Abstract
Stereocomplex crystallite (SC) between enantiomeric poly(l-lactic acid) (PLLA) and poly(d-lactic acid) (PDLA), with largely improved thermal resistance and mechanical properties compared with PLLA and PDLA, is a good nucleating agent for poly(lactic acid) (PLA). The effects of SC and/or polyethylene glycol (PEG) on the crystallization behaviors of PLA were investigated. The non-isothermal and isothermal crystallization kinetics revealed that SC and PEG can separately promote the crystallization rate of PLA by heterogeneous nucleation and increasing crystal growth rate, respectively. However, their promoting effect is limited when used alone, and the modified PLA cannot crystallize completely under a cooling rate of 20 °C/min. When SC and PEG are both present, the crystallization rate of PLA is greatly accelerated, and even under a cooling rate of 40 °C/min, PLA can crystallize completely and get a high crystallinity owing to the excellent balance between simultaneously improved nucleation and crystal growth rate.
Similar content being viewed by others
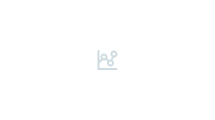
Explore related subjects
Discover the latest articles, news and stories from top researchers in related subjects.Avoid common mistakes on your manuscript.
Introduction
Poly(lactic acid) (PLA), as one of the most promising biodegradable polymers, has exhibited vast appeal in the last decades due to its excellent performance in biocompatibility, biodegradability, renewability, and mechanical properties, which leads to great potential in biomedical, agricultural, and packaging applications [1–5]. Unfortunately, despite being a semicrystalline polymer, PLA is usually amorphous after being processed, owing to its extremely slow crystallization rate and the fast cooling rate in conventional processing methods [6]. Additionally, its glass transition temperature is relatively low (in the range of 50 ~ 60 °C) [7], leading to poor thermal resistance. Increasing the crystallinity of PLA, which can be achieved by enhancing its crystallization, has been regarded as an effective means to improve the thermal resistance and has attracted considerable interest in recent years [6, 8–12]. At the same time, other properties such as barrier property and mechanical properties can also be improved by increasing the crystallinity of PLA.
To enhance the crystallization of PLA, nucleating agents are usually employed [9–11, 13], among which stereocomplex crystallites (SC) of enantiomeric poly(l-lactic acid) (PLLA) and poly(d-lactic acid) (PDLA) have been evidenced to be an efficient and biodegradable nucleating agent for PLA [8, 14–19]. SC, whose melting point, T m, is ca. 230 °C, which is 50 °C higher than that of the homocrystallites of PLLA or PDLA, was first reported by Ikada et al. in 1987 [20]. Since then, attempts have been made to identify the mechanism and the effect of molecular weight of homopolymer, optical purity, blending ratio, preparation methods, and crystallization conditions on the formation and properties of SC [21–29]. In previous work, taking advantage of the temperature window in which only the SC can grow and the homopolymers cannot crystallize [30], we discovered a low-temperature approach to prepare the SC of high molecular weight PLLA and PDLA [31].
Regarding the nucleating ability of SC, Yamane and Sasai demonstrated that the SC can act as heterogeneous nucleation sites of PLLA and enhance the crystallization of PLLA significantly in PLLA/PDLA asymmetric blends with 1–5 wt% of PDLA [14]. Tsuji et al. systematically investigated the effects of SC formed by the introduction of PDLA on the isothermal and non-isothermal crystallization behaviors of PLLA from the melt in a wide range of PDLA content, 0.1 to 10 wt%, and revealed that the addition of a small amount of PDLA and the formed SC was effective to accelerate the overall PLLA crystallization when the PDLA content and the crystallization conditions were scrupulously selected [15]. Rahman et al. reported that the crystallization peak temperature of a PLLA/PDLA blend with 3 % PDLA was 30 °C higher than that of pure PLLA at a cooling rate of 2.5 °C/min from 200 °C [16]. Sun et al. found that SC could stay at diverse states when the PLLA/PDLA asymmetric blends were cooled from different temperatures. Accordingly, the SC, as an effective nucleation agent, show distinct effects on the crystallization of PLLA [19]. It should also be noted that SC are also biodegradable [26], which does not do harm to the applications of PLA in biomedical and environmental fields compared with other nucleating agents for PLA.
However, in our exploration, we found that SC could promote the crystallization of PLA efficiently under a low cooling rate, but PLA, with the presence of SC, cannot crystallize completely under a high cooling rate, e.g., 20 °C/min. So the PLA samples modified by SC would still be amorphous due to a much higher cooling rate existing in conventional processing, revealing that more efforts are still needed for using SC as a nucleation agent to get high crystallinity of PLA products.
