Abstract
Neurogenic inflammation including calcitonin gene-related peptide (CGRP) and substance-P (SP) release plays a pivotal role in migraine pathogenesis. Prevalence of migraine is ~ 3 folds higher in women than in men, but its underlying mechanisms remained unclear. We investigated the effects of female sex hormones estrogen and progesterone on CGRP and SP in in-vivo and ex-vivo in rats of both sexes. For in-vivo experiments, male, female and ovariectomized rats were separated into four groups (n = 7) as control, estrogen, progesterone and estrogen + progesterone, respectively. Groups received daily intraperitoneal vehicle, 17β-estradiol, progesterone and 17β-estradiol + progesterone for 5 days, respectively. For ex-vivo experiments in both sexes, isolated trigeminal ganglia and hemiskull preparations were divided into four groups (n = 6 or 8), respectively, as in-vivo groups, and administered the same test substances. CGRP and SP contents in plasma and superfusates were determined using ELISA. In in-vivo experiments, 17β-estradiol decreased CGRP levels in males and SP levels in ovariectomized rats. Progesterone increased both CGRP and SP levels in females. Their combination decreased both CGRP and SP levels in males, and only SP levels in ovariectomized rats. In ex-vivo experiments, 17β-estradiol reduced CGRP release in males and SP release in females in trigeminal ganglia. While progesterone increased CGRP release in trigeminal ganglia, it reduced SP release from hemiskulls in both sexes. Their combination restored progesterone-mediated changes in neuropeptides releases in both trigeminal ganglia and hemiskulls in both sexes. Estrogen alleviates neurogenic inflammation through modulation of CGRP and SP release. Progesterone has dual effects on these neuropeptides in different sites associated with migraine pain.
Similar content being viewed by others
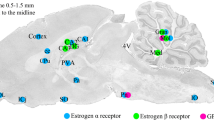
Avoid common mistakes on your manuscript.
Introduction
Migraine is one of the most disabling neurovascular disorders characterized by unilateral recurrent head pain often accompanied by nausea, vomiting, photophobia and phonophobia. Migraine has a complex pathophysiology, however, it is well established that neurogenic inflammation characterized by the release of vasoactive neuropeptides such as calcitonin gene-related peptide (CGRP) and substance P (SP) from trigeminal innervation plays a central role in this condition (Ramachandran 2018; Kilinc et al. 2020a). Cranial meninges are densely innervated by trigeminal nociceptive afferent nerve fibres. CGRP and SP released from these afferent terminals as a result of the meningeal irritation contribute to the neurogenic inflammation process by inducing plasma protein extravasation, vasodilatation, and degranulation of meningeal mast cells (Kilinc et al. 2018). Vast majority of previous studies, with a few exceptions, reported that plasma CGRP levels were elevated during acute migraine attacks or headache-free periods in migraineurs (Goadsby et al. 1990; Fusayasu et al. 2007; Ashina et al. 2000; Frederiksen et al. 2020). Moreover, recently, two systematic reviews and meta-analyses have reported that CGRP levels were elevated in cerebrospinal fluid and/or plasma of chronic migraine patients (van Dongen et al. 2017; Frederiksen et al. 2020).
The prevalence of migraine is two to three times higher in women than in men, but the mechanisms associated with this gender difference are poorly understood (Vetvik and MacGregor 2017; Labastida-Ramírez et al. 2019). It was suggested that the hormonal fluctuations of female gender hormones such as estrogens and progesterone may be responsible for this gender-dependent prevalence in migraine (Labastida-Ramírez et al. 2019). In relation to gender difference, estrogen withdrawal during the menstrual cycle has widely been accepted to play a role in migraine. However, since there is a gap in this field with very few studies in the literature, new studies are of great importance.
It was reported that a decrease in plasma estrogen levels during premenstrual phase of the menstrual cycle induces migraine attacks in the predisposed women (Pavlović et al. 2016; Somerville 1972). On the contrary, progesterone withdrawal does not seem to remit migraine attacks (Somerville 1972). Somerville demonstrated that intramuscular administration of 10 mg estradiol to women suffering from migraine during the perimenstrual phase of the menstrual cycle postponed the onset of migraine attacks (Somerville 1972).
In addition, it was reported that circulating CGRP levels could be affected by the menstrual cycle, pregnancy and menopausal states (Cupini et al. 2020). In a clinical study with healthy women, it was reported that the highest trigeminal CGRP release to blood circulation was detected around the menstruation when estrogen levels were low (Ibrahimi et al. 2013). In an experimental study, ovariectomy increased the gene expression of CGRP in trigeminal ganglion tissues, and this effect was substantially reduced by estrogen replacement to the ovariectomized rats (Aggarwal et al. 2012). Moreover, in cultured female rat trigeminal neurons, administration of physiological doses of estrogen down-regulated bradykinin B2 receptor and IL-1β receptor which are potentially relevant to CGRP release from sensory neurons (Puri et al. 2006). In addition, CGRP treatment to the rat dura mater increased cutaneous periorbital hypersensitivity in behavioral model of migraine (Avona et al. 2019).
Thus, it is known that female sex hormones are involved in the pathophysiology of migraine, particularly in women sufferers, but their exact mechanisms of action remain unclear. Moreover, there are currently no treatments peculiar to menstrual-related migraine in women sufferers.
To develop new treatment options specific to menstrual- or female sex hormones-associated migraine, there is primarily a need to clarify the mechanisms of action of these hormones involved in the pathophysiology of migraine. It is well known that estrogen and progesterone receptors are expressed in the parts of trigeminovascular system, which is a central site in the generation of migraine pain, including cranial vasculature and the meninges (Giuffre et al. 1992; Zuloaga et al. 2012). Therefore these structures involved in migraine are potential targets to investigate the effects of estrogen and progesterone in the modulation of CGRP and SP release. However, a little is known about the impacts of female sex hormones on the CGRP and SP releases in trigeminovascular system (Labastida-Ramírez et al. 2019). Therefore, the present study attempted to fill such above-mentioned gaps regarding the mechanisms of action of these hormones in migraine.
