Abstract
African swine fever virus (ASFV) is one of the most devastating diseases of domestic pigs for which no effective vaccines are available. Flavonoids, natural products isolated from plants, have been reported to have significant in vitro and in vivo antiviral activity against different viruses. Here, we tested the antiviral effect of five flavonoids on the replication of ASFV in Vero cells. Our results showed a potent, dose-dependent anti-ASFV effect of apigenin in vitro. Time-of-addition experiments revealed that apigenin was highly effective at the early stages of infection. Apigenin reduced the ASFV yield by more than 99.99 % when it was added at 1 hpi. The antiviral activity of apigenin was further investigated by evaluation of ASFV protein synthesis and viral factories. This flavonoid inhibited ASFV-specific protein synthesis and viral factory formation. ASFV-infected cells continuously treated with apigenin did not display a cytopathic effect. Further studies addressing the use of apigenin in vivo are needed.
Similar content being viewed by others
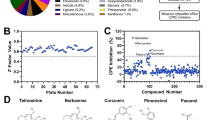
Avoid common mistakes on your manuscript.
Introduction
African swine fever virus (ASFV), the only member of the family Asfarviridae, is a large enveloped DNA virus with an icosahedral morphology [31]. The virulent ASFV isolates cause an acute hemorrhagic fever in domestic pigs. Pigs usually die in less than 10 days after infection. Due to the lack of effective vaccines, African swine fever (ASF) is considered one of the most important and devastating viral diseases of domestic pigs. Recent outbreaks reported in Trans-Caucasus countries and Eastern Europe highlight the urgent need to develop effective vaccines or antiviral drugs against ASFV.
Plants and plant-derived compounds are regarded as an important source for the discovery and development of new antiviral agents because they produce few side effects and are readily accessible in nature [22, 28]. Flavonoids are a group of plant secondary metabolites found in vegetables, fruits, herbs, seeds, and nuts, as well as in red wine and tea [29]. They consist of two benzene rings linked via a heterocyclic pyrene ring (Fig. 1a). Flavonoids are usually divided into a variety of classes, such as flavones, flavonols and flavanones. A great number of biological effects have been reported for flavonoids. For example, apigenin exerts anti-cancer activity by targeting the PI3K/Akt/mTOR signaling pathways and inducing autophagic cell death [30, 37]. Many flavonoids have been shown to have antiviral activity against a wide range of viruses [4, 10]. Apigenin was shown to inhibit RNA viruses, including enterovirus 71, hepatitis C virus and chikungunya virus [21, 27, 38]. Some reports have shown that catechin was able to reduce the infectivity of hepatitis C virus and influenza A and B viruses [18, 34]. Antiviral activity against Lassa virus and Ebola virus has been shown for genistein [19, 32]. Luteolin and quercetin have antiviral activity against enterovirus 71, Japanese encephalitis virus, and dengue virus type 2 [16, 33, 36].
Chemical structure of flavonoids and cytotoxicity to Vero cells. (a) The basic flavonoid structure (1) and different distribution of hydroxyl groups located on the flavonoid rings of apigenin (2), catechin (3), genistein (4), luteolin (5), and quercetin (6). (b) Graphs showing cell viability determined after 96 h treatment with different concentrations of flavonoids. (c) Graphs showing the CC50 value for each compound determined by a linear regression analysis. Values represent mean and standard deviation results from three independent experiments
There are currently no data available on the antiviral activity of flavonoids against ASFV. Here, we tested the in vitro anti-ASFV effect of apigenin, catechin, genistein, luteolin and quercetin (Fig. 1a). For the most active flavonoid, we identified the potential target stages of ASFV infection.
Materials and methods
Cells, compounds and virus
Vero cells were maintained and propagated at 37 °C in Eagle’s minimum essential medium (EMEM) (Lonza, Belgium) supplemented with 10 % of fetal bovine serum (FBS) (Sigma-Aldrich, Germany), 2 mM L-glutamine (Lonza, Belgium), and100 IU of penicillin (Sigma-Aldrich, Germany) and 100 μg of streptomycin (Sigma-Aldrich, Germany) per ml.
