Abstract
In this study, zeolite Y was synthesized from sodium silicate, aluminum hydroxide, sodium hydroxide and distilled water under hydrothermal method. The Box–Behnken design was used as a response surface method considering seven factors affecting the crystallization of zeolite to determine the number of experiments. The linear, square and interaction effects of the factors were investigated. The factors consist of four factors for the gel composition, including the molar value of Si, Al, Na and H2O and three factors for the synthesis conditions, including aging time, crystallization time and temperature. The XRD patterns of the synthesized samples were compared with the XRD pattern of standard zeolite Y. Among the samples, sample 50 with the highest intensity and maximum total area of 14 peaks, was selected as the reference sample and it was used to determine the relative crystallinity percentage of the remaining samples. Based on the results of the experiments, it was concluded that changes in the gel composition have a more significant effect on the response in comparison with changes in the synthesis conditions. In addition, optimization of the obtained model was carried out and a zeolite with higher relative crystallinity than the standard zeolite Y was synthesized. At the optimal point, zeolite Y was synthesized with a relative crystallinity of 117.5%. The composition of the gel was 0.59 SiO2: 0.0563 Al2O3: 0.4266 Na2O: 12.376 H2O. The total synthesis time was 30 h.
Similar content being viewed by others
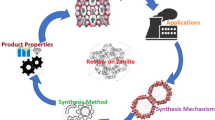
Avoid common mistakes on your manuscript.
Introduction
Zeolites are minerals composed of silicon, aluminum and oxygen elements. These elements are in tetrahedral forms and AlO4 and SiO4 exist in the zeolite structure. Zeolites are also called crystalline aluminosilicates that have a three-dimensional framework structure (Grand et al. 2016; Ma and Lothenbach 2020; Tang et al. 2017).
Zeolites have many applications in various industries due to their microporous structural properties and ion exchangeability. Synthetic zeolites have the highest market value. Faujasite-type synthetic zeolites are economical and have more than 95% of the catalyst market (Cejka et al. 2007). Zeolites Y and X with a faujasite crystal structure are synthetic zeolites that have attracted lots of attention as industrial catalysts in recent decades (Cejka et al. 2007; DA 2013; Liu et al. 2016). The obvious difference between zeolite X and zeolite Y is in the ratio of Si/Al and their acid strength. The ratio of Si/Al for zeolite X is between 1 and 1.5 and for zeolite Y this ratio is higher than 1.5. The higher ratio of Si/Al in zeolite Y improves its thermal stability compared to zeolite X. Also, zeolite X has a higher acid strength than zeolite Y due to the quantity of more acid sites (Julbe and Drobek 2016a, 2016b; Hiroshi et al. 1969). There are several applications for zeolite Y. It can be used for the removal of heavy metals (Chen et al. 2020a; Sulaiman et al. 2020; Sellaoui et al. 2021), and also desulfurization of petroleum products (Tian et al. 2014; Dias da Silva et al. 2019; Cerutti et al. 2019). The most important application of zeolite Y is the base for catalyst in cracking processes. Fluid catalytic cracking (FCC) is the main process in oil refineries for gasoline production from heavy residuals of the distillation columns. The main component of commercial FCC catalysts is zeolite Y or ultrastable zeolite Y (USY) (Louwen et al. 2020; Xie et al. 2019).
Many different variables can affect the crystallization of zeolites, such as the composition of precursor, sources used for reactants, crystallization time and temperature, aging time, alkalinity, organic templates, Si/Al ratio, seeding, and stirring (Cejka et al. 2007; Kacirek and Lechert 1975). Therefore, Achieving a model to study and control the crystal growth kinetics of zeolites based on effective factors, as well as finding an appropriate industrial synthesis method has been considered (Chen et al. 2020b).