In the present study, we used young adult rats weighing 200–250 g, because (1) such reproductive-age rats have maximum levels of sex hormones, and (2) they provide an opportunity to investigate how endogenous and exogenous application of female sex hormones may influence CGRP and SP levels in the trigeminovascular system and plasma. In accordance with this purpose, we investigated effects of estrogen and progesterone on the release of CGRP and SP biomarkers of neurogenic inflammation underlying migraine pain in the in-vivo as well as in the isolated rat trigeminal ganglia and meningeal trigeminal nerve terminals using ex-vivo preparations in rats of both sexes.
Background at the focus of the study
We focused on effects of estrogen and progesterone on the basal releases of CGRP and SP, instead of the stimulated-releases, using in-vivo and ex-vivo experimental models using rats of both sexes, mainly for the following reasons: (1) to reveal, first of all, fundamental physiological mechanisms of effects of female sex hormones fluctuations on the releases of CGRP and SP in the trigeminovascular system and plasma, (2) in this way to better understand the effects of female sex hormones fluctuations in the pathophysiology underlying gender-dependent difference in migraine, (3) to fill the gap in the literature on this issue.
Materials and methods
Animals
All experiments were approved by Bolu Abant Izzet Baysal University Animal Experiments Local Ethics Committee (licence number 2017/45). Wistar rats of both sexes weighing 200–250 g were used in the experiments. Rats were supplied from the Laboratory Animals Research and Application Centre of Bolu Abant Izzet Baysal University, Turkey. Rats were maintained in keeping with the Guide for the Care and Use of Laboratory Animals (Eighth Edition). Rats were given ad libitum with a standard rodent diet and tap water and were housed in spacious cages with a 12 h light/dark cycle at 22 ± 2 °C.
In-vivo experimental groups
A total of 107 rats were used in the in-vivo experiments. 35 of these 107 rats were male, and the others were female. While 28 of 35 male rats were used to produce four subgroups (n = 7 for each subgroup) including control (vehicle), estrogen, progesterone and estrogen + progesterone. The remaining seven rats were used as a control to compare with a control group of the four subgroups with ketamine anaesthesia (n = 7 for each subgroup) and, therefore, these seven rats were exposured CO2 inhalation shortly before blood collection. 35 of 72 female rats were used for the same procedures that male rats were exposed. 35 of the remaining 37 female rats (n = 7 for each subgroup) were used for ovariectomy procedure and afterwards for the same procedures as described above. Two rats died following the ovariectomy.
Rats were randomly allocated into 3 main groups, each containing four subgroups as follows: intact male (n = 28 in total), intact female (n = 28 in total) and ovariectomized female (n = 28 in total). We constituted both intact and ovariectomized female groups to distinguish the effects of endogenous and exogenous hormonal applications on the CGRP and SP releases. Each of the general groups including intact male, intact female and ovariectomized female were also randomly separated into four subgroups (n = 7 for each subgroup) as follows: control, estrogen, progesterone and estrogen + progesterone. All injections were administered through intraperitoneal route. Control group received 0.2 mL of 10% ethanol as a vehicle, estrogen group received 1 mg/kg 17β-estradiol, progesterone group received 8 mg/kg progesterone, estrogen + progesterone group received 1 mg/kg 17β-estradiol plus 8 mg/kg progesterone daily for 5 days, respectively. In-vivo groups and their experimental procedures are illustrated in Fig. 1.
Moreover, to ensure whether ketamine anaesthesia that was performed shortly before collection of the blood samples at the end of the experiments may affect the CGRP and SP levels, we produced additional three control groups for intact male, intact female and ovariectomized female groups that were received 10% ethanol as a vehicle (n = 7 for each group). Blood samples from these control groups were collected following CO2 inhalation instead of ketamine administration, contrary to the other all groups which were anaesthetized with ketamine. There was no difference between these controls and ketamine administered controls in terms of CGRP and SP levels (supplementary Fig. 1).
Ex-vivo experimental groups
A total of 42 rats were used in the ex-vivo experiments. 18 of these 42 rats were male, and the other 24 rats were female. Nine of 18 male rats were used to prepare isolated trigeminal ganglia preparations that were divided into three groups (n = 6 trigeminal ganglia for each group). The other nine male rats were used to prepare hemiskull preparations (n = 6 hemiskulls for each group). Please note, two preparations were prepared from each rat due to bilateral nature of the structures including trigeminal ganglion and hemiskull. 12 of 24 female rats were used to prepare isolated trigeminal ganglia preparations that were divided into three groups (n = 8 trigeminal ganglia for each group). The other 12 female rats were used to prepare hemiskull preparations (n = 8 hemiskulls for each group). Both male and female rats were randomly allocated to the groups listed below in detail, respectively. But, all-female rats that were allocated to the groups were chosen at the same phase (proestrus) of the estrous cycle, as it was performed in-vivo experiment, as described below.
Isolated trigeminal ganglia and hemiskull preparations from both male and female rats were randomly divided into three groups (n = 6 both trigeminal ganglia and hemiskulls for males, n = 8 both trigeminal ganglia and hemiskulls for females in each group), respectively, as follows: estrogen, progesterone, and estrogen + progesterone. Isolated trigeminal ganglia and hemiskull preparations were topically treated with 0.1% ethanol in artificial cerebrospinal-fluid (ACSF, pH 7.4) for the control group, 1 μM 17β-estradiol in ACSF for estrogen group, 1 μM progesterone in ACSF for progesterone group, and 1 μM 17β-estradiol plus 1 μM progesterone in ACSF for estrogen + progesterone group, respectively. Ex-vivo groups and their experimental procedures are illustrated in Fig. 2.