Similar to that of other semicrystalline polymers, the crystallization behavior of PLA consists of nucleation and crystal growth. With the incorporation of SC, the nucleation process of PLA can be enhanced by its heterogeneous nucleation effect. However, the crystal growth rate does not change and is still very slow owing to the rigidity of PLA chains [16]. So, taking advantage of the nucleation ability of SC, we may greatly accelerate the overall crystallization process of PLA by simultaneously improving the mobility of PLA chains. To enhance the mobility of chains, plasticizers are frequently used [6, 32–38]. Apart from decreasing the glass transition temperature (T g) of PLA, plasticizers have been proved to increase the crystal growth rate of PLA and thus accelerate its crystallization rate [6, 39–41]. Herein, we came up with the idea of introducing SC and plasticizer simultaneously to achieve a high overall crystallization rate of PLA by improving and balancing the nucleation and crystal growth rate. In this present work, polyethylene glycol (PEG), which is an efficient plasticizer for PLA and also biocompatible, was introduced into a PLLA/PDLA asymmetric blend to improve the mobility of PLA chain segments. The cooperative effects of SC and PEG on the non-isothermal and isothermal crystallization behaviors of PLA were investigated in order to achieve an efficient way to largely accelerate the overall crystallization rate of PLA.
Experimental section
Materials
A commercial PLA (trade name 4032D) was used as a PLLA source. It is a semicrystalline grade supplied by NatureWorks LLC (USA) that comprises about 2 % d-units; its weight-averaged molecular weight (M w) is 2.1 × 105 g/mol and its polydispersity (PDI) is 1.7. The PDLA, which was synthesized by the ring-opening polymerization of d-lactic acid, was kindly supplied by Professor Chen Xue-Si's research group at the State Key Laboratory of Polymer Physics and Chemistry, China. The M w of PDLA is about 1.0 × 105 g/mol and its PDI is 1.7. PEG, with a molecular weight of 800 g/mol, was purchased from Kelong Chemical Reagent Co. (Chengdu, China).
Sample preparation
Solution blending was utilized to prepare the PLA blend. The detailed preparation procedure was as follows: First, solutions of PLA, PEG, and PDLA with dichloromethane as the solvent were separately prepared. The concentrations of these solutions are 1.0, 1.0, and 0.1 g/dL, respectively. Then the PLA solution was mixed with different contents of PEG and/or PDLA solution to obtain the corresponding samples as shown in Table 1 and stirred for 2 h. The mixed solutions were cast onto flat dishes, and the solvent was evaporated in a hood at room temperature for 48 h. The obtained films were further vacuum-dried at 50 °C for 48 h to remove the residual solvent and used for differential scanning calorimetry (DSC) measurement and optical microscopy observation. For the sake of concision, samples were labeled according to their composition, also shown in Table 1.
Characterizations
The melting behaviors of the samples were investigated using a DSC Q20 (TA Instruments, USA) at a heating rate of 10 °C/min from 20 to 250 °C. The non-isothermal and isothermal crystallization behaviors were also monitored by DSC. The samples of around 5 mg were heated to 180 °C at a rate of 100 °C/min and held at 180 °C for 5 min to completely melt the homocrystallites (first heating) and then cooled to 0 °C at various rates of 2.5, 10, 20, and 40 °C/min and reheated from 0 to 180 °C (second heating) at a constant rate of 10 °C/min to record the non-isothermal crystallization behavior and the corresponding melting behavior. For the isothermal crystallization, the samples were heated to 180 °C at a rate of 100 °C/min, held at 180 °C for 5 min, and then cooled to 140 °C at a rate of 100 °C/min to monitor the isothermal crystallization process for 40 min. Then this heating and cooling cycle was repeated using different crystallization temperatures at 5 °C intervals from 125 to 140 °C. All these processes were carried out under nitrogen atmosphere.
Wide-angle X-ray diffraction (WAXD) measurements of the samples which had been cooled from 180 °C at various cooling rates were carried out with a DX-1000 X-ray diffractometer (Dandong Fanyuan Instrument Co. LTD) using a Cu Kα radiation source (λ = 0.154056 nm, 40 kV, 25 mA) in the scanning angle range of 2θ = 5°–50° at a scan speed of 3°/min.
The morphologies of PLA and its blends after isothermal crystallization at 140 °C were characterized using an Olympus BX51 polarizing optical microscope (Olympus Co., Tokyo, Japan) equipped with a hot-stage (LINKAM THMS 600). The experimental temperature procedure was the same as isothermal crystallization in the DSC measurement.