Two flavonoids, apigenin and genistein, were purchased from Molekula (UK). Three other flavonoids, catechin, luteolin and quercetin, were purchased from Cayman Chemical (USA). The purity of all flavonoids was ≥95 %. The compounds were dissolved in dimethylsulfoxide (DMSO) (Sigma-Aldrich, Germany) at a stock concentration of 20 mM and stored at 4 °C. At the time of experiments, dilutions in cell culture medium were performed with the final concentration of DMSO not exceeding 1 % (v/v).
The Vero-adapted BA71V isolate was propagated and titrated by cytopathic effect (CPE-based) assay on Vero cells. The titer was calculated by Reed-Münch endpoint calculation method, as described previously [3]. The viral titer was expressed as log 50 % tissue culture infective dose (TCID50).
Cytotoxicity assay
The cytotoxicity of flavonoids was evaluated by the crystal violet staining method, as described previously [26]. Briefly, confluent Vero cells in 96-well cell culture plates were treated with flavonoids at final concentrations ranging from 31.25 to 500 mM. The treated cells were incubated for 96 hours at 37 °C in 5 % CO2. After incubation, the medium was removed and cells were stained with 0.4 % crystal violet solution in ethanol for 30 minutes and carefully washed with distilled water. Colorimetric measurements were done by a microplate reader at 590 nm. The percentage of viable cells was calculated for each concentration as [(ODT/ODC) × 100], where ODT and ODC are the absorbance of treated and control cells, respectively. The 50 % cell cytotoxicity (CC50) was determined as the concentration of a compound that causes 50 % cell death and calculated by a linear regression analysis of dose-response curves generated from the data. The cytotoxicity of the final concentration of DMSO (1 %) was also measured.
Antiviral assays
For primary screening, Vero cells were grown in a 96-well plate (5 × 103 cell/well) in EMEM containing 10 % FBS for 24 h at 37 °C in 5 % CO2. The tested compounds were added together with 5 × 103 TCID50/well of ASFV per well. The infection was allowed to proceed for 96 h, and virus was then collected and titrated by CPE-based assay.
For time-of-addition assays, Vero cells were seeded in 96-well plate (5 × 103 cells/well) in EMEM containing 10 % FBS and incubated for 24 h at 37 °C in 5 % CO2. The wells were designated as -1, 0, 1, 4, 8, 12 h, according to the time of ASFV infection. For -1 h before infection, Vero cells were treated with the tested compound for 1 h (pre-treatment assay). After 1 h (at 0 h), each well was infected with 5 × 103 TCID50 of ASFV. The tested compound was then added to the 0-, 1-, 4-, 8- and 12-h wells. The plate was then incubated at 37 °C in 5 % CO2 for 96 h. The supernatant was collected and used in the CPE-based assay.
Anti-entry assays
For the binding assay, Vero cells in a 96-well plate were incubated with 5 ×103 TCID50 of ASFV per well and tested compound at 4 °C for 1 h to permit binding but prevent viral internalization. Unbound virus and flavonoid were then removed by washing with cold PBS, and EMEM containing 10 % FBS was added. The plate was then switched to 37 °C and incubated in 5 % CO2 for 96 h.
For the internalization assay, Vero cells in 96-well plate were incubated with 5 ×103 TCID50 of ASFV per well at 4 °C for 1 h. Unbound virus was then discarded, and EMEM containing 10 % FBS was added. The temperature was shifted to 37 °C to allow virus entry to proceed. The tested compound was added at 0 h and removed at 1 h following the temperature shift. The time point when cells were shifted to 37 °C was considered 0 h. After 96 h, the virus titer was determined.
Continuous treatment assay
Vero cells were grown in 6-well plate to about 2 × 105 cells/well and infected with 105 TCID50 of ASFV per well. At 1 h postinfection (hpi), cells were washed with PBS and incubated in EMEM supplemented with the tested flavonoid at different concentrations and 2 % FBS. The medium was replaced by fresh medium with flavonoid every 24 h during 144 h of infection. After six days of treatment, supernatants were used for titration and reinfection of fresh cells. The presence of ASFV in continuously treated cells was investigated by conventional PCR and ELISA.
