Abstract
In long-lived species, behaviour is expected to vary across the lifespan, first improving with maturation and experience and then declining with senescence, but measurement is rare, particularly during old age. Measuring nest defence intensity in 255 known-age blue-footed boobies (Sula nebouxii) of 19 age classes confirmed the inverted-U pattern: intensity of nest defence increased in young males and females until roughly 11–12 years and declined progressively afterwards. We discuss the processes that may generate this distinctive pattern. Regardless of the underlying processes, this study establishes the existence of age-related variation in parental care in a long-lived species. Natural variation in natal conditions has recently been shown to have long-term impacts on development, so this study raises the question whether age-related changes in parental care generate systematic variation in offspring phenotypes.
Significance statement
In theory, age-related change in parental care can result in differences among offspring produced at different ages. However, parental behaviour has never been measured over the whole age-span of any long-lived species because of the difficulty of sampling very old animals in the field. We measured intensity of nest defence over the age-span and confirmed that blue-footed boobies exhibit an age-related quadratic pattern in this parental behaviour: defence intensity increases in early life and declines progressively afterwards. Our results imply that similar-shaped patterns may exist in other components of parental care, such as feeding and social interaction, potentially affecting the long-term development of offspring.
Similar content being viewed by others
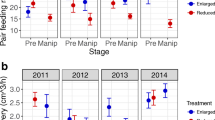
Avoid common mistakes on your manuscript.
Introduction
According to life history theory, trade-offs between growth, somatic maintenance and reproduction will occur throughout an individual’s life, affecting its fitness. Longitudinal studies of diverse taxa have shown that reproductive success, physiological function and survival tend to vary across the lifespan in a distinctive inverted-U pattern (Forslund and Pärt 1995; Ericsson et al. 2001; Berman et al. 2009). This suggests that fitness components first increase with age and experience (Forslund and Pärt 1995), probably due to maturation and learning (Robertson and Rendell 2001), then decline progressively with senescence (Jones et al. 2008; McNamara et al. 2009; Beamonte-Barrientos et al. 2010). In addition, long-lived species are expected to maximise lifetime fitness by strategically adjusting their reproductive effort and related behaviours across their lifetime (Stearns 2000). However, there is division of opinion over whether members of long-lived species maximise lifetime fitness by increasing (Clutton-Brock 1984) or reducing (McNamara et al. 2009) reproductive effort toward the end of the lifespan.
Behavioural traits should be sensitive indicators of both capability and willingness to invest but, to our knowledge, behaviour itself has never been measured over the whole age-span of any long-lived species because of the difficulty of sampling very old animals in the field. We investigated possible change across the lifespan in parental behaviour, potentially of great interest because it could have temporary or long-term effects on offspring development. A promising behaviour for doing this is the defence of progeny. In birds and mammals, avoiding hazards to the nest can be a major reproductive challenge because it involves elevated risk and, indeed, the intensity of nest defence is highly variable. It can increase with clutch age (Greig-Smith 1980), and it can be higher in males than females (Brunton 1990). Age-related increase in intensity of nest defence has been inferred in the California gull (Larus californicus; Pugesek 1983) and the great skua (Catharacta skua; Hamer and Furness 1991), but uncertainty over sampling of age classes and sexing of individuals leaves doubt over the shape of the relationship, particularly during old age.
To test for an inverted-U pattern in nest defence, we measured it over the age-span in a marked population of a long-lived marine bird where most individuals’ sexes and exact ages are known. In blue-footed boobies (Sula nebouxii), breeding traits such as hatching success, brood size and fledging have success peak at parental ages of 9–11 years and then decline (Drummond and Rodríguez 2015). There is also evidence that young and middle-aged adults strategically adjust their reproductive investment to their age, condition and natal environmental conditions (Velando et al. 2006; Ancona et al. 2011). Mean life expectancy is 10.01 ± 0.17 (± SE) and 9.55 ± 0.26 years for males and females, respectively, and the maximum recorded age is 25 years (HD, unpublished data).