Drugs and reagents
β-Estradiol, progesterone, phosphate-buffered saline and cOmplete™ protease inhibitor cocktail were purchased from Sigma-Aldrich, (Schnelldorf, Germany). CGRP and SP ELISA kits were purchased from Bioassay Technology Laboratory (Shanghai, China). 17β-estradiol and progesterone were dissolved in ethanol for all of in-vivo and ex-vivo experiments.
In-vivo experimental interventions
Ovariectomy
Rats were anaesthetized by intraperitoneal injection of ketamine (90 mg/kg, Ketalar; Pfizer, New York, NY) and xylazine (5 mg/kg; Rompun; Bayer, Leverkusen, Germany). After the skin was shaved off, bilateral dorsolateral incisions were carried out through the skin. The lower muscle tissues were dissected to locate the ovaries and fallopian tubes, which were ligated with a 4/0 silk suture, then the ovaries were removed except in the sham-control group. The ovariectomized (OVX) rats were housed in their individual cages and observed for a period of twenty-one days for any mortality or disease without any intervention. Two rats that died during this duration following surgical operations were excluded from the experiments. The others did not show any symptomps. After twenty-one days, drug administration was performed to the groups for 5 days.
Identification of the rat estrous cycle in the intact female group
In rats, an estrous cycle is composed of four discrete stages: proestrus, estrus, metestrus and diestrus. To determine the stages of the estrous cycle, vaginal secretion was collected twice a day, one in the morning (8 h) and one in the afternoon (16 h), for five consecutive days. Due to the short duration of the estrous phases, daily collections were carried out with an interval of 8 h to achieve an exact classification of each phase. Briefly, vaginal secretion was collected with a sterile plastic pipette filled with 10 µL of normal saline (NaCl 0.9%) by gently introducing the tip into the rat vagina (but not deeply). Vaginal fluid was placed on glass slides. After preparations were air-dried, Pap staining was used to distinguish cells in smear preparations. The classification of the estrous cycle was performed by observing the presence of leukocytes, cornified epithelial cells, and nucleated epithelial cells in the fluid. The representative images of vaginal fluid cytology and stages of the estrous cycle are shown in supplementary Fig. 2.
Drug administrations in the in-vivo groups
Rats in intact male, intact female and OVX female groups were treated intraperitoneally with the same drugs and concentrations. Control groups received 0.2 mL of 10% ethanol in saline as a vehicle, estrogen groups received 1 mg/kg 17β-estradiol (Li et al. 2016), progesterone groups received 8 mg/kg progesterone (Andrabi et al. 2017), estrogen + progesterone groups received 1 mg/kg 17β-estradiol plus 8 mg/kg progesterone daily for 5 days, respectively. To eliminate any variability caused by different phases of the menstrual cycle, we started all injections at the same phase (proestrus) of the estrous cycle in all rats of the intact female group. Moreover, such a design could allow to make a rational comparison between the ovariectomized group and intact female group due to endogenous estrogen and progesterone when they are at the highest level during proestrus stage of the estrous cycle. To achieve this, firstly, female rats were held together at two identical cages with 14 rats for a duration of one month. Thereafter, estrous cycle phases for each rat was determined as described above. Finally, on the sixth day of the experiments, blood collection from all rats in intact female group were carried out at proestrus phase of the estrous cycle.
Blood collection from in-vivo groups
Five days after drug administrations (on the 6th day), rats were anaesthetized with ketamine (90 mg/kg, i.p.) except for the additional three control groups mentioned above that were applied CO2 inhalation. Shortly after ketamine administration or CO2 inhalation, the chest of rats was opened in a V-shape by scissors, and the heart was exposed. The tip of a sterile 5 mL syringe was gently introduced into the right ventricle, and the blood was collected from right ventricle by the suction with the syringe. Blood samples were instantly mixed with protease inhibitor cocktail to prevent degradation of CGRP and SP in a tube and centrifuged at 3000×g for 15 min at 4 °C. The supernatants were stored at − 80 °C for a week. Then they were assayed for CGRP and SP immunoreactivities by ELISA.
Ex-vivo experimental interventions
To determine CGRP and SP releases in series of ex-vivo experiments, we used two well-established methods including isolated trigeminal ganglia (Eberhardt et al. 2008) and hemiskull preparations (Ebersberger et al. 1999; Kilinc et al. 2017a).
Ex-vivo meningeal preparations
To determine CGRP and SP releases from the peripheral terminals of meningeal afferents innervating cranial dura mater that is the origin site of migraine pain, we prepared the hemisected skulls with intact dura mater and trigeminal ganglia from both male and female rats, as described previously (Kilinc et al. 2017a, 2020a). Shortly, the skull halves were superfused for 30 min with carbogen-gased (with 95% O2; 5% CO2) artificial cerebrospinal fluid (ACSF) containing (in mmol/L): KCl 3, NaCl 115, MgCl2 1, CaCl2 2, NaH2PO4 1, NaHCO3 25 and glucose 11 (pH 7.4) at room temperature. The hemiskull cavities were filled with 0.1% ethanol in ACSF for controls or the test substances (1 μM 17β-estradiol (Fatehi et al. 2008) for estrogen group, 1 μM progesterone (Masuyama and Hiramatsu 2011) for progesterone group, and 17β-estradiol + progesterone for estrogen + progesterone group) and incubated for 15 min in a humid incubator at 37 °C. After 15 min, 250 μL of superfusates was collected and transferred to the tubes with a protease inhibitor cocktail (cOmplete, Sigma-Aldrich). The samples were stored at − 80 °C until assayed for CGRP and SP immunoreactivities.