Detection of ASFV by PCR and ELISA
Total DNA was extracted from treated and mock-treated cells at 144 h of infection using a commercial kit (ArchivePure DNA Cell/Tissue Kit, 5 Prime, Germany). The conventional PCR of the viral p72 gene was performed by adding 2 μl of extracted DNA to a PCR mix containing 16.3 μl of distilled water, 2.5 μl of PCR buffer (10X), 2.5 μl of MgCl2 (25 mM), 0.5 of μl dNTPs (10 mM), 0.125 μl of Taq polymerase, and 0.5 μl of each primer (20 μM), forward p72U (5’-GGCACAAGTTCGGACATGT-3’) and reverse p72D (5’-GTACTGTAACGCAGCACAG-3’) [1]. Thermal cycling conditions were as follows: 95 °C for 10 min, 40 cycles of denaturation at 95 °C for 30 s, annealing at 55 °C for 1 min, and elongation at 72 °C for 1 min, followed by an extra elongation step at 72 °C for 10 min [1]. The amplification product (478 bp) was analyzed by 2 % agarose gel electrophoresis.
For ELISA experiment, the cell culture supernatant was collected at 144 h of infection. Then, the product of the viral p72 gene (VP72 protein) was detected using commercially available ELISA kit (Ingenasa, Spain) and a colorimetric reader. The optical density (OD) was measured at 405 nm (OD405). Samples with an OD higher than 0.3 were considered positive for ASFV according to the manufacturer’s protocol.
Western blotting
To analyze the effect of apigenin on viral protein synthesis, ASFV-infected cells (5 × 105 TCID50/flask) were incubated for 48 h in the absence or presence of tested flavonoid. The infected cells were lysed in TNT buffer (20 mM Tris-HCl, 200 mM NaCl, 1 % Triton X-100) supplemented with protease inhibitor cocktail (Sigma-Aldrich, Germany). Proteins (40 μg) were subjected to 12 % SDS-PAGE electrophoresis and then transferred onto a nitrocellulose membrane (Sigma-Aldrich, Germany). The blot membranes were incubated with primary polyclonal antibodies (a kind gift from Dr. Y. Revilla) against ASFV BA71V proteins [5] and treated with horseradish-peroxidase-conjugated secondary antibodies (goat anti-rabbit; Santa Cruz Biotechnology, USA) followed by autoradiography.
Detection of viral factories
Cells were grown on coverslips and infected with 105 TCID50 of virus. After 1 hpi, cells were washed with PBS and incubated in EMEM supplemented with the most effective flavonoid at different concentrations and 10 % FBS. At 16 hpi, cells were fixed in 96 % ethanol for 30 min and stained with Schiff’s reagent (DNA hydrolysis in 5 N hydrochloric acid for 60 min at 22 °C) by the method of Feulgen [17]. Then, the number of viral factories were counted in treated and untreated cells in a total of 103 cells per sample. The DNA content of viral factories was measured by computer-equipped microscope-cytometer SMP 05 (Carl Zeiss, Germany) at 575 nm and expressed as integrated optical density (IOD), the cytometric equivalent of DNA content. The measurement was carried out for 100 viral factories per sample.
Statistics
All experiments were performed in triplicate. Data are expressed as mean ± SD and were analyzed for significance using Student’s t-test. P < 0.05 was considered to be statistically significant.
Results
Cytotoxicity of flavonoids
Different concentrations at which flavonoids may exhibit cytotoxic activity on Vero cells were tested. After treatment for 96 h- which was the same duration used for antiviral assays, the percent of viable cells was determined using the crystal violet staining method. All flavonoids had a cytotoxic effect at 250 and 500 μM concentrations (Fig. 1b). In contrast, flavonoids at 31.25 and 62.5 μM had little or no effect on Vero cells. The calculation of CC50 showed that luteolin with a CC50 of 212.1 ± 11.5 μM is the most cytotoxic flavonoid for Vero cells (Fig. 1c). Meanwhile, catechin with a CC50 of 450.2 ± 6.9 μM, had the least cytotoxic effect. Cells treated with 1 % DMSO did not display cytotoxic changes (data not shown).
