractive index of the sample. This is label-free detection technique and gives a real-time sensing response that makes it extraordinary with respect to other biosensing techniques like ELISA, RTPCR, etc. [7]. We have calculated various performance indicating parameters like sensitivity, Figure of Merit (FoM), and Detection accuracy. The mathematical concepts and advantages of this technique is also discussed in brief.
2 Proposed sensing structure
Surface plasmons are like electron clouds at the surface of noble metals like gold(Au), Silver(Ag), Copper(Cu), etc. These plasmon get excited in some particular condition and start to resonate this is called surface plasmon resonance (SPR). SPR provides some significant conditions that are highly useful for a sensing points of view. Plasmon excitation can initiated by various methods like using grating, waveguide, and prism. A traditional Kretschmann configuration [8] is used in this paper where a prism is used as a dielectric substrate and at the base of the prism a thin layer of metal is applied. The proposed sensor structure, optical and physical parameters of each layer are given in Fig. 1. When a p-polarized laser light of any particular wavelength is incident at a metal–dielectric interface with total internal reflection(TIR) condition the surface plasmon gets excited and tends to resonate. This action cause to generate a surface plasmon wave (SPW) along the interface. A non-propagating electromagnetic field known as the evanescent field is observed here and is highly sensitive toward the change in the refractive index of the sample under consideration. The value of the evanescent field is maximum where incident light strikes the metal–dielectric interface and at the same time the reflection intensity of the reflected wave is reported as minimum. This results in sharp dip in resonance angle, known as SPR angle (\({\theta }_{\mathrm{spr}}\)). This angle shifts from its position when the incident light angle changes. In general, the prism having a low refractive index like BK7, CaF2, FK51A is used to get a large shift in SPR angle while high refractive index prism-like SF5, ZnSe, H-ZF62 etc. is used to find large dynamic range. The refractive index of the prism can be calculated using the Sellmeier equation and the refractive index of metal estimate using Drude-Lorentz model. Prism increases the momentum of incident light necessary to with momentum of surface plasmon wave which is a key requirement for plasmon excitation [9]. Nanomaterials(2D material) layering over metal have a significant role in sensor performance. It protects of metal layer from corrosion, provide a high surface-to-volume ratio, less electron scattering, and less toxicity towards biomolecules. The aim of functionalizing the conventional structure of the SPR sensor with 2D material is to enhance the performance of the sensor. The only metal layer based structure or conventional structure is not suitable to attach biomolecules and the stability of the thin metal layer in the ambient environment is also an issue. It's observed that the conventional structure shows a sensitivity of 84\(^\circ /{\mathrm{RIU}}\) that is not up to the mark and needs to modify. PtSe2 belongs to the family of Transition metal dichalcogenide (TMDC), its monolayer film thickness is 0.37 nm [10] and monolayer bandgap is 1.2 eV. Similarly, its bilayer (0.74 nm) thickness shows a bandgap of 0.21 eV. This property shows that PtSe2 has thickness-dependent tuneable bandgap properties (exhibit the property of semiconductor to metal). The bandgap modulation property is useful in optoelectronic devices. High electron mobility upto 3000 cm2 V−1 s−1, large electrical conductivity (this is much high than well-known TMDC MoS2, 50–200 cm2 V−1 s−1), low toxicity, and air-stablity makes it a suitable candidate to work as a Bio recognition element (BRE) layer [11]. Some biological process are required to immobilize the ligands on sensor surface so that desired biomolecules may be attached (attachment of antigen with antibody). Silicon (Si) is used as an interlayer between the metal and dielectric medium and an additional MoS2 layer is used before the BRE layer. The aim of including these nanolayers is to enhance the sensitivity by means of increasing the Goos-Hänchen (GH) shift. A 5 nm thick interlayer of silicon (Si) between the metal and dielectric sample work as a nearly guided wave [12]and increases the field intensity of the excitation light at the silicon–sensing layer interface [13]. Enhancement in field intensity helps to enhance the excitation of surface plasmons. The MoS2 belongs to the TMD materials family also called beyond graphene material. It has high light absorption (≈ 5%) and thickness-dependent tunable bandgap. These layers are used to improve the light absorption of the proposed sensor and provide enough excitation energy for effective charge transfer [14]. Therefore, the multilayered structure (Ag/Si/MoS2/PtSe2) is used to achieve significant enhancement in performance of the sensor in comparison to conventional SPR sensors[15].