Isolated trigeminal ganglia preparations
To investigate CGRP and SP releases from trigeminal ganglia that is the origin of CGRP and SP expression, we prepared isolated trigeminal ganglia preparations from both male and female rats as described previously (Eberhardt et al. 2008). Briefly after the head was cut, the brain was removed and the skull halves were plunged in ACSF with carbogen-gased (with 95% O2; 5% CO2, pH 7.4). Under stereo microscope, the trigeminal ganglia were harvested through dissecting 1 mm proximal and distal to the branching point of the mandibular nerve, then the dura was carefully removed. The preparations were plunged in ACSF for 30 min at 37 °C prior to the experimental protocol was carried out. One trigeminal ganglion was immersed in 220 μL ACSF in the caps of reaction tubes and incubated at 37 °C for two periods of 5 min. In the first incubation period, the preparation was incubated in the control solution consisting ACSF with 0.1% ethanol, to determine the basal release of CGRP or SP as a control for each group. During the second period, the preparations were incubated with the test substances (1 μM 17β-estradiol for estrogen group, 1 μM progesterone for progesterone group, and 17β-estradiol + progesterone for estrogen + progesterone group). After each incubation period, the incubation fluid containing the released substances (CGRP and SP) was collected from the caps without touching the ganglia, and a sample volume of 200 μL was mixed with protease inhibitor cocktail and stored at − 80 °C.
Determination of CGRP and substance P contents in plasma, meningeal and trigeminal ganglia superfusates
CGRP and SP contents in the samples including plasma, meningeal and trigeminal ganglia superfusates were measured by an enzyme-linked immunosorbent assay kit as described previously (Kilinc et al. 2020a). The sensitivity was 1.02 pg/mL, and range: 2–600 pg/mL for CGRP. The sensitivity was 2.49 pg/mL, and range: 5–1000 pg/mL for SP. The protocol was carried out according to the manufacturer’s instructions and performed as duplicates. Shortly, 50 μL of CGRP or SP standard was added to standard wells. 40 μL of the samples and then 10 μL anti-CGRP or anti-SP antibody was added to sample wells. 50 μL streptavidin-HRP was added to standard wells and sample wells. Then, the 96-well plates were incubated at 37 °C for 60 min; later on 50 μL substrate solution A and B were added to each well respectively, and the plate was incubated at 37 °C for 10 min. Immediately after the incubation, 50 μL of stop solution was added to each well. The optical density was measured at 450 nm using a microplate reader (Epoch BioTek Instruments, Inc. Highland Park, Winooski, VT, USA). Optical density curve was plotted by using standards with defined CGRP and SP concentrations.
Statistical analysis
The data obtained from all experimental groups were expressed as mean ± standard error of mean. Statistical analysis was performed using SPSS for Windows (version 17.0, SPSS Inc., Chicago, IL, USA). Shapiro–Wilk test was used for testing the normality of data. All data obtained from in-vivo and ex-vivo experiments exhibit a normal distribution. Therefore, data obtained from in-vivo multiple groups were compared by one-way analysis of variance (ANOVA) followed by LSD multiple comparison test. Data obtained from unpaired samples of ketamine applied controls and CO2 inhaled controls were compared by independent sample’s t-test. Data obtained from paired samples in the ex-vivo experiments were compared by paired t-test. P < 0.05 was accepted as statistically significant.
Results
Effects of estrogen and progesterone on the in-vivo plasma CGRP and SP levels
Intact male rats
17β-Estradiol treatment decreased plasma CGRP levels (P = 0.019, Fig. 3a), but it did not change plasma SP levels (P = 0.89, Fig. 3d) in intact male rats compared to their controls. Moreover, progesterone did not change plasma CGRP (P = 0.102, Fig. 3a) and SP (P = 0.71, Fig. 3d) levels in intact male rats compared to their controls. On the other hand, the combination of 17β-estradiol and progesterone decreased plasma CGRP (P = 0.001, Fig. 3a) and SP levels (P = 0.006, Fig. 3d) in intact male rats compared to their controls, respectively.
Effects of estrogen and progesterone on the plasma basal CGRP and SP levels in the in-vivo groups. Effects of estrogen, progesterone and their combination on the plasma basal CGRP levels a in male rats, b in female rats, and c in ovariectomized rats. Effects of estrogen, progesterone and their combination on the plasma basal SP levels d in male rats, e in female rats, and f in ovariectomized rats. n = 7 rats for each group. Control groups were treated with 10% ethanol in saline as a vehicle in all groups. CGRP: calcitonin gene-related peptide, SP: substance P, Estro: 17β-estradiol, Progest: progesterone, Est + Prog: 17β-estradiol plus progesterone. n.s.: non-significance, *P < 0.05, **P < 0.01, and ***P < 0.001
Intact female rats
17β-estradiol treatment did not change plasma CGRP (P = 0.874, Fig. 3b) and SP (P = 0.742, Fig. 3e) levels in intact female rats compared to their controls, respectively. In addition, progesterone treatment increased plasma concentrations of both CGRP (P = 0.0001, Fig. 3b) and SP (P = 0.0001, Fig. 3e) in intact female rats compared to their controls, respectively. On the other side, the combination of 17β-estradiol and progesterone decreased progesterone-induced plasma CGRP levels (P = 0.0001, Fig. 3b), but not SP levels (P = 0.272, Fig. 3e), in intact female rats, compared to their controls, respectively.
Ovariectomized rats
While 17β-estradiol treatment did not change plasma CGRP levels (P = 0.67, Fig. 3c), it decreased plasma SP levels (P = 0.001, Fig. 3f) in ovariectomized rats, compared to their controls, respectively. Moreover, progesterone treatment did not change plasma CGRP (P = 0.82, Fig. 3c) and SP (P = 0.38, Fig. 3f) levels in ovariectomized rats compared to their controls, respectively. On the one hand, the combination of 17β-estradiol and progesterone did not change plasma CGRP levels (P = 0.13, Fig. 3c) but it reduced SP levels (P = 0.01, Fig. 3f) in ovariectomized rats compared to their controls, respectively.