Antiviral activity of tested compounds against ASFV
For primary screening, Vero cells were treated with flavonoids at the following non-cytotoxic concentrations: 25 μM for luteolin, 50 μM for apigenin and 100 μM for genistein, quercetin and catechin. To test the antiviral activity of flavonoids, the collected virus was titrated using a CPE-based assay. It was shown that, of the analyzed compounds, only apigenin was able to significantly reduce (P < 0.02) the viral titer in AFSV-infected cells (Fig. 2a). This reduction occurred in a dose-dependent manner (Fig. 2b).
Effect of flavonoids on ASFV yield in different antiviral effect assays. Virus yield in (a) primary screening, (b) dose-dependence, (c) time-of-addition, and (d) entry assays. In the primary screening assay, cells were treated with luteolin (25 μM), apigenin (50 μM), genistein (100 μM), quercetin (100 μM) and catechin (100 μM). In the remaining assays, cells were treated with apigenin (50 μM). Values represent mean and standard deviation results from three independent experiments
Apigenin was selected for a time-of-addition assay conducted with aim of determining which stage of ASFV infection this flavonoid affected. As shown in Fig. 2c, the inhibition was most efficient when apigenin was added at 1 hpi. Apigenin decreased the viral titer from 5.5 log TCID50/ml (control) to 2.2 log TCID50/ml (P < 0.01), reducing the ASFV yield by more than 99.99 %. Similar results were observed when apigenin was added at -1, 0 and 4 hpi. In contrast, apigenin showed slight or no significant inhibitory effect on ASFV replication when it was added at 8 and 12 hpi. The solvent DMSO has no effect on ASFV at non-toxic concentrations [15].
To determine whether apigenin can inhibit ASFV binding to Vero cells, apigenin was added in a binding assay at 4 °C, conditions under which ASFV binds to but does not enter cells. Apigenin had no effect on virus replication when added during the period of virus attachment (Fig. 2d). Furthermore, as previous studies have reported that ASFV is internalized within 1 h [15], apigenin was added after virus attachment and removed 1 h later to determine whether it acts at the internalization stage of infection. This experiment did not reveal any significant activity against internalization of attached ASFV to Vero cells (Fig. 2d).
Effect of continuous treatment
To determine whether the prolonged treatment of ASFV-infected cells with apigenin may eliminate the virus from cell culture, different concentrations of apigenin were added continuously to Vero cells from 1 h after infection up to 144 hpi. After continuous treatment, ASFV-infected cells were observed microscopically. As shown in Fig. 3a, no ASFV-associated CPE was observed in cells treated with apigenin at 25 μM and 50 μM concentrations. In contrast, the complete CPE was seen in cells treated with 12.5 μM apigenin. The viral titer in supernatants collected from cells exposed to 25 μM and 50 μM apigenin was less than the detection limit of the CPE-based assay (Fig. 3b). Therefore, these supernatants were also tested by ELISA to detect VP72 antigen. ELISA analysis showed that both supernatants were negative to ASFV because their OD values were less than 0.3 (Table 1). Then, PCR amplification of the p72 gene was performed to detect the presence of viral DNA in apigenin-treated cells. As shown in Fig. 3c, the amount of p72 gene PCR product (478 bp) decreased when apigenin was added at 25 μM and 50 μM concentrations. However, Vero cells cultivated with supernatants collected from 25 μM and 50 μM treatments did not demonstrate CPE after a 7-day cultivation (data not shown).
Effect of continuous treatment on ASFV infection. (a) Inhibition of ASFV-associated CPE in Vero cells after 144 h of treatment. Uninfected Vero cells were used as a control (1), whereas ASFV-infected but untreated cells were used as a negative control (2). Vero cells treated with apigenin at 25 μm and 50 μm concentrations (3 and 5, respectively) showed no CPE, in contrast to 12.5 μm treatment, where complete CPE was seen (4). (b) The viral titer of supernatants collected from continuous treatment experiments. (c) PCR amplification of the viral p72 gene isolated from ASFV-infected cells exposed to apigenin treatment
Effect of apigenin on viral protein synthesis
Infection was allowed to proceed for 48 h, a time point at which most of the cells became infected with ASFV. Then, western blot analysis was used to compare the synthesis of viral proteins between ASFV-infected Vero cells that were exposed or not exposed to apigenin. As shown in Fig. 4a, both early (p32) and late (p72) ASFV-specific proteins were detected in control ASFV-infected cells. In contrast, the presence of apigenin at a concentration of 50 μM resulted in the inhibition of early and late virus-specific proteins. Apigenin-treated cells also did not display expression of the 25-kDa viral protein.