The starting point of the application of statistical design in various industries dates back to the 1930s, but in the following decades, the response surface methodology (RSM) was developed and became even more practical by Box and Wilson. This method has been widely used for research and development in various process industries, including the chemical industries (Montgomery 2017). The RSM, which has all the statistical and mathematical techniques, is used to identify the process and provide a significant model for the required response. The central composite design (CCD) and the Box–Behnken design (BBD) as the standard response surface designs are used frequently. Achieving the optimal process conditions and providing the operating requirements by specifying the appropriate range of factors is the ultimate aim of the RSM method (Montgomery 2017).
The ternary reaction composition diagram of the gel composition of zeolites has been reported for zeolites Y, X and A by several researchers such as Breck (1974) and Kostinko (1983). Lutz showed an example of these diagrams with the specified area for these three zeolites. By comparing these areas, it can be seen that the area of gel composition for zeolites Y and X is significantly smaller than this area for zeolite A (Lutz 2014). A similar diagram is reported by Garcia for eight samples synthesized with different gel compositions considering the determined Breck zone, which shows the synthesis of zeolites Y and P together (Garcia et al. 2016). Reiprich et al. presented a more complete ternary diagram by collecting reported data for the synthesis of FAU zeolites and comparing them with the IZA-verified syntheses for zeolite X and Y (Reiprich et al. 2020).
Oyinade et al. have studied the influential factors in the synthesis and characterization of zeolite Y. Their experimental design was performed using BBD and the following linear model was obtained for measuring crystallinity in terms of effective factors, including aging time, crystallization time and temperature (Oyinade et al. 2016).
Bortolatto et al. have investigated the effects of temperature and crystallization time over the crystallinity of zeolite Y using experimental design. 32 factorial design was used and the results confirmed that time is a key factor in the crystallization of zeolite Y (Bortolatto et al. 2017).
In the current study, the main goal was to achieve a proper gel composition and determine process conditions for the synthesis of zeolite Y from industrial raw materials. Taking advantage of the simple synthesis process without using seed was one of the goals of this research. In addition, compared to the previous studies of researchers, an effort was made to study the effect of more factors that are involved in the production process, to have a more convenient and accurate control over the synthesis process. The effect of seven factors affecting the crystallization process was also investigated. Four factors, including the moles of constituent elements such as silicon, aluminum, sodium and molar value of water were considered to investigate their effect on gel composition. In addition, three factors including, aging time, crystallization time and temperature were considered to survey the effect of synthesis conditions on the crystallinity of zeolite Y. The effects were studied analytically using the Box-Behnken design. Consequently, a model was presented based on the significant factors of zeolite Y crystallinity. Optimization of the model was done to increase the crystallinity percentage and reduce the synthesis process time (sum of crystallization and aging times). Characterization of the synthesized samples was performed by XRD analysis. The optimum sample was characterized using XRD, XRF, FTIR and FESEM analyses.
Experimental
Materials
Sodium silicate (water glass) was used as the source of silicate, and sodium aluminate was used as the source of aluminate. Sodium hydroxide and aluminum hydroxide were used to make sodium aluminate. All chemicals used in all syntheses were industrial grade and had a specific and fixed composition. Table 1 shows the specifications of the chemicals used.
Experimental design
The BBD as a response surface method is one of the experimental design methods, which is used to identify affecting factors in the synthesis process and to determine optimal conditions. BBD design includes a special subset of the factorial compounds from the 3 k factorial design where each factor has three levels. This design is rotatable (Oyinade et al. 2016; Dean et al. 2015).
In this study, based on the preliminary results of some experiments, a 7-factor BBD in Minitab 19 was used for the design experiments. Factors, which were found effective in the synthesis, are temperature, aging time, crystallization time, molar values of the main constituent elements such as silicon, aluminum, sodium, and molar value of water on the crystallinity percentage. The factors and their levels are given in Table 2. A BBD with 62 experimental runs was designed including, 6 replicate runs for the center point and 56 experiments for the non-center points.