Results and discussion
Non-isothermal crystallization
Figure 1 shows the DSC traces obtained during the first heating process. No cold crystallization peak appeared for all the samples, indicating that all these samples were completely crystallized during the process of sample preparation [17, 39]. Here, a tiny melting peak at 215 °C, corresponding to the melting of SC, could be observed for LD and LDG. It revealed that by the incorporation of PDLA, even at a concentration as low as 1 wt%, SC were successfully introduced to the blends of LD and LDG.
To explore the promoting effect of PEG and/or the formed SC on the crystallization of PLA, the non-isothermal crystallization of samples were investigated by DSC, and the cooling curves with the cooling rate of 2.5 °C/min are displayed in Fig. 2. Before cooling, all these samples were isothermal for 5 min under 180 °C (above the melting point of homocrystallite of PLA but below the melting point of SC) at which only homocrystallites were melted and SC were reserved in the melts of LD and LDG. As shown in Fig. 2, no trace of separate crystallization of PEG was found for samples LG and LDG, confirming that the phase separation of PEG did not occur at such content of PEG [6, 39]. A very weak and broad crystallization peak appeared at 96.7 °C for neat PLA. With the addition of PEG, the crystallization peak of LG appeared at 108.3 °C, showing an 11.6 °C increase of the crystallization peak temperature (T p) compared to that of neat PLA. Furthermore, the peak became much stronger. These results clearly show that PEG can promote the crystallization rate of PLA during the cooling process from the melt. With the incorporation of SC, a strong and sharp crystallization peak was observed at 137.4 °C for sample LD, which almost shifted to a higher temperature by 40 °C compared with that of neat PLA. It evidently manifested that the introduction of SC facilitated the crystallization rate of PLA remarkably by acting as heterogeneous nucleation seeds. Dramatically, for LDG, with the coexistence of SC and PEG, the sharpest crystallization peak and the highest peak temperature (138.8 °C) were observed among all the four samples, showing that there existed a cooperative effect of PEG and SC on the crystallization of PLA so that the crystallization rate of PLA could be further promoted when both PEG and SC were presented. What should be mentioned is that PEG cannot act as heterogeneous nucleation seeds for PLA, but the samples with the presence of PEG showed a higher peak temperature for LG and LDG compared to L and LD, respectively. Li and Huneault thought that this phenomenon was the result of a significantly sharpened crystallization peak as the crystallization rate of PLA was accelerated by the addition of PEG and this sharpened peak pushed up the crystallization peak temperature (T p) closer to the onset crystallization temperature [6]. As shown in Fig. 2, samples LD and LDG almost showed the same onset crystallization temperature and sample LDG showed a sharper crystallization peak compared to sample LD, which are exactly fit for their characterization.
The second heating curves were used to express the melting behavior of the crystallites formed in the previous cooling and heating cycles. Particularly, as to PLA, the presence or absence of a cold crystallization peak during the second heating provides further information about the complete or incomplete crystallization during the previous cooling process [17, 18, 42]. Figure 3 shows the DSC heating curves of L, LG, LD, and LDG samples that were previously cooled from 180 °C at various cooling rates, and the heating scan rate was 10 °C/min. Here, a cold crystallization peak was observed for the neat PLA that experienced a previous cooling rate of 2.5 °C/min, revealing that the crystallization rate of PLA was indeed so slow that it could not crystallize completely at such a low cooling rate (2.5 °C/min). For LG and LD that experienced a previous cooling rate of 2.5 °C/min, they did not exhibit a cold crystallization peak while their cold crystallization peak appeared for the sample that experienced a cooling rate of 10 and 20 °C/min, respectively. These results demonstrate that the incorporation of PEG or SC can indeed accelerate the crystallization rate of PLA, while their promoting effects are limited and PLA cannot completely crystallize under a higher cooling rate. Fascinatingly, when both PEG and SC were presented, the cold crystallization peak was removed for samples that experienced all the previous cooling rates. All these results obviously reveal that there exists a cooperative effect of PEG and SC on the crystallization of PLA. As a result of this cooperative effect, the crystallization rate of PLA was promoted substantially so that LDG can completely crystallize under a higher cooling rate.