ASFV-specific protein synthesis and formation of viral factories in apigenin-treated cells. (a) Early and late protein expression (indicated by arrows) in cells with and without apigenin treatment. (b) An ASFV factory (indicated by an arrow) stained by the Feulgen method. (c) Graphs showing the percentage of viral factories at different concentrations of apigenin. (d) Graphs showing the DNA content of viral factories at different concentrations of apigenin
Effect of apigenin on viral factories
As viral factories contain newly synthesized viral DNA, the Feulgen method allowed the identification of ASFV factories by light microscopy. ASFV-infected Vero cells showed a compact accumulation of viral DNA close to the nucleus (Fig. 4b). This result coincided with those of previous studies demonstrating the perinuclear localization of ASFV factories [7, 13]. Apigenin at 12.5 μM, 25 μM and 50 μM concentrations decreased the number of viral factories by 44 %, 60 % and 74 %, respectively (Fig. 4c). These factories contained significantly less DNA (P < 0.05) than ASFV factories in mock-treated cells (Fig. 4d).
Discussion
Since its re-introduction onto the European continent in 2007, ASFV has caused significant problems for both affected countries and neighboring ones, including countries of the European Union [25]. Considering the negative effects on porcine production and the absence of vaccines, the search for effective antiviral compounds is an urgent need [35]. Several agents that affected ASFV infection have been reported previously. For example, sulfated polysaccharides were shown to inhibit ASFV attachment to the host cell [8]. Plant extracts from marine microalgae such as Pophyridium cruentum and Ellipsoidon sp. were shown to produce a significant inhibition of the in vitro replication of ASFV in dose-dependent manner, possibly due to sulfated polysaccharides [6]. Polyphenolic phytoalexins, such as resveratrol and oxyresveratrol were shown to reduce ASFV production by inhibiting viral DNA replication, late viral protein synthesis, and viral factory formation [7].
Flavonoids constitute a large family of plant-derived compounds with various biological properties. Some of them demonstrate antiviral activity by interfering with the viral replication cycle. During productive infection, the viral replication cycle consists of virion attachment and internalization, viral protein synthesis and genome replication, assembly and shedding. The inhibition of any of these stages can be considered a potential target for flavonoids. For example, (-)-epigallocatechin-3-gallate acts as the inhibitor of viral attachment and entry [2, 11]. Apigenin inhibits foot-and-mouth disease virus at the post-treatment stage by interfering with the translation activity of viral genes [23]. Catechin and naringenin have the ability to inhibit hepatitis C virus assembly [18].
This study represents the first report on the antiviral activity of flavonoids against ASFV. Initially, we tested five flavonoids belonging to different structural families. The primary screening of flavonoids demonstrated that apigenin was the most active antiviral flavonoid among the tested compounds. This flavonoid did not exert antiviral effect on ASFV attachment and internalization into the host cells. However, the results of the time-of-addition assay suggested that the addition of apigenin was highly effective at early stages of infection but less effective at 8 hpi and ineffective at 12 hpi. Western blotting detected a significant reduction of early (p32) and late (p72) protein expression in cells treated with 50 μM apigenin. We also studied the formation of viral factories in cells treated with different concentrations of apigenin. All concentrations reduced the number of ASFV factories. When apigenin was continuously added to ASFV-infected cells, the virus became undetectable by CPE-based assay and ELISA.