If the desired response of BBD analysis is well modeled with a linear function of independent variables, the approximating function is called the first-order model. Otherwise, a higher-degree polynomial, like the second-order model must be applied. The mathematical relationship between the seven independent variables can be approximated using the following quadratic polynomial model (Eq. 1) (Montgomery 2017; Dean, et al. 2015).
where y represents the response variable (percentage of relative crystallinity) and xj’s are independent variables. The parameter β° is the constant of the model, βj’s are linear coefficients, βij’s are interaction coefficients, βjj’s represent the effects of quadratic, and ε is a random error that indicates the difference between the observed and predicted values (Montgomery 2017; Dean, et al. 2015).
The final model is fitted with experimental results. The model was examined in terms of model quality and then the analysis of variance (ANOVA) of the model was performed.
Synthesis of zeolite Y
In all the various methods reported for the synthesis of Faujasite (FAU) type crystals, the basis of the operation is hydrothermal treatment. In these methods, a precursor mixture is prepared which includes a base such as sodium hydroxide and two sources for aluminate and silicate. The precursor mixture is crystallized under definite time, temperature and autogenous pressure and is then turned into FAU crystals (Reiprich et al. 2020).
In this work, sodium silicate and sodium aluminate were used as sources of silicon and aluminum, respectively, to prepare the initial gel. The crystallization process was performed without the use of seed crystals and without stirring during the process. To prepare a solution of sodium aluminate, flake sodium hydroxide was dissolved in water. Then, aluminum hydroxide was added and the mixture was transferred to a balloon. The mixture was refluxed at 120 °C for 3 h. The synthesized solution was filtered to remove the impurities. The filtered sodium aluminate solution and sodium silicate were both diluted separately with distilled water. Diluted sodium aluminate solution was added to the diluted sodium silicate solution in a polyethylene container under vigorous stirring until non-crystalline aluminosilicate gel was formed. The obtained gel was aged at room temperature at different times. The prepared initial gel was transferred to a Teflon-lined stainless steel autoclave, and the crystallization step was carried out in an oven at the temperature range of 80°-94 °C. At the end of the crystallization time, the obtained zeolite was washed with distilled water to remove excess sodium ions and then dried in an oven at 120 °C. After drying, the final white powder was collected and stored in a sealed container.
Characterization and analysis
The X-ray diffraction patterns of the prepared samples were recorded in the range of 2θ = 5°–50° using an X-ray Multifunctional Diffractometer (XRD, XMD-300, Unisantis, Germany) with CuKa radiation source (λ = 1.54 Å, 40 kV).
After comparing the XRD graph peaks of the synthesized zeolites with the reference peaks for zeolite Y (D A 2013), the analysis of the reference peaks, including area, peak position and FWHM parameter (full width at half maximum) for zeolites Y was performed by using Origin software. Finally, the crystallinity percentage of synthesized zeolites Y was estimated using Eq. 2.
In Eq. 2, Ii, sample represents the area under the reference peaks (14 peaks, with a Bragg angle between 5 and 35 degrees) for the synthesized samples of zeolite Y and Ii, reference represents the area under the reference peaks for the standard zeolite Y. In this work, one of the synthesized samples that had the highest crystallinity was considered as the reference and the crystallinity of other samples was calculated in comparison to that (Akhoundzadeh et al. 2018; Soheili et al. 2021).
The crystal size was calculated using Scherrer’s equation (Eq. 3) (Patterson 1939). In this equation, parameter FWHM is used, which is obtained from the XRD graph.
In Eq. 3, L is the size of the crystal in nanometer, K is the shape factor for spherical crystals with cubic symmetry (K = 0.94) (Oyinade et al. 2016), λ is the wavelength of the diffraction (λ = 0.1541874 nm), B is the full width at half maximum (FWHM), and χ is equal to 2θ both in radians.
The morphology of the prepared samples was investigated using field emission scanning electron microscopy (FESEM, MIRA3, TESCAN, Czech Republic) operating at 10 kV. Fourier transform infrared spectrometer (FTIRZ, AVATAR 380, Thermo Fisher Scientific, USA) was used. X-ray fluorescence (XRF, PW-1410/70, PHILIPS, Netherland) analysis was done to study the composition of the prepared zeolites.