3 Basic concept of Evanescent wave and transfer matrix method
Let light be launched from one medium to another medium having a refractive index n1 and n2 respectively. Some of its part get reflected from the interface and some refracted. let x direction is parallel to the interface and z is normal to the interface as given in Fig. 2.from Snell's law, we have-
Or \({\theta }_{T}={\mathrm{sin}}^{-1}\left(\frac{{n}_{1}}{{n}_{2}}\mathrm{sin}{\theta }_{I}\right)\)
This shows that \(\mathrm{sin}{\theta }_{I}>\frac{{n}_{2}}{{n}_{1}}\) , \({\theta }_{I}>{\theta }_{C}\), where \({\theta }_{C}\) is a critical angle.
Total internal reflection (TIR) mean incident light beam is completely reflects back into the medium from where it comes. For it, incident light angle must be greater than the critical angle. The ray diagram dictates that:
\(\overrightarrow{K}\) is propagation vector, \(\omega\) is the frequency of light, \({\overrightarrow{E}}_{T}\) is electric field vector amplitude and \({\overrightarrow{E}}_{\mathrm{OT}}\) is a constant and \(\gamma\) is pointing vector. As \({\overrightarrow{E}}_{y}\) is component coming out from the plane so that it can ignored. Splitting Eq. 1 into x and z components we have:
As
So, Eq. 2 will be as:
Since \(\mathrm{sin}{\theta }_{T}>1\) the solution of Eq. 4 gives an imaginary term.
Let \({k}_{T}\mathrm{cos}{\theta }_{T}=Ai\) then Eq. 4 will be:
From Eq. 5, shows damping nature of the wave in the z direction. Using the Eulers formula we have two components real and imaginary. Considering only real terms we have-
This is an expression of evanescent wave which shows that this kind of wave has some amplitude and damping nature. The mathematical expression of surface Plasmon resonance generation is given in Eq. 7. Its clear from this equation that the wavevector of incident light must match to wavevector of the SPR wave [16].
where \({\varepsilon }_{m} and {\varepsilon }_{s}\) are the dielectric constants of the metal and the sensing layers respectively. \({k}_{0}=2\pi /{\lambda }_{0}\) is the wavenumber of the incident light in free space and \({n}_{p}\) is the refractive index of the prism [17]. For calculating the reflectivity of the reflected light of the multilayer model, the transfer matrix method is used. This method is efficient and no approximations are considered. First boundary tangential fields related to the final boundary by the relation given in Eq. 8.
where X1 and Y1 represent the tangential component of electric and magnetic fields respectively at the boundary of the first layer and XN − 1 and YN − 1 are the corresponding field at the nth layer. For p-polarized light the characteristic matrix of the combined structure of the sensor is denoted by Aij.
with
where \({q}_{k}={\left({\mu }_{k}/{\varepsilon }_{k}\right)}^{1/2}\mathrm{cos}{\theta }_{k}\)
One can get the value of reflection coefficient for p polarized light by driving some mathematical steps.
Finally, reflectivity R of given multilayer structure is directly related to reflection coefficient by \(R={\left|{r}_{p}\right|}^{2}\)
4 Result and discussion
An analysis of the performance of the sensor using different kinds of prism with a high refractive index (greater than 1.5) is presented here. Low refractive index prism has the advantage that they are robust, cost-effective, show low scattering behavior and excellent transmission from visible to IR range, but they have a very short dynamic range so it’s difficult to analyze the liquid sample which has a high refractive index (RI) [18]. This paper aims to detect protozoan in drinking water which have major differences in refractive index concerning drinking water RI. Therefore prism analysis is intentionally done with prisms having high RI. Sensitivity is a major factor for sensor. Sensitivity is the ratio of a shift in SPR angle concerning a change in refractive index. From the Table 1 and Fig. 3 its concluded that the sensor using SF5 Prism shows a maximum value of sensitivity of 136.31\(^\circ /{\mathrm{RIU}}\). Although detection accuracy (DA) and figure of merit (FoM) is relatively low in comparison to other prism. There are various method like long-range SPR method(LRSPR) [19] to increase the value of these parameters.