Although it is difficult to be definitive regarding how apigenin inhibits ASFV infection, our results suggest that it interferes with the early stages of the viral life cycle. After entry by endocytosis or macropinocytosis, ASFV early genes such as C204L encoding p32 are expressed as early as 1 hpi [20, 24]. It is possible that some of the early proteins of ASFV represent a direct target for apigenin. Previous studies have demonstrated the importance of p32 in early stages of ASFV infection [9]. Furthermore, p32 interacts with cellular hnRNP-K, thereby reducing cellular transcriptional activity [14]. Therefore, the downregulation of p32 synthesis by apigenin may have effects on ASFV replication. On the other hand, apigenin can inhibit ASFV infection indirectly through activation of cellular factors such as autophagosomes. Since autophagy has been shown to reduce the number of ASFV-infected cells, and since apigenin has autophagy-inducing effects, its induction may account for the antiviral activity of apigenin against ASFV [12, 37].
In conclusion, this study represents an important first report on the anti-ASFV properties of flavonoids. Our findings suggest that apigenin has an inhibitory effect on ASFV replication, interfering with early steps of infection. These results indicate that apigenin may be a potential candidate as an antiviral drug for ASFV infection. Therefore, further studies addressing the use of apigenin in vivo are required.
References
Bastos AD, Penrith ML, Crucière C, Edrich JL, Hutchings G, Roger F, Couacy-Hymann E, Thomson RG (2003) Genotyping field strains of African swine fever virus by partial p72 gene characterisation. Arch Virol 148:693–706. doi:10.1007/s00705-002-0946-8
Calland N, Albecka A, Belouzard S, Wychowski C, Duverlie G, Descamps V, Hober D, Dubuisson J, Rouillé Y, Séron K (2012) (-)-Epigallocatechin-3-gallate is a new inhibitor of hepatitis C virus entry. Hepatology 55:720–729. doi:10.1002/hep.24803
Carrascosa AL, Bustos MJ, de Leon P (2011) Methods for growing and titrating African swine fever virus: field and laboratory samples. Curr Protoc Cell Biol Chapter 26, Unit 26.14
Chiang LC, Chiang W, Liu MC, Lin CC (2003) In vitro antiviral activities of Caesalpinia pulcherrima and its related flavonoids. J Antimicrob Chemother 52:194–198. doi:10.1093/jac/dkg291
del Val M, Viñuela E (1987) Glycosylated components induced in African swine fever (ASF) virus-infected Vero cells. Virus Res 7:297–308. doi:10.1016/0168-1702(87)90044-X
Fabregas J, García D, Fernandez-Alonso M, Rocha AI, Gómez-Puertas P, Escribano JM, Otero A, Coll JM (1999) In vitro inhibition of the replication of haemorrhagic septicaemia virus (VHSV) and African swine fever virus (ASFV) by extracts from marine microalgae. Antiviral Res 44:67–73. doi:10.1016/S0166-3542(99)00049-2
Galindo I, Hernáez B, Berná J, Fenoll J, Cenis JL, Escribano JM, Alonso C (2011) Comparative inhibitory activity of the stilbenes resveratrol and oxyresveratrol on African swinefever virus replication. Antiviral Res 91:57–63. doi:10.1016/j.antiviral.2011.04.013
García-Villalón D, Gil-Fernández C (1991) Antiviral activity of sulfated polysaccharides against African swine fever virus. Antiviral Res 15:139–148. doi:10.1016/0166-3542(91)90031-L
Gómez-Puertas P, Rodríguez F, Oviedo JM, Brun A, Alonso C, Escribano JM (1998) The African swine fever virus proteins p54 and p30 are involved in two distinct steps of virus attachment and both contribute to the antibody-mediated protective immune response. Virology 243:461–471. doi:10.1006/viro.1998.9068