Effects of estrogen and progesterone on CGRP and SP releases in the ex-vivo preparations prepared from male rats
In hemiskull preparations, while progesterone treatment did not change CGRP release (P = 0.61, Fig. 4a), it reduced SP release (P = 0.04, Fig. 4c) from the peripheral terminals of meningeal trigeminal nerve compared to their controls, respectively. However, none of the 17β-estradiol (P = 0.56 for CGRP, Fig. 4a, P = 0.29 for SP, Fig. 4c) and the combination of 17β-estradiol and progesterone (P = 0.83 for CGRP, Fig. 4a, P = 0.52 for SP, Fig. 4c) changed CGRP or SP release from the peripheral terminals of meningeal trigeminal nerve compared to their controls, respectively.
Effects of estrogen and progesterone on the basal CGRP and SP release from isolated trigeminal ganglia and hemiskull preparations obtained from male rats. Effects of estrogen, progesterone and their combination on the basal CGRP release from (a) the peripheral terminals of the meningeal trigeminal nerve, (b) trigeminal ganglia in the ex-vivo preparations. Effects of estrogen, progesterone and their combination on the basal SP release from (c) the peripheral terminals of meningeal trigeminal nerve, (d) trigeminal ganglia in the ex-vivo preparations. n = 6 hemiskulls or trigeminal ganglions for each group. Control groups were treated with 0.1% ethanol in artificial cerebrospinal-fluid as a vehicle in all groups. CGRP calcitonin gene-related peptide, Estrogen 17β-estradiol, Progest progesterone, Est + Prog 17β-estradiol plus progesterone, ns non-significance, *p < 0.05
In isolated trigeminal ganglia preparations, 17β-estradiol treatment reduced CGRP release (P = 0.046, Fig. 4b), on the contrary, progesterone treatment increased CGRP release (P = 0.027, Fig. 4b) from the trigeminal ganglion neurons compared to their controls, respectively. Moreover, the combination of 17β-estradiol and progesterone did not change CGRP release (P = 0.31, Fig. 4b) from the trigeminal ganglion neurons compared to their control. However, none of the 17β-estradiol, progesterone and their combination changed SP release (P = 0.85, P = 0.52 and P = 0.71 respectively, Fig. 4d) from the trigeminal ganglion neurons compared to their controls, respectively.
Effects of estrogen and progesterone on CGRP and SP releases in the ex-vivo preparations prepared from female rats
In hemiskull preparations, any of the progesterone, 17β-estradiol or their combination did not change CGRP release from the peripheral terminals of meningeal trigeminal nerve compared to their controls, respectively (P = 0.433, P = 0.523 and P = 0.746, Fig. 5a). On the other hand, while progesterone decreased SP release from the peripheral terminals of the meningeal trigeminal nerve (P = 0.001, Fig. 5c), 17β-estradiol or their combination did not change it compared to their controls, respectively (P = 0.165, P = 0.142, Fig. 5c).
Effects of estrogen and progesterone on the basal CGRP and SP releases from isolated trigeminal ganglia and hemiskull preparations obtained from female rats. Effects of estrogen, progesterone and their combination on the basal CGRP release from (a) the peripheral terminals of the meningeal trigeminal nerve, (b) trigeminal ganglia in the ex-vivo preparations. Effects of estrogen, progesterone and their combination on the basal SP release from (c) the peripheral terminals of the meningeal trigeminal nerve, (d) trigeminal ganglia in the ex-vivo preparations. n = 8 hemiskulls or trigeminal ganglions for each group. Control groups were treated with 0.1% ethanol in artificial cerebrospinal-fluid as a vehicle in all groups. CGRP calcitonin gene-related peptide, Estrogen 17β-estradiol, Progest progesterone, Est + Prog 17β-estradiol plus progesterone, ns non-significance, *P < 0.05 and **P < 0.01
In isolated trigeminal ganglia preparations, while progesterone treatment increased CGRP release (P = 0.003), 17β-estradiol or their combination did not change CGRP release from the trigeminal ganglion neurons compared to their controls, respectively (P = 0.935, P = 0.087, Fig. 5b). However, while 17β-estradiol decreased SP release (P = 0.034, Fig. 5d), progesterone increased SP release (P = 0.036 Fig. 5d) from the trigeminal ganglion neurons compared to their controls, respectively. However, the combination of 17β-estradiol and progesterone did not change SP release compared to their control (P = 0.31, Fig. 5d). A schematic illustration of the effects of estrogen, progesterone and their combination on the basal CGRP and SP release in the plasma and structures associated with migraine pain is shown in Fig. 6.
Schematic illustration of effects of estrogen, progesterone and their combination on the basal CGRP and SP release in the plasma and structures associated with migraine pain. In male rats, systemic administration of estrogen decreased plasma basal CGRP levels. Moreover, a combination of estrogen and progesterone reduced both plasma basal CGRP and SP levels. In female rats, while systemic administration of progesterone increased both plasma basal CGRP and SP levels, their co-administration increasesd SP levels without affecting the CGRP levels. In ovariectomized rats, systemic administration of estrogen or their co-administration decreased plasma basal SP levels without changing the CGRP levels. In the ex-vivo preparations obtained from male rats, while topical administration of estrogen diminished basal CGRP release from trigeminal neurons that were the origin site of both CGRP and SP expression, progesterone increased their release. Interestingly estrogen, progesterone or their combination did not affect basal SP release. In addition, in the peripheral terminals of the meningeal trigeminal nerve, progesterone decreased basal SP release without changing the CGRP levels. Moreover, estrogen, progesterone or their combination did not affect both basal CGRP and SP release. In the ex-vivo preparations obtained from female rats, while topical administration of estrogen decreased basal SP release without changing the CGRP release from trigeminal neurons, progesterone increased both basal CGRP and SP release. Moreover, in the peripheral terminals of the meningeal trigeminal nerve, while progesterone decreased basal SP release, any of the other administrations did not change basal CGRP or SP release. On the other hand, their co-administration did not change basal CGRP or SP release from both trigeminal neurons and the peripheral terminals of the meningeal trigeminal nerve. e 17β-estradiol, p progesterone, e + p 17β-estradiol plus progesterone, CGRP calcitonin gene-related peptide, SP substance P
Discussion
Major findings of the in vivo experiments of the current study were that estrogen decreased CGRP release in the male rats without changing it in both intact female and ovariectomized rats. In addition, progesterone increased CGRP and SP release in the females, whereas following ovariectomy the concentration of these peptides returned to similar levels as those in the males. This clearly indicates a critical role of the estrous cycle. Progesterone increased both CGRP and SP release in female rats, whereas estrogen may have a reducing effect. The lack of the effect of exogenous estrogen could be due to the fact that endogenous estrogen is already quite high in proestrus stage and peptide levels can not be further lowered. On the other hand, in the ex-vivo experiments, estrogen decreased CGRP release in the males and SP release in the females in the isolated trigeminal ganglia. Moreover, progesterone administration caused an increase of CGRP and SP in the isolated ganglia of females (and, less pronounced, for CGRP in males), again indicating an effect of endogenous hormones, particularly in the females.