Results and discussion
Characterization
XRD patterns obtained for the samples were compared with the standard zeolite Y and it was concluded that, 50 out of 62 synthesized samples led to the production of zeolite Y. The rest were either zeolite P or amorphous. Some of the XRD graphs are given in Fig. 1. The results of the XRD test for all cases were given in Table 3. The ternary phase diagram (SiO2–Al2O3–Na2O) of zeolite Y synthesis is plotted in Fig. 2. This figure was plotted based on the composition of gels that formed zeolite Y, regardless of the synthesis conditions. The areas indicated by Lotus (Lutz 2014) for the formation of zeolite X and Y are also plotted in Fig. 2 and it can be claimed that this research has presented a larger area for the synthesis of zeolite Y.
As aforementioned, to calculate the crystallinity percentage, the area under the XRD peaks of the prepared zeolites should be figured out. For this purpose, 14 reference peaks were determined with the angles presented in Table 4 and the area under each peak was calculated after the baseline drawing and background removal. In the synthesized samples, sample 50, which had the highest intensity and maximum total area of 14 peaks, was selected as a good reference with the highest crystallinity. Figure 3 shows the XRD pattern of sample 50. The characterization of the specified 14 peaks for sample 50 is presented in Table 5. The main product type for 62 synthesized samples, relative crystallinity and the average crystal size for the prepared zeolites were reported in Table 3.
Effects of the molar value of silicon, sodium, aluminum and water on zeolite Y crystallization
Among all the influential factors on zeolite crystallization, it should be mentioned that gel composition has an essential and decisive role in determining the crystallized zeolite products (Cejka, et al. 2007; Kacirek and Lechert 1975). Figure 4 represents the effect of gel composition on the crystallinity percentage. Figure 4a shows that the low molar value of silicon resulted in low relative crystallinity, but in higher crystal size. Figure 4b displays that the low molar value of sodium resulted in high relative crystallinity and lower crystal size. Figure 4c also shows the relationship between relative crystallinity and crystal size with the molar value of aluminum. The changes in the molar value of aluminum do not affect the response. Figure 4d represents the effects of changes in the amount of water in gel composition over the crystallinity and crystal size. At the highest level of molar value of water, all points possess high crystallinity and low crystal size. Similar results have been reported by others. Kacirek et al. stated that the growth rate of Faujasite crystal is proportional to the concentration of SiO2 in the liquid phase and the aluminate concentration does not affect the crystallization rate (Kacirek and Lechert 1976). Kim et al. reported that increasing the molar value of water enhances the molar ratio of SiO2/Al2O3 in the zeolite structure. However, the formation of zeolite Y will be difficult in the presence of too much water (i. e. more than 500 mol), and in such case, more crystallization time will be required (Kim et al. 2009).
Figure 5 shows the relationship between the molar ratio changes in gel composition. Figure 5a shows that if SiO2/Al2O3 molar ratio is selected in the range of 8.3 to 14, the relative crystallinity above 90% can be reached. Li et al. demonstrated that by increasing the SiO2/Al2O3 molar ratio, the relative crystallinity is first increased and then remained almost constant. The relative crystallinity decreases due to an increase in the concentration of Silicon species, where its excess amount cannot be incorporated into the structure of zeolite Y. This extra amount precipitates as amorphous silicates (Li et al. 2010). Figure 5b shows that if Na2O/SiO2 molar ratio is selected in the range of 0.67 to 0.84, the relative crystallinity above 70% can be obtained. Li et al. reported that with increasing Na2O/SiO2 molar ratio, the relative crystallinity slightly increases at first and then decreases due to the dissolution of the crystal structure (Li et al. 2010). From Fig. 5c, it is depicted that to achieve high relative crystallinity, it is necessary to increase the molar value of water and decrease the molar value of Na2O. In Fig. 5c, it can be seen that zeolites with higher relative crystallinity will be formed in higher ratios of H2O/Na2O.