Ha SY, Youn H, Song CS, Kang SC, Bae JJ, Kim HT, Lee KM, Eom TH, Kim IS, Kwak JH (2014) Antiviral effect of flavonol glycosides isolated from the leaf of Zanthoxylum piperitum on influenza virus. J Microbiol 52:340–344. doi:10.1007/s12275-014-4073-5
Helfer M, Koppensteiner H, Schneider M, Rebensburg S, Forcisi S, Müller C, Schmitt-Kopplin P, Schindler M, Brack-Werner R (2014) The root extract of the medicinal plant Pelargonium sidoides is a potent HIV-1 attachment inhibitor. PLoS One 9:e87487. doi:10.1371/journal.pone.0087487
Hernaez B, Cabezas M, Muñoz-Moreno R, Galindo I, Cuesta-Geijo MA, Alonso C (2013) A179L, a new viral Bcl2 homolog targeting Beclin 1 autophagy related protein. Curr Mol Med 13:305–316. doi:10.2174/156652413804810736
Hernaez B, Escribano JM, Alonso C (2006) Visualization of the African swine fever virus infection in living cells by incorporation into thevirus particle of green fluorescent protein-p54 membrane protein chimera. Virology 350:1–14. doi:10.1016/j.virol.2006.01.021
Hernaez B, Escribano JM, Alonso C (2008) African swine fever virus protein p30 interaction with heterogeneous nuclear ribonucleoprotein K (hnRNP-K) during infection. FEBS Lett 582:3275–3280. doi:10.1016/j.febslet.2008.08.031
Hernaez B, Alonso C (2010) Dynamin- and clathrin-dependent endocytosis in African swine fever virus entry. J Virol 84:2100–2109. doi:10.1128/JVI.01557-09
Johari J, Kianmehr A, Mustafa MR, Abubakar S, Zandi K (2012) Antiviral activity of baicalein and quercetin against the Japanese encephalitis virus. Int J Mol Sci 13:16785–16795. doi:10.3390/ijms131216785
Karalova EM, Sargsyan KhV, Hampikian GK, Voskanyan HE, Abroyan LO, Avetisyan AS, Hakobyan LA, Arzumanyan HH, Zakaryan HS, Karalyan ZA (2011) Phenotypic and cytologic studies of lymphoid cells and monocytes in primary culture of porcine bone marrow during infection of African swine fever virus. In Vitro Cell Dev Biol Anim 47:200–204. doi:10.1007/s11626-010-9380-5
Khachatoorian R, Arumugaswami V, Raychaudhuri S, Yeh GK, Maloney EM, Wang J, Dasgupta A, French SW (2012) Divergent antiviral effects of bioflavonoids on the hepatitis C virus life cycle. Virology 433:346–355. doi:10.1016/j.virol.2012.08.029
Kolokoltsov AA, Adhikary S, Garver J, Johnson L, Davey RA, Vela EM (2012) Inhibition of Lassa virus and Ebola virus infection in host cells treated with the kinase inhibitors genistein and tyrphostin. Arch Virol 157:121–127. doi:10.1007/s00705-011-1115-8
Miguel Ángel CG, Chiappi M, Galindo I, Barrado-Gil L, Muñoz-Moreno R, Carrascosa JL, Alonso C (2015) Cholesterol flux is required for endosomal progression of African swine fever virions during the initial establishment of infection. J Virol 90:1534–1543. doi:10.1128/JVI.02694-15
Murali KS, Sivasubramanian S, Vincent S, Murugan SB, Giridaran B, Dinesh S, Gunasekaran P, Krishnasamy K, Sathishkumar R (2015) Anti-chikungunya activity of luteolin and apigenin rich fraction from Cynodon dactylon. Asian Pac J Trop Med 8:352–358. doi:10.1016/S1995-7645(14)60343-6
Potterat O, Hamburger M (2008) Drug discovery and development with plant-derived compounds. Prog Drug Res 65:47–118
Qian S, Fan W, Qian P, Zhang D, Wei Y, Chen H, Li X (2015) Apigenin restricts FMDV infection and inhibits viral IRES driven translational activity. Viruses 7:1613–1626. doi:10.3390/v7041613
Rodríguez JM, Salas ML (2013) African swine fever virus transcription. Virus Res 173:15–28. doi:10.1016/j.virusres.2012.09.014