Neuropeptides, predominantly CGRP and SP, released from axons of primary afferent nociceptive neurons of trigeminal nerve via an axon reflex are important contributors in the triggering neurogenic inflammation (Ramachandran 2018; Kilinc et al. 2017b; Dagistan et al. 2019). CGRP and SP released from primary afferents could, in turn, induce plasma protein extravasation, vasodilatation, and activation of meningeal mast cells, consequently all of these events leads to the neurogenic inflammation underlying migraine pain (Koyuncu Irmak et al. 2019; Levy et al. 2019; Kilinc et al. 2020b; Kilinc and Gunes 2019). Estrogen and progesterone receptors are located in components of trigeminovascular system (Giuffre et al. 1992; Gupta et al. 2011; Zuloaga et al. 2012; Labastida-Ramírez et al. 2019), suggesting that fluctuations in the levels of these hormones may regulate activation and sensitization of trigeminovascular system by modulating expression and release of neuro-inflammatory neuropeptides associated with migraine pain such as CGRP and SP.
In the current study, estrogen did not change the basal CGRP levels in the plasma in both intact female and ovariectomized rats. There are very limited and controversial data in the literature regarding the association between estrogen and CGRP levels. In one study, it was shown that CGRP concentrations were increased in post-menopausal women once estrogen levels started to diminish (Wyon et al. 1998). Conversely, in an experimental study, it was demonstrated that estrogen increased CGRP contents in the dorsal root ganglion in ovariectomized rats (Sarajari and Oblinger 2010). Thus, further studies are needed to clarify this dilemma. On the other hand, in the present study, estrogen reduced basal plasma CGRP levels in intact male rats. Moreover, estrogen also decreased basal CGRP release in male isolated trigeminal ganglia, but not in female using the ex-vivo experimental model. These findings are compatible with previous studies, where most of them focused on CGRP contents in the tissues associated with migraine pain. In one of these studies, it was reported that ovariectomy (lack of female sex hormones) increased CGRP concentrations in the dorsolateral periaqueductal gray of rats that has an important role in regulating migraine pain (Wang et al. 2014). Our findings are also compatible with the studies demonstrating an increased gene expression of CGRP following ovariectomy in trigeminal ganglion (Aggarwal et al. 2012), and reduced CGRP levels following estrogen treatment in dorsal root ganglia (Yang et al. 1998) in rats. However, in the present study, topical administration of estrogen did not change basal CGRP release from the peripheral terminals of the meningeal trigeminal nerve in both male and female isolated hemiskull preparations. Previous studies suggested that estrogen shows different effects in the nervous system since estrogen receptors have distinct distribution patterns in the nervous system (Bolay et al. 2011; Hazell et al. 2009; Amandusson et al. 2010; Bereiter et al. 2005). One of these studies demonstrated that estrogen receptor alpha expression was higher in proestrous and diestrous females than male rats (Bereiter et al. 2005). Therefore, based on the results of the present study, we can speculate that such distinct distribution patterns of estrogen receptors may be responsible for different effects of estrogen on the CGRP levels at least between different genders.
In the current study, in addition to CGRP, estrogen did not change basal plasma SP levels in intact male and female rats, while decreased the SP levels in ovariectomized rats. Moreover, in the isolated preparations of males, estrogen did not also change basal SP releases from both meningeal trigeminal nerve terminals and trigeminal ganglia. A previous study reported that estrogen treatment decreased SP contents in the dorsal root ganglion in ovariectomized rats (Sarajari and Oblinger 2010). Therefore, our plasma SP findings in ovariectomized rats are comparable with the results of that study. On the other hand, in the female isolated preparations, while estrogen decreased basal SP release from trigeminal ganglia, it did not change its release from meningeal trigeminal nerve terminals. This finding suggests that estrogen may exhibit different effects on the SP releases in discrete structures including trigeminal ganglia and meningeal trigeminal nerve terminals.
Most of the studies about the effects of sex hormones in structures associated with migraine pain have centered upon effects of estrogen, however, little is known about potential effects of progesterone on central or peripheral trigeminal neurons. In the current study, we found that progesterone increased both plasma basal CGRP and SP levels in intact female rats, while it did not affect them in both male and ovariectomized rats. Moreover, in the male isolated preparations, progesterone decreased basal SP release from peripheral terminals of the trigeminal nerve. In the female isolated preparations, while progesterone increased both basal CGRP and SP release from trigeminal ganglia, it decreased basal SP release from peripheral terminals of the trigeminal nerve. Therefore, our findings suggest that progesterone has dual effects on releases of the neuropeptides in different structures. In terms of such dual effects of progesterone on releases of the neuropeptides, our findings are comparable with previous studies reporting that progesterone reduced CGRP content in the trigeminal nucleus (Moussaoul et al. 1996), while it raised CGRP in the dorsal root ganglion and in plasma (Gangula et al. 2009). Taken all together, our ex-vivo results demonstrated that estrogen exhibited the same effects in peripheral terminals of meningeal trigeminal nerve on both the CGRP (unchanged) and SP (unchanged) release, respectively, in both sexes. However, it shows different effects of trigeminal ganglia in different sexes. In addition, it was also seen that progesterone exhibited the same effects in peripheral terminals of the meningeal trigeminal nerve on the CGRP (unchanged) and SP (decreased) release, respectively, in both sexes. Likewise, similar effects were observed in trigeminal ganglia on both the CGRP (increased) and SP (increased) release, respectively, in both sexes.