Moreover, from Fig. 3, a clear glass translation was observed around 59 °C for neat PLA and LD while around 37 °C for LG. The decrease of T g from 59 to 37 °C was the result of the plasticizing effect of PEG which enhanced the segmental mobility of PLA. Interestingly, the glass translation disappeared for all the samples of LDG, which may be the result of the LDG samples being completely crystallized so that most of the chains were crystallized and the motion of the chains in the amorphous region was significantly confined by the presence of vast crystallites, which greatly depressed the glass translation behavior. The glass transition was also absent for the completely crystallized LG sample that experienced a previous cooling rate of 2.5 °C/min, which also confirmed the conjecture above. Also, as displayed in Fig. 3, there existed abundant information about the melting behaviors, but this is beyond the scope of this work and will be investigated in detail in the following work.
These crystallized samples under various cooling rates were also investigated by means of WAXD, and the results are shown in Fig. 4. The diffraction peaks at 2θ values of 14.8°, 16.7°, 19.1°, and 22.3°, which correspond to the (010), (200) and/or (110), (203), and (015) planes of PLA crystals in α-phase [43], respectively, were observed for all the samples when they experienced a previous cooling rate of 2.5 °C/min. However, these diffraction peaks disappeared for sample L when it experienced a previous cooling rate of 10 °C/min and for samples LG and LD when they experienced a previous cooling rate of 20 and 40 °C/min, respectively, suggesting that these samples were almost in amorphous state. For sample LDG, these strong diffraction peaks of PLA crystals still existed after experiencing a previous cooling rate of 40 °C/min. This trend is in accordance with that of DSC results which have been shown in Fig. 3. Except for the diffraction peaks of PLA crystals, the WAXD profiles do not show the visible diffraction peaks of SC for both LD and LDG that should be at 2θ values of 11.6°, 20.6°, and 23.5° [44], which is owing to their tiny content of SC. In addition, by the WAXD method, the crystallinity of these samples after they experienced various cooling rates are obtained and presented in Fig. 5. Compared with pure PLA, a high increase of crystallinity for samples LG and LD that experienced a cooling rate of lower than 20 °C/min was observed, but under a higher previous cooling rate of 40 °C/min, LG and LD samples were almost in amorphous state, similar to pure PLA. These results also reveal the limited promoting effect of PEG or SC on the crystallization of PLA. Interestingly, the crystallinity of sample LDG still remained at a high level (>35 %) when it experienced a previous cooling rate of 40 °C/min. These results clearly demonstrate that a high degree of crystallinity of PLA samples can be successfully obtained under a higher cooling rate during the non-isothermal crystallization process because of the cooperative effect of SC and PEG on enhancing the crystallization of PLA.
Isothermal crystallization
To further reveal the cooperating effect of PEG and SC on the crystallization of PLA, the isothermal crystallization behaviors were investigated under various crystallization temperatures, and the development of heat flow and relative degree of crystallinity X c of compositions as a function of time are displayed in Figs. 6 and 7, respectively. X c is a relative parameter defined as follows [45]:
where dH/dt is the rate of heat evolution, ΔH t is the heat generated at time t, and ΔH ∞ is the total heat. As shown in Fig. 5, no heat flow peak for samples L and LG was present in the investigated time scale when isothermally crystallized at 140 °C, indicating that no crystallization occurred at such a high temperature. However, obvious heat flow peaks could be seen for samples LD and LDG with the presence of SC, which attested the heterogeneous nucleation effect of SC on the crystallization of PLA. At T c = 135 °C, the crystallization of samples L and LG occurred and the heat flow peak began to appear for sample LG, while the peak began to appear at T c = 130 °C for sample L. These results reveal that PEG promotes the crystallization rate of PLA by increasing the chain mobility again. In addition, among all these compositions, the time at the peak position of the exothermic heat flow curve (standing for the time when the maximum crystallization rate appears) [10] was the shortest for sample LDG while the longest for sample L, indicating that the overall crystallization rate of PLA was accelerated by the presence of PEG or SC and further cooperatively promoted when both PEG and SC were present. Likewise, the growth of X c shown in Fig. 7 and the half crystallization time displayed in Table 2 revealed the same conclusions.
As shown in Table 2, at T c = 125 °C, the half crystallization time (t 0.5), obtained from the curve of the development of X c to t, was as long as 909 s for neat PLA. Thanks to the promoting effect of PEG and SC, the t 0.5 of LG and LD were both shortened compared with that of neat PLA. Particularly, the t 0.5 of LDG at T c = 125 °C was sharply decreased to 39 s, 1/23.5 of the neat one, revealing that the overall crystallization rate of PLA can be greatly improved owing to the cooperative effect of PEG and SC on the crystallization of PLA.