Effects of aging time, crystallization time and temperature on zeolite Y crystallization
Figure 4e shows that, at the bounds of aging time, relative crystallinity above 80% and crystal size of less than 60 nm can be achieved. According to the results obtained in Table 3, most of the samples with relative crystallinity of more than 90% had an aging time of 10 h. Oyinade et al. reported that the optimal time for aging, which had the highest relative crystallinity and crystal size, was 24 h (Oyinade et al. 2016). Kim et al. showed that 18 h is the most suitable aging time for obtaining nano zeolite Y crystals (Kim et al. 2009). Based on the results shown in Fig. 4f, most of the points with relative crystallinity above 90% had a crystallization temperature of 87 ºC. It can also be found that at the bounds of the temperature range, zeolite can be formed with high relative crystallinity. Figure 4g displays that at the lowest level of the crystallization time it is not possible to obtain a sample with relative crystallinity above 70% and it is necessary to select a higher level to achieve the desired response.
According to the obtained results, it is concluded that the gel composition has a very significant effect on the relative crystallinity of zeolite Y in comparison with the synthesis conditions. Therefore, the molar composition of the initial gel is the significant factor for achieving high relative crystallinity.
Response surface method (Box-Behnken design, BBD) for crystallinity study of synthesized zeolite Y
To achieve the mathematical relationship between the response factor (relative crystallinity percentage) and independent variables, F-test was used to carry out statistical testing of the model. The ratio of the total variability in the data is measured by R-squared as described by ANOVA. The response surface method was used to achieve the area in space in which a combination of various independent variables leads to zeolite Y. Finally, a point in the space was determined with a higher relative crystallinity percentage. The interaction effects of independent variables on the relative crystallinity are illustrated by cubic plots.
Percentage of relative crystallinity for zeolite Y
The relationship between response and independent variables was described by a quadratic polynomial model. Based on considering the significant terms and removing the non-significant terms, the final hierarchical model was presented by the Backward elimination method with α = 0.1 as follows:
The summary of the statistical parameters of the model is given in Table 6. The predicted R-squared of 0.9339 is in an advisable agreement with the adjusted R-squared of 0.9717. The signal-to-noise ratio is measured by adequate precision, which indicates an adequate signal since a ratio greater than 4 is desirable. The predicted error sum of squares (PRESS) which is presented in this table, is a criterion for accurate prediction of the model in new experiments. Table 7 shows the ANOVA results for fitting the quadratic polynomial model. Based on the results presented in Table 7 and considering the p-value of the model which is less than 0.0001 and the Lack of Fit p-value which is equal to 0.379 and is non-significant as a good criterion, it was concluded that this model can be applied to navigate the design space.
The results presented in Table 7 indicate that, in linear parameters, square parameters and interaction of independent variables, the effects of outstanding variables on response with a p-value less than 0.05 are significant.
The model F-value of 77.35 is remarkably larger than the reported F-distribution value of 1.95 at 95% significance (Montgomery 2017), which confirms the model’s efficiency in predicting the experimental results. The linear effect of the molar value of Si, Na and H2O and also the quadratic effect of Si molar value are the most significant terms on the relative crystallinity percentage of zeolite Y (p-value < 0.05). Furthermore, the interaction effects of some variables are also significant. The order of the variables affecting the relative crystallinity was ranked in Pareto analysis which is shown in Fig. 6. Factors with a standardized effect higher than 1.7 are considered effective. The contribution of linear, square and interaction effects of variables is, respectively, 86.6%, 3.05% and 8.79%.