On the other hand, based on the results of the in-vivo experiments, it was observed that both estrogen and progesterone show different effects on both the plasma CGRP and SP levels in male, female and ovariectomized rats, respectively. Thus, different effects of sex hormones on the neuropeptides, particularly in intact females and ovariectomized rats, suggested that the estrous cycle might be responsible for this difference. Moreover, our findings support the hypothesis that estrogens and progesterons modulate CGRP and SP release, which could contribute to the prevalence of migraine in women compared to men. CGRP and SP levels could fluctuate regularly in the blood throughout the estrus cycle. Hence, we can speculate that higher CGRP and SP levels may appear in the blood during diestrus/metestrus compared to the proestrus/estrus. Therefore, it is important to determine the regular fluctuations of CGRP and SP in the blood during the estrus cycle. In the present study, female rats were included only at the proestrus phase of the estrous cycle. In the future, it may be beneficial to perform experiments including the females at the other phases of the cycle.
Moreover, our ex-vivo findings also support our hypotheses, such a difference was not observed in our ex-vivo preparations that were excised from the body, most likely because these structures were not linked to the ovaries. Although there are inconsistent results in the literature (Puri et al. 2005; Moussaoul et al. 1996; Aggarwal et al. 2012; Ibrahimi et al. 2017), such a difference may be caused by the different stages of the estrous cycle. Since, in the present study, all female rats were at the same phase (proestrus) of the estrous cycle, and we did not study the other phases such as estrus, metestrus and diestrus stage. However, further studies including all phases of the estrous cycle are required to verify this difference. One of the most possible explanations for this difference between in the in-vivo and ex-vivo experiments, as well as for the difference between intact female and ovariectomized rats in the in-vivo experiments could be caused by the different effects of endogenous and exogenous female sex hormones. In addition, the difference between the in-vivo and ex-vivo experiments could also be caused by the systemic administration of female sex hormones that may act by modulating other neuro-endocrine cascades, such as hypothalamic functions (Cupini et al. 2020). Because our ex-vivo preparations did not contain any neuro/endocrine structure, except for trigeminal ganglion or its meningeal peripheral terminals. Therefore, we can speculate that the hypothalamus may modulate the effects of female sex hormones through CGRP and SP release in migraine. In addition, a combination of estrogen and progesterone reduced both plasma basal CGRP and SP levels in male rats. Moreover, their co-administration decreased progesterone-induced CGRP levels in intact female rats without changing progesterone-induced SP levels. Moreover, in ovariectomized rats, their co-administration decreased plasma basal SP levels without changing CGRP levels. According to our findings, abundant sex hormones in females with intact ovaries could be resulted in the desensitization of their receptors. Because in male and partially in ovariectomized rats, their co-administration reduced plasma CGRP and SP levels. The results of the present study were also consistent with the results of a previous fundamental study reporting that plasma levels of CGRP were higher in women than in men in healthy subjects (Valdemarsson et al. 1990). According to the in-vivo and ex-vivo results of the current study, estrogen seems to antagonize the inducing effects of progesterone on the CGRP and SP releases, and consequently the combination of estrogen and progesterone seems to stabilize basal CGRP and SP releases from pain-related principal structures, such as peripheral terminals and cell bodies of trigeminal neurons. These findings are also consistent with the menstrual migraine that is triggered by menstruation in women migraine patients. It is considered that estrogen withdrawal during the menstrual cycle could be responsible for menstrual migraine (Somerville 1972; Cupini et al. 2020). It was suggested that a falling in estrogen levels might lead to an increased susceptibility to prostaglandins, which were able to cause neurogenic inflammation through inducing the release of neuropeptides including CGRP and SP (Cupini et al. 2020). It is of capital importance to decrease the stimulated CGRP and SP release in both the menstrual and the other types of migraine since these neuropeptides contribute to the neurogenic inflammation underlying migraine. In the present study, estrogen alleviated progesterone-induced plasma CGRP levels in intact female rats, and reduced plasma SP levels in ovariectomized rats. In addition, it also suppressed stimulatory effects of progesterone on the CGRP and SP releases in trigeminal ganglia in intact female rats. Therefore, our findings confirm the inhibitory effects of estrogen on the CGRP and SP release.
Conclusion
In conclusion, our findings suggested that estrogen exhibited protective effects against neurogenic inflammation underlying pathophysiology of migraine through modulating CGRP and SP release in both sexes. Progesterone demonstrated dual effects on the neurogenic inflammation by inducing or inhibiting CGRP and SP release in females.
Limitations of the study
In the present study, we chose all female rats at the same phase (proestrus) of the estrous cycle, and did not study the other phases such as estrus, metestrus and diestrus stage. In addition to the proestrus stage of the estrous cycle, if the other phases were also examined, the impact of each phase of the estrus cycle on these neuropeptides would be investigated more comprehensively. Moreover, in the present study, we focused on predominant markers of neurogenic inflammation underlying migraine such as CGRP and SP. However, it would be favorable if the other markers associated with neurogenic inflammation were also studied, including neurokinin A, bradykinin and nitric oxide.