Discussion on the mechanism of the cooperative effect
Based on the above results, SC or PEG can accelerate the crystallization rate of PLA by improving the peak temperature of crystallization and shortening the half crystallization time, respectively, but their promoting effects are limited and PLA cannot crystallize completely under a higher cooling rate. However, when SC and PEG are both present, the crystallization rate of PLA is further and greatly promoted as its crystallization peak temperature is highest (as shown in Fig. 2) and its half crystallization time is shortest (as displayed in Table 2). In addition, even under a high cooling rate, a high degree of crystallinity of PLA samples can be obtained. These results obviously reveal the cooperative effect of SC and PEG on the crystallization (both the crystallization rate and crystallinity) of PLA. Curiously, why does this cooperative effect appear?
As we know, typical crystallization is a two-step process: (1) formation of stable nuclei (the nucleation stage) followed by (2) crystal growth characterized by the crystal growth rate (G). The overall crystallization rate is the result of the combination of nucleation rate and crystal growth rate. About the effect of PEG on the crystallization of PLA, there have been a lot of great works [7, 33, 38–40]. Among them, it has been reported that blending with PEG increases the spherulite growth rate of PLA but decreases the nucleation density [33, 39, 41, 46]. The increase of spherulite growth rate can be ascribed to the plasticizing role of PEG which enhances the segmental mobility of PLA. Thus, with the incorporation of PEG, the crystal growth rate of PLA was enhanced, while the nucleation process of PLA was not improved because PEG cannot act as heterogeneous nucleation seeds for PLA. Besides, the fact that the increase of the segmental mobility goes against the formation and stability of the homogeneous nuclei results in the decrease of nucleation density with the addition of PEG. As a result, with the presence of PEG, LG showed a larger crystal growth rate but a smaller nucleation density than neat PLA. So the promoting effect of PEG on the crystallization of PLA was limited by the smaller nucleation density. With the incorporation of PDLA, SC were successfully introduced. The presence of SC acted as heterogeneous nucleating seeds for PLA which improved the crystallization temperature substantially as shown in the results of non-isothermal and isothermal crystallization results. Here, with the formed SC, there were many heterogeneous nucleating seeds for PLA, but the crystal growth rate of PLA was not improved yet. Furthermore, it has been reported that the presence of SC can reduce the crystal growth rate of the homocrystallites due to the increase of the viscosity and the block of the PDLA chains which do not discharge into the SC [16]. Consequently, with the presence of SC, sample LD showed a larger nucleation density but a slower crystal growth rate than neat PLA. Hence, the promoting effect of SC on the crystallization of PLA was limited by its slower crystal growth rate. In summary, the addition of PEG and PDLA (SC) alone can promote the crystallization rate of PLA by the improvement of crystal growth rate and nucleation density, respectively, but unfortunately their promoting effects were limited by their poor nucleation density and poor crystal growth rate, respectively.
For sample LDG, with the presence of PEG and SC simultaneously, the nucleation density of PLA was improved by the heterogeneous nucleating seeds supplied by SC, meanwhile the growth rate of PLA was also enhanced by the plasticizing role of PEG. Hence, the incorporation of PEG and SC simultaneously overcame the shortages of adding them alone, so the crystallization rate of PLA was promoted substantially.
To confirm the above conjectures, the morphologies and crystal growth processes of samples L, LG, LD, and LDG were investigated by polarizing optical microscopy (POM). Figure 8 shows the eventual morphologies of the samples isothermally crystallized at 140 °C. It is clear that the addition of PEG increased the size of spherulites and decreased the nucleation density of PLA for sample LG compared with pure PLA, which is in accordance with our statement that the promoting effect of PEG is limited by the low nucleation density, even lower than that of neat PLA. The presence of SC resulted in the formation of a mass of small-sized spherulites for sample LD, obviously revealing the effective heterogeneous nucleation effect of SC on PLA. Interestingly, when PEG and SC were both present, the spherulites were much more than those of sample LG (owing to the larger nucleation density and the heterogeneous nucleation effect of SC) but less than those of sample LD, revealing that the nucleation density of sample LDG is lower than that of sample LD which is caused by the addition of PEG.
To observe the spherulite growth processes of samples LD and LDG, 155 °C, a relatively high temperature, was taken as the isothermal crystallization temperature, due to their high nucleation density and the large amount of small-sized spherulites under lower T c (seen in Fig. 8). At the same time, under such high T c, the nucleation rate of samples L and LG were indeed slow and no crystals emerged for as long as 2 h, at which the degradation of PLA should be serious. Herein, a special temperature procedure was adopted, i.e., samples L and LG were first crystallized at 130 °C for 3 min before being observed at 155 °C. After samples L and LG were crystallized at 130 °C for 3 min, some small crystals emerged. Then the growth processes of these crystals could be investigated at 155 °C, and all the results of these four samples are displayed in Fig. 9a.