Based on the quadratic polynomial model, the interaction effects of independent variables with a p-value less than 0.02, which have a significant effect on the relative crystallinity, are shown in Fig. 7 by 3D surface plots. In each 3D plot, two variables are changed while the rest of the variables are kept constant at their zero-coded level. As can be seen in Fig. 7a, with the increasing mole value of silicon, there is an increase in relative crystallinity, and simultaneously the change in mole value of aluminum has a very low effect on the response. Figure 7b shows the interaction effect of the mole value of sodium and the mole value of silicon on the response and indicates that to achieve a high relative crystallinity, the mole value of silicon must be increased while the mole value of sodium must be decreased. Figure 7c displays the positive effect of increasing both the mole value of water and silicon on relative crystallinity.
T3D surface plots of relative crystallinity (%) as a function of; a molar value of Al and Si, b molar value of Na and Si, c molar value of Si and H2O, d molar value of Si and crystallization time, e aging time and molar value of H2O, f aging time and crystallization temperature, g crystallization temperature and crystallization time
Figure 7d shows that with increasing the molar value of silicon and also crystallization time from 10 to 30 h, the relative crystallinity first increases and then decreases. Therefore, a high crystallinity percentage can be achieved in the middle range of 20 h. Figure 7e shows the interaction of the molar value of water and aging time, which indicates that in the shortest time for aging, i.e. 10 h, and considering the highest level for the molar value of water, nearly 100% relative crystallinity can be achieved. Figure 7f displays the simultaneous effect of crystallization temperature and aging time on response, which indicates that an increase in temperature and a decrease in aging time can enhance the response. Figure 7g shows the interaction effect of the temperature and the crystallization time on the response. In the investigated temperature range, as the crystallization time increases, the relative crystallinity first increases, and then, after 20 h, the relative crystallinity decreases rapidly. As a result, to achieve high relative crystallinity, a range of 20 h is suggested for crystallization time.
Determination of optimal conditions
According to the content expressed above, it can be concluded that if synthesis conditions and gel composition are optimized based on the obtained model, it is possible to obtain the zeolite with a relative crystallinity percentage higher than sample 50. In other words, there may be another zeolite Y in the study area that has an intensity and total area of 14 peaks higher than the sample 50. As shown in Table 8, the optimal values of independent variables were obtained by a response optimizer to achieve a relative crystallinity of 120% and also to take into account the reduction of both crystallization time and aging time.
The relative crystallinity of zeolite Y, obtained from the experiment at the optimal conditions shows a reasonable agreement with the predicted value by the BBD model. Based on the obtained results, it can be stated that the use of BBD to optimize the synthesis conditions and gel composition to achieve higher relative crystallinity in the study area, has been successful. Finally, the optimal sample was characterized by various analyses. Figure 8 shows the XRD pattern for optimal zeolite and zeolite sample no. 50. Based on the peak analyzer and the data presented in Table 4, it can be stated that the intensity of peak 1 for the optimal sample is higher than this peak for sample 50. Therefore, this difference increases the area under this peak or in other words optimal sample becomes more crystalline. The result of XRF analysis shows that the SiO2/Al2O3 ratio in the final zeolite Y is 3.24. The mass fraction of Na2O in zeolite was 0.121.
Figure 9 illustrates the FTIR pattern of optimal zeolite. The double ring vibration and symmetric stretching vibration of the Al–O–Si in the zeolite framework can be related to the faujasite characteristic bands which emerge from 569, 694 and 764 cm−1. Also, the band at 962 cm−1 is related to the asymmetric stretching vibration of AlO4 and SiO4 tetrahedral (Tang et al. 2017; Huang et al. 2010).
Figure 10 shows the FESEM images for the optimal zeolite, which represents particles with sizes of 1–3 μm and the spherical crystal morphology.