Incident light wavelength also influences the performance of the sensor so its analysis using different wavelengths is also important. The refractive index of each layer depends on the incident light wavelength [20] hence we have centralized our analysis in the visible region and varied the incident light wavelength from 550 to 750 nm. The effect on the performance is shown in Fig. 4a, b and Table 2. It's observed that maximum sensitivity is obtained when the incident light wavelength of 550 nm is used, but the minimum reflectance (Rmin) is obtained at 633 nm wavelength. This shows that at 633 nm wavelength, maximum light is utilized for surface plasmon generation. Moreover, the 633 nm wavelength has the advantage that it shows minimum optical nonlinearity and minimum Kerr effect hence more advantageous for an efficient sensing point of view [7].
Another analysis is given in Table 3. It shows that by increasing the number of layers of PtSe2 up to four layers the maximum sensitivity of 142.42\(^\circ /{\mathrm{RIU}}\) obtained but at the same time value of Rmin increase. The resonance angle becomes more shallow hence FWHM value increases.
It observed from theoretical analysis that the drinking water having G.Lamblia protozoan shows 142.42\(^\circ /{\mathrm{RIU}}\) sensitivity concerning pure water (RI = 1.33) while water contaminated with Cryptosporidium parvum type protozoan exhibit the sensitivity of 117.96\(^\circ /{\mathrm{RIU}}\). The graphical representation of the reflectance curve is shown in this Fig. 5. The work is theoretical and its expected that in future this can help in the area of optical sensing. At the end of this paper authors want to acknowledge some recent and notable waork related to optical sensing [21,22,23,24].
5 Conclusion
Theoretical study and numerical simulation of SPR based sensor for protozoan detection in drinking water carried out. Two different types of protozoan G. Lamblia and C. Parvum is detected and for each case sensitivity is calculated.An angle interrogation based on Kretschmann configuration is used for plasmon excitation and some additional nanolayer of Si, MoS2 and PtSe2 is used with conventional structure for performance enhancement. The proposed sensor is driven by different incident light wavelengths and at each wavelength performance is analyzed. Several types of high refractive index prism are taken and for each case performance parameters are estimated. Maximum sensitivity obtained as 142.42\(^\circ /\mathrm{RIU}\) and 117.96\(^\circ /\mathrm{RIU}\) for G. Lamblia and C. Parvum respectively.
References
Nemati, S., Shalileh, F., Mirjalali, H., Omidfar, K.: Toward waterborne protozoa detection using sensing technologies. Front. Microbiol. 14, 363 (2023)
Erickson, T.A., Nijjar, R., Kipper, M.J., Lear, K.L.: Characterization of plasma-enhanced teflon AF for sensing benzene, toluene, and xylenes in water with near-IR surface plasmon resonance. Talanta 119, 151–155 (2014)
Sharma, S., Bhattacharya, A.J.A.W.S.: Drinking water contamination and treatment techniques. Appl. Water Sci. 7(3), 1043–1067 (2017)
Chin, L. K., Ayi, T. C., Yap, P. H., & Liu, A. Q.: Null-method in immersion refractometry for biophysical measurement of cryptosporidium and giardia lamblia. In: 15th International Conference on Miniaturized Systems for Chemistry and Life Sciences 2011, pp. 707–709. MicroTAS 2011 (2011).
Kang, C., Lee, S., Park, T., Sim, S.: Performance enhancement of real-time detection of protozoan parasite, Cryptosporidium oocyst by a modified surface plasmon resonance (SPR) biosensor. Enzyme Microb. Technol. 39, 387–390 (2006). https://doi.org/10.1016/j.enzmictec.2005.11.039
Rajan, G.: Introduction to optical fiber sensors. In: Optical Fiber Sensors, pp. 1–12. CRC Press, Boca Raton (2017)
Singh, S.K., Srivastava, A., Dwivedi, L.K.: A Theoretical analysis of Milk adulteration/contamination detection in camel, buffalo and cow milk using SPR Technique. J. Phys. Confer. Ser. 2426(1), 012040 (2023)
Srivastava, A., Prajapati, Y.K.: Surface plasmon resonance (SPR)-based biosensor using MXene as a BRE layer and magnesium oxide (MgO) as an adhesion layer. J. Mater. Sci. Mater. Electr. 1–10 (2021)
Liu, Z., Lu, F., Jiang, L., Lin, W., Zheng, Z.: Tunable Goos–Hänchen shift surface plasmon resonance sensor based on graphene-hBN heterostructure. Biosensors 11(6), 201 (2021)
Shi, J., Huan, Y., Hong, M., Xu, R., Yang, P., Zhang, Z., et al.: Chemical vapor deposition grown large-scale atomically thin platinum diselenide with semimetal–semiconductor transition. ACS Nano 13(7), 8442–8451 (2019)
Minev, N., Buchkov, K., Dikov, H., Videva, V., Avramova, I., Rafialov, P., et al.: Properties analysis of 2D PtSe2 layers grown by thermally assisted conversion of chemical vapor deposition. In: 2021 XXX International Scientific Conference Electronics (ET), pp 1–4. IEEE (2021).