As shown in Fig. 9a, all the crystal growth rates of these four samples stay at a relatively low level due to the high T c. It is clear that the presence of SC reduced the crystal growth rate of PLA for sample LD compared to sample L, which is in accordance with a previous report [16] and reveals that the promoting effect of SC is limited by the low spherulite growth rate. As expected, with the addition of PEG, sample LG shows a faster crystal growth rate than neat PLA. Moreover, this promoting effect is greater at a low crystallization temperature (140 °C) at which the crystal growth rate of LG is almost three times than that of L as shown in Fig. 9b. Simultaneously, the presence of PEG also enhances the crystal growth of sample LDG greatly compared to sample LD.
In summary, the nucleation density and crystal growth rate of sample LDG were simultaneously improved by the addition of PDLA (SC) and PEG, respectively, compared to L. Meanwhile, even through with a lower crystal growth rate than LG and a smaller nucleation density than LD, sample LDG showed a much faster overall crystallization rate both under non-isothermal condition (seen in Figs. 2, 3, 4, and 5) and isothermal crystallization (seen in Figs. 6 and 7 and Table 2) than samples LD and LG. Thus, it can be concluded that the cooperative effect of PEG and SC on the crystallization (both the crystallization rate and crystallinity) of PLA is really the result of the excellent balance between the enhanced nucleation and the increased crystal growth rate.
Conclusions
Effects of the addition of PDLA and/or PEG on the crystallization behaviors of PLA were investigated. By the incorporation of PDLA, even at a concentration as low as 1 wt%, the SC were successfully introduced to PLA/PDLA blends and PLA/PDLA/PEG blends. The non-isothermal and isothermal crystallization kinetics showed that the introduced SC or PEG could accelerate the crystallization rate of PLA by improving the crystallization peak temperature and shortening the half crystallization time, but their promoting effects were limited and PLA could not crystallize completely under a higher cooling rate. Interestingly, when SC and PEG were both present, the crystallization rate of PLA was further promoted as it showed the highest crystallization peak temperature and the shortest half crystallization time; in addition, even under a high cooling rate (40 °C/min), a completely crystallized PLA could be obtained. These results obviously revealed the cooperative effect of SC and PEG on the crystallization of PLA. From the POM results, when PEG and SC were both present, the high nucleation density and the fast crystal growth rate were confirmed, which revealed that the cooperative effect of SC and PEG on the crystallization of PLA was the result of the excellent balance between nucleation and crystal growth rate which were both improved by the addition of PDLA (SC) and PEG, respectively. Moreover, due to the cooperative effect of SC and PEG, high crystallinity of PLA samples can be successfully obtained even under a high cooling rate.
References
Lim L-T, Auras R, Rubino M (2008) Processing technologies for poly(lactic acid). Prog Polym Sci 33(8):820–852
Gilding D, Reed A (1979) Biodegradable polymers for use in surgery—polyglycolic/poly(actic acid) homo- and copolymers: 1. Polymer 20(12):1459–1464
Athanasiou KA, Niederauer GG, Agrawal C (1996) Sterilization, toxicity, biocompatibility and clinical applications of polylactic acid/polyglycolic acid copolymers. Biomaterials 17(2):93–102
Trimaille T, Pichot C, Elaissari A, Fessi H, Briancon S, Delair T (2003) Poly(d, l-lactic acid) nanoparticle preparation and colloidal characterization. Colloid Polym Sci 281(12):1184–1190
Wang C-F, Xie H-Y, Cheng Y-P, Chen L, Hu MZ, Chen S (2011) Chemical synthesis and optical properties of CdS-poly(lactic acid) nanocomposites and their transparent fluorescent films. Colloid Polym Sci 289(4):395–400
Li HB, Huneault MA (2007) Effect of nucleation and plasticization on the crystallization of poly(lactic acid). Polymer 48(23):6855–6866