Conclusion
Zeolite Y was synthesized in an acceptable area of the gel composition. Also, the effect of more factors on the response was investigated compared to the previous studies of the researchers. The synthesis process was simple and with less equipment due to not using seed, and it was easy to control the process. The process and method of the synthesis are very cost-effective. In terms of the used raw materials, it is predicted that the method can be scaled up. According to the response surface method, among 62 synthesized samples, sample number 50 which has the highest intensity and the maximum total area of 14 peaks was selected as a good reference for studying the crystallinity of the other samples of synthesized zeolites Y. The effects of seven factors including the molar value of four components such as Si, Al, Na, H2O, aging time, crystallization time and crystallization temperature on the crystallinity of zeolite Y were investigated. Based on the model results and Pareto chart, it was found that the linear effects of the molar value of both Na and H2O as well as the linear, square, and interaction effects of the molar value of Si had the most effect on the response. The contribution of interaction effects in this model was remarkably 8.8%. According to the obtained model for relative crystallinity, the optimal zeolite Y was synthesized to reduce the aging and crystallization time to 10 and 20 h, respectively. At the optimal point, relative crystallinity of 117.5% in comparison with sample 50 was achieved. FESEM images indicated the production of spherical particles.
References
Akhoundzadeh H, Taghizadeh M, Pajaie HS (2018) Synthesis of highly selective and stable mesoporous Ni–Ce/SAPO-34 nanocatalyst for methanol-to-olefin reaction: Role of polar aprotic N, N-Dimethylformamide. Solvent Particuol 40:113–122
Bortolatto LB et al (2017) Synthesis and characterization of Y zeolites from alternative silicon and aluminium sources. Microporous Mesoporous Mater 248:214–221
Breck DW (1974) Zeolite molecular sieves. Wiley, New York, pp 313–320
Cejka J et al (2007) Introduction to zeolite molecular sieves. Elsevier
Cerutti MLN et al (2019) Copper-exchanged Y zeolites for gasoline deep-desulfurization. Adsorption 25(8):1595–1609
Chen M et al (2020a) Renewable P-type zeolite for superior absorption of heavy metals: Isotherms, kinetics, and mechanism. Sci Total Environ 726:138535
Chen C-T et al (2020b) Understanding the nucleation and crystal growth of zeolites: a case study on the crystallization of ZSM-5 from a hydrogel system under ultrasonication. J Phys Chem C 124(21):11516–11524
DA (2013) Standard Test method for determination of relative X-ray diffraction intensities of faujasite-type zeolite-containing materials. ASTM International West Conshohocken
Dean A et al (2015) Handbook of design and analysis of experiments, vol 7. CRC Press, Berlin
Dias da Silva P et al (2019) Adsorptive desulfurization of liquid fuels at elevated temperatures using metal exchanged zeolite Y. Ind Eng Chem Res 58(42):19623–19632
Garcia G et al (2016) Synthesis of zeolite Y from diatomite as silica source. Microporous Mesoporous Mater 219:29–37
Grand J, Awala H, Mintova S (2016) Mechanism of zeolites crystal growth: new findings and open questions. CrystEngComm 18(5):650–664
Hiroshi O, Yoshimasa A, Hiroshi U (1969) The Acidic Nature of Synthetic Zeolites: Acid Strength and Its Correlation with the Catalytic Activity. Bull Chem Soc Jpn 42(9):2449–2453
Huang Y et al (2010) Synthesis of hierarchical porous zeolite NaY particles with controllable particle sizes. Microporous Mesoporous Mater 127(3):167–175
Julbe A, Drobek M (2016a) Zeolite X-type. In: Drioli E, Giorno L (eds) Encyclopedia of membranes. Springer, Berlin
Julbe A, Drobek M (2016b) Zeolite Y type. In: Drioli E, Giorno L (eds) Encyclopedia of membranes. Springer,Berlin Heidelberg, Berlin, pp 2060–2061
Kacirek H, Lechert H (1975) Growth of the zeolite type NaY. J Phys Chem 79(15):1589–1593
Kacirek H, Lechert H (1976) Rates of crystallization and a model for the growth of sodium-Y zeolites. J Phys Chem 80(12):1291–1296