Methods
Study species
The blue-footed booby lays one to three eggs on the ground and cares for the eggs during roughly 40–48 days and the chicks during 3–4 months. Throughout this time, the parents singly and jointly defend their nest and territory against conspecific and heterospecific intruders. In the study population, they regularly defend against Heermann’s gulls (Larus heermanni), Mexican spiny-tailed iguanas (Ctenosaura pectinata), feral cats (Felis silvestris catus, extinct since 1995; CONANP 2005), milksnakes (Lampropeltis triangulum nelsoni) and humans, using menaces, yes head-shakes, wing flails and, occasionally, violent pecks and jabbing or seizing and tossing (Nelson 1978; Sánchez-Macouzet and Drummond 2011). However, fearful boobies sometimes back away from a human or flee, leaving their nest temporarily exposed (HD, personal observations).
General methods
On Isla Isabel, Mexico (21° 52′ N, 105° 54′ W), between 6 and 16 March 2014, after the start of hatching, we recorded how 112 males 3–20 years old (\( \overline{X} \) = 9.08) and 143 females 3–25 years old \( \Big(\overline{X} \) = 8.65) defended against human intruders. In nesting areas with few courting pairs at 7.00–13.00 and 15.00–18.00 hours, two observers haphazardly sampled boobies ringed at fledging that were caring singly (at that moment) for progeny at 255 nests (240 with eggs, 15 with chicks). No neighbours within a 10-m radius of any focal nest were tested; local nest densities within this radius ranged from 0 to 41 nests (\( \overline{X} \) = 13.09).
After identifying each subject’s sex (females grunt, males whistle; Nelson 1978), both observers slowly and silently walked in single file toward the nest, approximately 50 cm apart. The rear observer stopped 6 m from the nest and recorded the subject’s behaviour. The leading observer continued approaching, looking the subject in the eyes, then stopped 50 cm from the nest and waited for 5 s. If the subject fled, exposing its nest, both observers immediately withdrew 10 m from the nest until the subject’s return; eggs were covered with leaves to conceal them from gulls. All fleeing boobies returned to their nests within a minute and no clutch or brood was lost nor abandoned as a result of the tests.
On the basis of each subject’s response to the approach, the intensity of its defence was assigned to one of seven categories (in ascending order): (1) fled when the observer was > 5 m from the nest or (2) < 5 m from it, (3) uncovered its clutch but remained within 1 m of the nest, (4) remained on the nest without making vocal threats, (5) remained on the nest and started making vocal threats when the observer was 0.75–3.4 m distant or (6) 3.5–6 m distant or (7) remained on the nest and pecked toward the observer. The seven categories were assigned 0–6 points, respectively; each subject was allocated to the highest category it performed. Data were recorded blindly; identities/ages of subjects were not known at the time of testing or assigning response categories.
Statistical analyses
To analyse the relationship between nest defence and parental age, we built a linear mixed model (LMM), including six additional predictors that might influence defence, using an information-theoretic approach (Burnham and Anderson 2002). All variables were standardized prior to model fitting to minimize linear and quadratic terms’ collinearity and to facilitate the interpretation of the relative strength of parameter estimates (Grueber et al. 2011; Cade 2015). Fixed effects comprised the linear and quadratic expressions of the focal bird’s age, sex, nest content (clutch/brood), brood size, local nest density (number of nests in a 10-m radius), linear and quadratic expressions of time of day and date of the test. Nest content and brood size were included to control for their possible effects on investment in defence; local nest density was included to account for neighbours’ possible influence on the subjects’ responses to an intrusion, and time of day was included to control for possible circadian changes in behaviour.
We fitted the models with a Gaussian error distribution and an identity link function, including nest identity as a random effect because both members of the pair were studied in 37 cases. Rather than fitting models for all possible combinations of predictors, we generated 19 hypothesis-based candidate models (plus a null model), ranked from “best” to “worst” using Akaike’s information criterion corrected for small samples (AICc). We then computed Akaike weights to estimate the relative support in the data for each of the fitted models (Burnham and Anderson 2002). Based on these Akaike weights, we averaged the fits of a subset of the best-fitting models which, together, had a cumulative Akaike weight (w i) of ~ 0.95 (Table 1). This multi-model averaging approach accounts for model selection uncertainty and reduces model selection bias by considering the relative support for each competing model, thus providing more robust parameter estimates than those obtained from any single-model approach (Burnham and Anderson 2002). Additionally, we repeated the analysis after deleting an extremely old bird (25 years) from the sample to test whether it influenced the regression coefficients of age and age2 (Supplementary material, S1).