Kulinski Z, Piorkowska E (2005) Crystallization, structure and properties of plasticized poly(l-lactide). Polymer 46(23):10290–10300
Saeidlou S, Huneault MA, Li H, Sammut P, Park CB (2012) Evidence of a dual network/spherulitic crystalline morphology in PLA stereocomplexes. Polymer 53(25):5816–5824
Barrau S, Vanmansart C, Moreau M, Addad A, Stoclet G, Lefebvre J-M, Seguela R (2011) Crystallization behavior of carbon nanotube–polylactide nanocomposites. Macromolecules 44(16):6496–6502
Xu Z, Niu Y, Yang L, Xie W, Li H, Gan Z, Wang Z (2010) Morphology, rheology and crystallization behavior of polylactide composites prepared through addition of five-armed star polylactide grafted multiwalled carbon nanotubes. Polymer 51(3):730–737
Xu Z, Niu Y, Wang Z, Li H, Yang L, Qiu J, Wang H (2011) Enhanced nucleation rate of polylactide in composites assisted by surface acid oxidized carbon nanotubes of different aspect ratios. ACS Appl Mater Interfaces 3(9):3744–3753
Na B, Zou S, Lv R, Luo M, Pan H, Yin Q (2011) Unusual cold crystallization behavior in physically aged poly(l-lactide). J Phys Chem B 115(37):10844–10848
Zhong Y, Zhang Y, Yang J, Li W, Wang Z, Xu D, Chen S, Ding Y (2013) Exponentially increased nucleation ability for poly(l-lactide) by adding acid-oxidized multiwalled carbon nanotubes with reduced aspect ratios. Sci China-Chem 56(2):181–194
Yamane H, Sasai K (2003) Effect of the addition of poly(d-lactic acid) on the thermal property of poly(l-lactic acid). Polymer 44(8):2569–2575
Tsuji H, Takai H, Saha SK (2006) Isothermal and non-isothermal crystallization behavior of poly(l-lactic acid): effects of stereocomplex as nucleating agent. Polymer 47(11):3826–3837
Rahman N, Kawai T, Matsuba G, Nishida K, Kanaya T, Watanabe H, Okamoto H, Kato M, Usuki A, Matsuda M, Nakajima K, Honma N (2009) Effect of polylactide stereocomplex on the crystallization behavior of poly(l-lactic acid). Macromolecules 42(13):4739–4745
Narita J, Katagiri M, Tsuji H (2011) Highly enhanced nucleating effect of melt-recrystallized stereocomplex crystallites on poly(l-lactic acid) crystallization. Macromol Mater Eng 296(10):887–893
Narita J, Katagiri M, Tsuji H (2013) Highly enhanced accelerating effect of melt-recrystallized stereocomplex crystallites on poly(l-lactic acid) crystallization, 2—effects of poly(d-lactic acid) concentration. Macromol Mater Eng 298(3):270–282
Sun J, Yu H, Zhuang X, Chen X, Jing X (2011) Crystallization behavior of asymmetric PLLA/PDLA blends. J Phys Chem B 115(12):2864–2869
Ikada Y, Jamshidi K, Tsuji H, Hyon SH (1987) Stereocomplex formation between enantiomeric poly(lactides). Macromolecules 20(4):904–906
Tsuji H, Horii F, Hyon SH, Ikada Y (1991) Stereocomplex formation between enantiomeric poly(lactic acid)s. 2. Stereocomplex formation in concentrated solutions. Macromolecules 24(10):2719–2724
Tsuji H, Hyon SH, Ikada Y (1991) Stereocomplex formation between enantiomeric poly(lactic acid)s. 3. Calorimetric studies on blend films cast from dilute solution. Macromolecules 24(20):5651–5656
Tsuji H, Hyon SH, Ikada Y (1992) Stereocomplex formation between enantiomeric poly(lactic acids). 5. Calorimetric and morphological studies on the stereocomplex formed in acetonitrile solution. Macromolecules 25(11):2940–2946
Tsuji H, Ikada Y (1999) Stereocomplex formation between enantiomeric poly(lactic acid)s. XI. Mechanical properties and morphology of solution-cast films. Polymer 40(24):6699–6708
Tsuji H, Tezuka Y (2004) Stereocomplex formation between enantiomeric poly(lactic acid)s. 12. Spherulite growth of low-molecular-weight poly(lactic acid)s from the melt. Biomacromolecules 5(4):1181–1186
Tsuji H (2005) Poly(lactide) stereocomplexes: formation, structure, properties, degradation, and applications. Macromol Biosci 5(7):569–597
He Y, Xu Y, Wei J, Fan Z, Li S (2008) Unique crystallization behavior of poly(l-lactide)/poly(d-lactide) stereocomplex depending on initial melt states. Polymer 49(26):5670–5675