Kim YC et al (2009) Influencing factors on rapid crystallization of high silica nano-sized zeolite Y without organic template under atmospheric pressure. J Porous Mater 16(3):299–306
Kostinko JA (1983) Factors influencing the synthesis of zeolites A, X, and Y, G. D. Stucky and F. G. Dwyer, Eds., ACS Symposium Series 218, American Chemical Society, Washington, DC, USA pp. 3–19
Li Q et al (2010) Influence of synthesis parameters on the crystallinity and Si/Al ratio of NaY zeolite synthesized from kaolin. Pet Sci 7(3):403–409
Liu Z, et al (2016) A simple method of preparation of high silica zeolite Y and its performance in the catalytic cracking of cumene. J Nanotechnol
Louwen JN et al (2020) Role of rare earth ions in the prevention of dealumination of zeolite Y for fluid cracking catalysts. J Phys Chem C 124(8):4626–4636
Lutz W (2014) Zeolite Y: synthesis, modification, and properties—a case revisited. Adv Mater Sci Eng 2014(1):1–20
Ma B, Lothenbach B (2020) Synthesis, characterization, and thermodynamic study of selected Na-based zeolites. Cem Concr Res 135:106111
Montgomery DC (2017) Design and analysis of experiments. Wiley
Oyinade A, Kovo AS, Hill P (2016) Synthesis, characterization and ion exchange isotherm of zeolite Y using Box-Behnken design. Adv Powder Technol 27(2):750–755
Patterson A (1939) The Scherrer formula for X-ray particle size determination. Phys Rev 56(10):978
Reiprich B et al (2020) Layer-like FAU-type zeolites: A comparative view on different preparation routes. Front Chem Sci Eng 14(2):127–142
Sellaoui L et al (2021) Trapping of Ag+, Cu2+, and Co2+ by faujasite zeolite Y: New interpretations of the adsorption mechanism via DFT and statistical modeling investigation. Chem Eng J 420:127712
Soheili S, Pour AN, Mohammadi A (2021) SAPO-34 synthesis by combinations of structure-directing agents: experimental and Monte Carlo simulations studies. Microporous Mesoporous Mater 317:111003
Sulaiman KO, Sajid M, Alhooshani K (2020) Application of porous membrane bag enclosed alkaline treated Y-Zeolite for removal of heavy metal ions from water. Microchem J 152:104289
Tang T et al (2017) Organic template-free synthesis of zeolite Y nanoparticle assemblies and their application in the catalysis of the Ritter reaction. RSC Adv 7(13):7711–7717
Tian F et al (2014) Enhanced adsorption desulfurization performance over hierarchically structured zeolite Y. Fuel Process Technol 128:176–182
Xie M et al (2019) Enhanced catalytic performance of the FCC catalyst with an alumina matrix modified by the zeolite Y structure-directing agent. Ind Eng Chem Res 58(14):5455–5463
Acknowledgements
The authors of this work appreciate the financial support of the Ferdowsi University of Mashhad Research Council, Mashhad, Iran (Grant No. 3/51517).
Author information
Authors and Affiliations
Contributions
MRRT, MP, MK, AN and AM wrote the main manuscript text. All authors reviewed the manuscript.
Corresponding author
Ethics declarations
Conflict of interest
The authors declare that they have no known competing financial interests or personal relationships that could have appeared to influence the work reported in this paper.
Additional information
Publisher's Note
Springer Nature remains neutral with regard to jurisdictional claims in published maps and institutional affiliations.
Rights and permissions
Springer Nature or its licensor (e.g. a society or other partner) holds exclusive rights to this article under a publishing agreement with the author(s) or other rightsholder(s); author self-archiving of the accepted manuscript version of this article is solely governed by the terms of such publishing agreement and applicable law.
About this article
Cite this article
Ranjbar Toroghi, M.R., Panahi, M., Karimi, M. et al. Development of the crystallinity model and investigation of the effective factors on the crystallinity of zeolite Y using the response surface method. Phys Chem Minerals 50, 26 (2023). https://doi.org/10.1007/s00269-023-01251-8
Received:
Accepted:
Published:
DOI: https://doi.org/10.1007/s00269-023-01251-8