Sánchez-Vizcaíno JM, Mur L, Gomez-Villamandos JC, Carrasco L (2015) An update on the epidemiology and pathology of African swine fever. J Comp Pathol 152-9-21. doi:10.1016/j.jcpa.2014.09.003
Saotome K, Morita H, Umeda M (1989) Cytotoxicity test with simplified crystal violet staining method using microtitre plates and its application to injection drugs. Toxicol Vitro 3:317–321. doi:10.1016/0887-2333(89)90039-8
Shibata C, Ohno M, Otsuka M, Kishikawa T, Goto K, Muroyama R, Kato N, Yoshikawa T, Takata A, Koike K (2014) The flavonoid apigenin inhibits hepatitis C virus replication by decreasing mature microRNA122 levels. Virology 462–463:42–48. doi:10.1016/j.virol.2014.05.024
Son M, Lee M, Sung GH, Lee T, Shin YS, Cho H, Lieberman PM, Kang H (2013) Bioactive activities of natural products against herpesvirus infection. J Microbiol 51:545–551. doi:10.1007/s12275-013-3450-9
Terahara N (2015) Flavonoids in foods: a review. Nat Prod Commun 10:521–528
Tong X, Pelling JC (2013) Targeting the PI3K/Akt/mTOR axis by apigenin for cancer prevention. Anticancer Agents Med Chem 13:971–978. doi:10.2174/18715206113139990119
Tulman ER, Delhon GA, Ku BK, Rock DL (2009) African swine fever virus. Curr Top Microbiol Immunol 328:43–87
Vela EM, Bowick GC, Herzog NK, Aronson JF (2008) Genistein treatment of cells inhibits arenavirus infection. Antiviral Res 77:153–156. doi:10.1016/j.antiviral.2007.09.005
Xu L, Su W, Jin J, Chen J, Li X, Zhang X, Sun M, Sun S, Fan P, An D, Zhang H, Zhang X, Kong W, Ma T, Jiang C (2014) Identification of luteolin as enterovirus 71 and coxsackievirus A16 inhibitors through reporter viruses and cell viability-based screening. Viruses 6:2778–2795. doi:10.3390/v6072778
Yang ZF, Bai LP, Huang WB, Li XZ, Zhao SS, Zhong NS, Jiang ZH (2014) Comparison of in vitro antiviral activity of tea polyphenols against influenza A and B viruses and structure-activity relationship analysis. Fitoterapia 93:47–53. doi:10.1016/j.fitote.2013.12.011
Zakaryan H, Revilla Y (2016) African swine fever virus: current state and future perspectives in vaccine and antiviral research. Vet Microbiol 185:15–19. doi:10.1016/j.vetmic.2016.01.016
Zandi K, Teoh BT, Sam SS, Wong PF, Mustafa MR, Abubakar S (2011) Antiviral activity of four types of bioflavonoid against dengue virus type-2. Virol J 8:560. doi:10.1186/1743-422X-8-560
Zhang L, Cheng X, Gao Y, Zheng J, Xu Q, Sun Y, Guan H, Yu H, Sun Z (2015) Apigenin induces autophagic cell death in human papillary thyroid carcinoma BCPAP cells. Food Funct 6:3464–3472. doi:10.1039/c5fo00671f
Zhang W, Qiao H, Lv Y, Wang J, Chen X, Hou Y, Tan R, Li E (2014) Apigenin inhibits enterovirus-71 infection by disrupting viral RNA association with trans-acting factors. PLoS One 9:e110429. doi:10.1371/journal.pone.0110429
Acknowledgements
We thank ARMACAD for investments in laboratory renovation. We thank Dr. Haig Eskandarian (EPFL, Switzerland) for critical reading and useful comments.
Author information
Authors and Affiliations
Corresponding author
Ethics declarations
Ethical standards
This work was supported by a Grant from the Armenian National Science and Education Fund (Biotech-3751). All authors declare that they have no conflict of interest. This article does not contain any studies with human participants or animals performed by any of the authors.
Rights and permissions
About this article
Cite this article
Hakobyan, A., Arabyan, E., Avetisyan, A. et al. Apigenin inhibits African swine fever virus infection in vitro . Arch Virol 161, 3445–3453 (2016). https://doi.org/10.1007/s00705-016-3061-y
Received:
Accepted:
Published:
Issue Date:
DOI: https://doi.org/10.1007/s00705-016-3061-y