All analyses were performed in R statistical environment (v3.3.3) (R Development Core Team 2017). We used lmer function in lme4 package (Bates et al. 2016) to construct our model. To standardize all model variables we used rescale function in package arm (Gelman et al. 2016). We used the mod.avg function to average models within a 95% confidence interval (CI) (Burnham et al. 2011) in MuMIn package (Bartón 2016) and confint function to calculate the CI of each parameter.
Results
We used the six best-fitting models (Table 1) for the model averaging procedure. Age and age2 were the predictors of nest defence intensity with the highest relative support in the data (both of 1), and the only terms whose regression coefficients were statistically different from zero (β = 0.87 and 95% confidence interval (CI) = 0.42 to 1.32 and β = − 1.37, CI = 2.01 to − 0.73, respectively; Table 2; Fig. 1). There was low relative support for the linear and quadratic expressions of time of day, nest content, date of test, local nest density and brood size (Table 2). After excluding the 25-year-old bird, only the regression coefficient of the quadratic relationship between parental age and nest defence intensity remained statistically different from zero; its relative support was diminished and the pattern of increase followed by decline in defence with age was more symmetrical (Supplementary material, S2, S3, S4, S5). To test whether the decline in defence in old age was supported by the data, we re-applied the model selection procedure to the sample of boobies older than 11 years (the age at which reproductive variables peak; Beamonte-Barrientos et al. 2010; Drummond and Rodríguez 2015), including the factors retained in the averaged model except the quadratic expression of age. This analysis confirmed the linear decrease in nest defence intensity between ages 12 and 25 years (β = − 0.57, CI = − 1.39 to − 0.03; Supplementary material, S6, S7, S8).
Nest defence intensity according to parental age. Black dots denote mean nest defence of sampled age classes with standard errors. Solid line represents model prediction with 95% confidence intervals (in dashed lines). Dot size is related to number of birds per age class. Sample sizes, from left to right, are 17, 10, 8, 63, 39, 31, 5, 12, 1, 6, 5, 13, 21, 10, 7, 1, 2, 3 and 1
Discussion
The intensity with which blue-footed boobies of Isla Isabel defended their nests against human intruders varied with parental age, increasing up to roughly age 11–12 years and declining thereafter. This quadratic relationship between parental age and nest defence was independent of sex, nest content, brood size, local nest density, time of day and test date. After the removal of a 25-year-old bird from the sample, the U-pattern remained, but the decline with old age was less well supported; widening of the confidence interval at old ages admits the possibility that nest defence plateaus in late life, as observed in other species (Angelier et al. 2007; Berman et al. 2009), rather than declining. Nonetheless, the combined analyses of the complete data set and the subset for ages 12–25 years supported a pattern of increase in early life and decline in old age, and the confidence intervals showed that late-life decline is unlikely to be absent and may be steep. To our knowledge, this is the first study to report directly observed parental behaviour of known-age individuals across the entire natural age-span of a long-lived species in the wild.
The increase in intensity of nest defence through roughly age 11–12 years is most readily explained by maturation and learning (Robertson and Rendell 2001): older birds with more experience in pairing, territory acquisition, foraging and breeding (including nest defence) may simply be more able to prudently mount a strong defence. Similarly, progressive reduction in nest defence intensity in old age is most readily explained by senescent decline in those same capabilities. However, this species’ age-related curves of responsiveness to human intruders (an inverted-U; this study) and to experimentally standardised conspecific intruders (no effect across ages 5–13 years; Sánchez-Macouzet and Drummond 2011) differ, implying that strategy is also involved.
Sampling of ages was cross-sectional, so the observed increase and decrease in nest defence could alternatively be explained by differential survival of individuals that differed in defensiveness (and possibly in other reproductive traits; Forslund and Pärt 1995; McCleery et al. 2008) or even by differences among birth cohorts. It is difficult to construct a plausible hypothesis based on such effects that can account for the whole inverted-U pattern, but the decline in old age could in theory arise from high mortality among more aggressive individuals. Strategic modification of resource allocation with age could partially account for the inverted U-shaped curve and also for the high variation in nest defence in old blue-footed boobies, which sometimes increase investment in their last reproductive event (Torres et al. 2011). Complete accounting for the shape of the whole inverted-U pattern may well require consideration not only of maturation, learning and senescence but also of differential survival of varied phenotypes and strategic adjustments of resource allocation.