Purnama P, Kim SH (2009) Stereocomplex formation of high-molecular-weight polylactide using supercritical fluid. Macromolecules 43(2):1137–1142
Sun J, Shao J, Huang S, Zhang B, Li G, Wang X, Chen X (2012) Thermostimulated crystallization of polylactide stereocomplex. Mater Lett 89:169–171
Bao R-Y, Yang W, Jiang W-R, Liu Z-Y, Xie B-H, Yang M-B (2013) Polymorphism of racemic poly(l-lactide)/poly(d-lactide) blend: effect of melt and cold crystallization. J Phys Chem B 117(13):3667–3674
Bao R-Y, Yang W, Jiang W-R, Liu Z-Y, Xie B-H, Yang M-B, Fu Q (2012) Stereocomplex formation of high-molecular-weight polylactide: a low temperature approach. Polymer 53(24):5449–5454
Zou S, Na B, Lv R, Pan H (2012) The plasticizer-assisted formation of a percolating multiwalled carbon nanotube network in biodegradable poly(l-lactide). J Appl Polym Sci 123(3):1843–1847
Yang JM, Chen HL, You JW, Hwang JC (1997) Miscibility and crystallization of poly(l-lactide) poly(ethylene glycol) and poly(l-lactide)/poly(epsilon-caprolactone) blends. Polym J 29(8):657–662
Martin O, Averous L (2001) Poly(lactic acid): plasticization and properties of biodegradable multiphase systems. Polymer 42(14):6209–6219
Li Y, Wu H, Wang Y, Liu L, Han L, Wu J, Xiang F (2010) Synergistic effects of PEG and MWCNTs on crystallization behavior of PLLA. J Polym Sci B Polym Phys 48(5):520–528
Zhang Y, Zhang QH, Zha LS, Yang WL, Wang CC, Jiang XG, Fu SK (2004) Preparation, characterization and application of pyrene-loaded methoxy poly(ethylene glycol)–poly(lactic acid) copolymer nanoparticles. Colloid Polym Sci 282(12):1323–1328
Nakafuku C, Takehisa SY (2004) Glass transition and mechanical properties of PLLA and PDLLA–PGA copolymer blends. J Appl Polym Sci 93(5):2164–2173
Nakafuku C (1996) Effects of molecular weight on the melting and crystallization of poly(l-lactic acid) in a mixture with poly(ethylene oxide). Polym J 28(7):568–575
Hu Y, Hu Y, Topolkaraev V, Hiltner A, Baer E (2003) Crystallization and phase separation in blends of high stereoregular poly(lactide) with poly(ethylene glycol). Polymer 44(19):5681–5689
Jia ZY, Zhang KY, Tan JJ, Han CY, Dong LS, Yang YM (2009) Crystallization behavior and mechanical properties of crosslinked plasticized poly(l-lactic acid). J Appl Polym Sci 111(3):1530–1539
Zhang Y, Xu H, Yang J, Chen S, Ding Y, Wang Z (2013) Significantly accelerated spherulitic growth rates for semicrystalline polymers through the layer-by-layer film method. J Phys Chem C 117(11):5882–5893
Yasuniwa M, Tsubakihara S, Sugimoto Y, Nakafuku C (2004) Thermal analysis of the double-melting behavior of poly(l-lactic acid). J Polym Sci B Polym Phys 42(1):25–32
Hoogsteen W, Postema A, Pennings A, Ten Brinke G, Zugenmaier P (1990) Crystal structure, conformation and morphology of solution-spun poly(l-lactide) fibers. Macromolecules 23(2):634–642
Cartier L, Okihara T, Lotz B (1997) Triangular polymer single crystals: stereocomplexes, twins, and frustrated structures. Macromolecules 30(20):6313–6322
Cebe P, Hong S-D (1986) Crystallization behaviour of poly(ether-ether-ketone). Polymer 27(8):1183–1192
Zhang Y, Wang Z, Jiang F, Bai J, Wang Z (2013) Effect of miscibility on spherulitic growth rate for double-layer polymer films. Soft Matter 9(24):5771–5778
Acknowledgments
The authors gratefully acknowledge the financial support of the Special Funds for Major Basic Research (nos. 2011CB606006 and 2012CB025902), the National Natural Science Foundation of China (nos. 51033003 and 51073109), and the Fundamental Research Funds for the Central Universities (no. 2011SCU04A03).
Author information
Authors and Affiliations
Corresponding author
Rights and permissions
About this article
Cite this article
Wei, XF., Bao, RY., Cao, ZQ. et al. Greatly accelerated crystallization of poly(lactic acid): cooperative effect of stereocomplex crystallites and polyethylene glycol. Colloid Polym Sci 292, 163–172 (2014). https://doi.org/10.1007/s00396-013-3067-x
Received:
Revised:
Accepted:
Published:
Issue Date:
DOI: https://doi.org/10.1007/s00396-013-3067-x