Pal, S., Verma, A., Saini, J.P., Prajapati, Y.K.: Sensitivity enhancement using silicon-black phosphorus-TDMC coated surface plasmon resonance biosensor. IET Optoelectron. 13(4), 196–201 (2019)
Lahav, A., Auslender, M., Abdulhalim, I.: Sensitivity enhancement of guided-wave surface- plasmon resonance sensors. Opt. Lett. 33(21), 2539–2541 (2008)
Ouyang, Q., Zeng, S., Dinh, X.Q., Coquet, P., Yong, K.T.: Sensitivity enhancement of MoS2 nanosheet based surface plasmon resonance biosensor. Procedia Eng. 140, 134–139 (2016)
Suvarnaphaet, P., Pechprasarn, S.: Graphene-based materials for biosensors:a review. Sensors 17(10), 2161 (2017)
Homola, J.: Present and future of surface plasmon resonance biosensors. Anal. Bioanal. Chem. 377, 528–539 (2003)
Srivastava, A., Verma, A., & Prajapati, Y.K.: Effect of 2D, TMD, perovskite, and 2D transition metal carbide/nitride materials on performance parameters of SPR biosensor. In: Handbook of Nanomaterials for Sensing Applications, pp. 57–90. Elsevier (2021).
Canning, J., Qian, J., Cook, K.: Large dynamic range SPR measurements using a ZnSe prism. Photonic Sens. 5, 278–283 (2015)
Srivastava, A., Prajapati, Y.K.: Effect of sulfosalt and polymers on performance parameter of SPR biosensor. Opt. Quant. Electron. 52(10), 440 (2020)
Pandey, P.S., Raghuwanshi, S.K., Kumar, S.: Recent advances in two-dimensional materials-based Kretschmann configuration for SPR sensors: a review. IEEE Sens. J. 22(2), 1069–1080 (2021)
Pandey, P. S., Raghuwanshi, S. K., Singh, R., Kumar, M., Kumar, S.: Performance enhancement of surface plasmon resonance biosensor based on prism with Kretschmann configuration assisted by 2D materials. In: 2D Photonic Materials and Devices VI, Vol. 12423, pp. 52–58. SPIE (2023)
Pandey, P. S., Raghuwanshi, S. K., Awasthi, S., Shadab, A., Ansari, M. T. I., Kumar, S.: Sensitivity enhancement of the surface plasmon resonance sensor with nobel structure based on PtSe2 and 2D materials. In: Quantum Sensing and Nano Electronics and Photonics XIX, Vol. 12430, pp. 298–303. SPIE (2023)
Pandey, P.S., Raghuwanshi, S.K., Singh, R., Kumar, S.: Surface plasmon resonance biosensor chip for human blood groups identification assisted with silver-chromium-hafnium oxide. Magnetochemistry 9(1), 21 (2023)
Pandey, P.S., Raghuwanshi, S.K.: Sensitivity enhancement of surface plasmon resonance (SPR) sensor assisted by BlueP/MoS 2 based composite heterostructure. IEEE Access 10, 116152–116159 (2022)
Author information
Authors and Affiliations
Corresponding author
Ethics declarations
Conflict of interest
The author has no conflict of interest.
Additional information
Publisher's Note
Springer Nature remains neutral with regard to jurisdictional claims in published maps and institutional affiliations.
Rights and permissions
Springer Nature or its licensor (e.g. a society or other partner) holds exclusive rights to this article under a publishing agreement with the author(s) or other rightsholder(s); author self-archiving of the accepted manuscript version of this article is solely governed by the terms of such publishing agreement and applicable law.
About this article
Cite this article
Singh, S.K., Srivastava, A., Dwivedi, L.K. et al. Detection of biological contamination protozoa in drinking water using surface plasmon resonance-based technique. Opt Rev 30, 478–484 (2023). https://doi.org/10.1007/s10043-023-00823-x
Received:
Accepted:
Published:
Issue Date:
DOI: https://doi.org/10.1007/s10043-023-00823-x