Discovery of this booby’s age-related U-shaped pattern of defence implies that similar-shaped patterns may exist in other components of parental care such as incubation, nestling provisioning and social interactions, with potentially important consequences for offspring characteristics and parental fitness. Similarly, other animal species may show age-related linear or quadratic patterns in components of parental care, resulting in differences in offspring produced at different ages. Avian and mammalian offspring raised in poor ecological conditions in nature have shown a panoply of effects on their adult survival, reproduction and ageing, including adoption of alternative life histories (Nussey et al. 2007; Drummond and Ancona 2015; Douhard et al. 2016) and, in principle, age-related variation in parental care could generate similar developmental effects. Effects of parental age over the age-span on offspring growth and viability have recently been uncovered (Torres et al. 2011; Drummond and Rodríguez 2015; Preston et al. 2015), but to our knowledge, the possibility of age-related change in parental care itself affecting the characteristics of offspring has not been directly tested in any species.
References
Ancona S, Sánchez-Colón S, Rodríguez C, Drummond H (2011) El Niño in the warm tropics: local sea temperature predicts breeding parameters and growth of blue-footed boobies. J Anim Ecol 80:799–808
Angelier F, Moe B, Weimerskirch H, Chastel O (2007) Age-specific reproductive success in a long-lived bird: do older parents resist stress better? J Anim Ecol 76:1181–1191
Bartón K (2016) Multi-model inference. R package version 1.15.6, https: //CRAN.R-project.org/package=MuMIn
Bates D, Maechler Martin, Walker S (2016) Package “lme4”: linear mixed-effects models using “Eigen” and S4, https://github.com/lme4/lme4/ http://lme4.r-forge.r-project.org/
Beamonte-Barrientos R, Velando A, Drummond H, Torres R (2010) Senescence of maternal effects—aging influences egg quality and rearing capacities of a long-lived bird. Am Nat 175:469–470
Berman M, Gaillard J-M, Weimerskirch H (2009) Contrasted patterns of age-specific reproduction in long-lived seabirds. Proc R Soc Lond B 276:375–382
Brunton D (1990) The effects of nesting stage, sex, and type of predator on parental defense by killdeer (Charadrius vociferous): testing models of avian parental defense. Behav Ecol Sociobiol 26:181–190
Burnham KP, Anderson DR (2002) Model selection and multi-model inference: a practical information-theoretic approach, 2nd edn. Springer, New York
Burnham KP, Anderson DR, Huyvaert KP (2011) AIC model selection and multimodel inference in behavioral ecology: some background, observations, and comparisons. Behav Ecol Sociobiol 65:23–35
Cade BS (2015) Model averaging and muddled multimodel inferences. Ecology 96:2370–2382
Clutton-Brock TH (1984) Reproductive effort and terminal investment in iteroparous animals. Am Nat 123:212–229
CONANP (2005) Programa de conservación y manejo del Parque Nacional Isla Isabel. Conanp-Semarnat, México
Douhard M, Loe LE, Stien A, Bonenfant C, Irvine RJ, Veiberg V, Ropstad E, Steve Albon S (2016) The influence of weather conditions during gestation on life histories in a wild Arctic ungulate. Proc R Soc B 283:20161760
Drummond H, Ancona S (2015) Observational field studies reveal wild birds responding to early-life stresses with resilience, plasticity, and intergenerational effects. Auk 132:563–576
Drummond H, Rodríguez C (2015) Viability of booby offspring is maximized by having one young parent and one old parent. PLoS One 10:e0133213
Ericsson G, Wallin K, Ball JP, Broberg M (2001) Age-related reproductive effort and senescence in free-ranging moose, Alces alces. Ecology 82:1613–1620
Forslund P, Pärt T (1995) Age and reproduction in birds—hypotheses and tests. Trends Ecol Evol 10:374–378
Gelman A, Su Y-S, Yajima M, Hill J, Pittau MG, Kerman J, Zheng T, Dorie V (2016) Data analysis using regression and multilevel/hierarchical models, https://cran.r-project.org/web/packages/arm/index.html
Greig-Smith PW (1980) Parental investment in nest defence by stonechats (Saxicola torquata). Anim Behav 28:604–619
Grueber CE, Nakagawa S, Laws RJ, Jamieson IG (2011) Multimodel inference in ecology and evolution: challenges and solutions. J Evol Biol 24:699–711
Hamer KC, Furness RW (1991) Age-specific breeding performance and reproductive effort in Great Skuas Catharacta skua. J Anim Ecol 60:693–704
Jones OR, Gaillard J-M, Tuljapurkar S et al (2008) Senescence rates are determined by ranking on the fast-slow life-history continuum. Ecol Lett 11:664–673
McCleery RH, Perrins CM, Sheldon BC, Charmantier A (2008) Age-specific reproduction in a long-lived species: the combined effects of senescence and individual quality. Proc R Soc Lond B 275:963–970
McNamara JM, Houston AI, Barta Z, Scheuerlein A, Fromhage L (2009) Deterioration, death and the evolution of reproductive restraint in late life. Proc R Soc Lond B 276:4061–4066
Nelson JB (1978) The Sulidae: gannets and boobies. Oxford University Press, Oxford
Nussey DH, Kruuk LEB, Morris A, Clutton-brock TH (2007) Environmental conditions in early life influence ageing rates in a wild population of red deer. Curr Biol 17:1000–1001
Preston BT, Saint Jalme M, Hingrat Y, Lacroix F, Sorci G (2015) The sperm of aging male bustards retards their offspring’s development. Nat Commun 6:6146
Pugesek BH (1983) The relationship between parental age and reproductive effort in the California Gull (Larus californicus). Behav Ecol Sociobiol 13:161–171
Development Core Team R (2017) R: a language and environment for statistical computing. R Foundation for Statistical Computing, Vienna, Austria http://www.R-project.org/
Robertson RJ, Rendell WB (2001) A long-term study of reproductive performance in tree swallows: the influence of age and senescence on output. J Anim Ecol 70:1014–1031
Sánchez-Macouzet O, Drummond H (2011) Sibling bullying during infancy does not make wimpy adults. Biol Lett 7:869–871
Stearns SC (2000) Life history evolution: successes, limitations, and prospects. Naturwissenschaften 87:476–486
Torres R, Drummond H, Velando A (2011) Parental age and lifespan influence offspring recruitment: a long-term study in a seabird. PLoS One 6:e27245
Velando A, Drummond H, Torres R (2006) Senescent birds redouble reproductive effort when ill: confirmation of the terminal investment hypothesis. Proc R Soc Lond B 273:1443–1448
Acknowledgements
We thank numerous volunteers for enthusiastic work in the field and on the database; Alejandro Gonzalez and Lynna Kiere for statistical advice; and the Armada de México, staff of SEMARNAT and local fishermen for logistical support. For comments on the manuscript, we thank Sergio Ancona, Péter Szenczi and Oxána Bánszegi, as well as two anonymous reviewers.
Funding information
This study was funded by Universidad Nacional Autónoma de México (PAPIIT grant IN205313).
Author information
Authors and Affiliations
Corresponding author
Ethics declarations
Conflict of interest
The authors declare that they have no conflict of interest.
Ethical approval
All applicable international, national and/or institutional guidelines for the care and use of animals were followed. The Secretaría de Medio Ambiente y Recursos Naturales (SEMARNAT) provided the permit for this study (SGPA/DGVS/10856/13).
Additional information
Communicated by J. A. Graves
Data availability
All data generated or analysed during this study are included in this published article and its supplementary information files.
Rights and permissions
About this article
Cite this article
Ortega, S., Sánchez-Macouzet, O., Urrutia, A. et al. Age-related parental care in a long-lived bird: implications for offspring development. Behav Ecol Sociobiol 71, 132 (2017). https://doi.org/10.1007/s00265-017-2364-7
Received:
Revised:
Accepted:
Published:
DOI: https://doi.org/10.1007/s00265-017-2364-7