References
Aggarwal M, Puri V, Puri S (2012) Effects of estrogen on the serotonergic system and calcitonin gene-related peptide in trigeminal ganglia of rats. Ann Neurosci 19:151–157
Amandusson A, Blomqvist A (2010) Estrogen receptor-alpha expression in nociceptive-responsive neurons in the medullary dorsal horn of the female rat. Eur J Pain 14:245–248
Andrabi SS, Parvez S, Tabassum H (2017) Progesterone induces neuroprotection following reperfusion-promoted mitochondrial dysfunction after focal cerebral ischemia in rats. Dis Model Mech 10:787–796
Ashina M, Bendtsen L, Jensen R, Schifter S, Olesen J (2000) Evidence for increased plasma levels of calcitonin gene-related peptide in migraine outside of attacks. Pain 86:133–138
Avona A, Burgos-Vega C, Burton MD, Akopian AN, Price TJ, Dussor G (2019) Dural calcitonin gene-related peptide produces female-specific responses in rodent migraine models. J Neurosci 39:4323–4331
Bereiter DA, Cioffi JL, Bereiter DF (2005) Oestrogen receptor-immunoreactive neurons in the trigeminal sensory system of male and cycling female rats. Arch Oral Biol 50:971–979
Bolay H, Berman NE, Akcali D (2011) Sex-related differences in animal models of migraine headache. Headache 51:891–904
Cupini LM, Corbelli I, Sarchelli P (2020) Menstrual migraine: what it is and does it matter? J Neurol. https://doi.org/10.1007/s00415-020-09726-2
Dagistan Y, Kilinc E, Nur Balci C (2019) Cervical sympathectomy modulates the neurogenic inflammatory neuropeptides following experimental subarachnoid hemorrhage in rats. Brain Res 146366
Eberhardt M, Hoffmann T, Sauer SK, Messlinger K, Reeh PW, Fischer MJ (2008) Calcitonin gene-related peptide release from intact isolated dorsal root and trigeminal ganglia. Neuropeptides 42:311–317
Ebersberger A, Averbeck B, Messlinger K, Reeh PW (1999) Release of substance P, calcitonin gene-related peptide and prostaglandin E2 from rat dura mater encephali following electrical and chemical stimulation in vitro. Neuroscience 89:901–907
Fatehi M, Fatehi-Hassanabad Z (2008) Effects of 17beta-estradiol on neuronal cell excitability and neurotransmission in the suprachiasmatic nucleus of rat. Neuropsychopharmacology 33:1354–1364
Frederiksen SD, Bekker-Nielsen Dunbar M, Snoer AH, Deen M, Edvinsson L (2020) Serotonin and neuropeptides in blood from episodic and chronic migraine and cluster headache patients in case-control and case-crossover settings: a systematic review and meta-analysis. Headache 60:1132–1164
Fusayasu E, Kowa H, Takeshima T, Nakaso K, Nakashima K (2007) Increased plasma substance P and CGRP levels, and high ACE activity in migraineurs during headache-free periods. Pain 128:209–214
Gangula PR, Chauhan M, Reed L, Yallampalli C (2009) Age-related changes in dorsal root ganglia, circulating and vascular calcitonin gene-related peptide (CGRP) concentrations in female rats: effect of female sex steroid hormones. Neurosci Lett 454:118–123
Giuffrè R, Palma E, Liccardo G, Sciarra F, Pastore FS, Concolino G (1992) Sex steroid hormones in the pathogenesis of chronic subdural haematoma. Neurochirurgia 35:103–107
Goadsby PJ, Edvinsson L, Ekman R (1990) Vasoactive peptide release in the extracerebral circulation of humans during migraine headache. Ann Neurol 28:183–187
Gupta S, McCarson KE, Welch KM, Berman NE (2011) Mechanisms of pain modulation by sex hormones in migraine. Headache 51:905–922
Hazell GG, Yao ST, Roper JA, Prossnitz ER, O’Carroll AM, Lolait SJ (2009) Localisation of GPR30, a novel G protein-coupled oestrogen receptor, suggests multiple functions in rodent brain and peripheral tissues. J Endocrinol 202:223–236
Ibrahimi K, Danser AHJ, Villalón CM, van den Meiracker AH, MaassenVanDenBrink A (2013) Influence of varying estrogen levels on trigeminal CGRP release in healthy women. J Headache Pain 14:P123
Ibrahimi K, Vermeersch S, Frederiks P, Geldhof V, Draulans C, Buntinx L, Lesaffre E, MaassenVanDenBrink A, de Hoon J (2017) The influence of migraine and female hormones on capsaicin-induced dermal blood flow. Cephalalgia 37:1164–1172
Kilinc E, Gunes H (2019) Modulatory effects of neuropeptides on pentylenetetrazol-induced epileptic seizures and neuroinflammation in rats. REV ASSOC MED BRAS 65:1188–1192
Kilinc E, Dagistan Y, Kotan B, Cetinkaya A (2017a) Effects of Nigella sativa seeds and certain species of fungi extracts on number and activation of dural mast cells in rats. Physiol Int 104:15–24
Kilinc E, Guerrero-Toro C, Zakharov A, Vitale C, Gubert-Olive M, Koroleva K, Timonina A, Luz LL, Shelukhina I, Giniatullina R, Tore F, Safronov BV, Giniatullin R (2017b) Serotonergic mechanisms of trigeminal meningeal nociception: implications for migraine pain. Neuropharmacology 116:160–173
Kilinc E, Dagistan Y, Kukner A, Yilmaz B, Agus S, Soyler G, Tore F (2018) Salmon calcitonin ameliorates migraine pain through modulation of CGRP release and dural mast cell degranulation in rats. Clin Exp Pharmacol Physiol 45:536–546
Kilinc E, Ankarali S, Torun IE, Dagistan Y (2020a) Receptor mechanisms mediating the anti-neuroinflammatory effects of endocannabinoid system modulation in a rat model of migraine. Eur J Neurosci. https://doi.org/10.1111/ejn.14897
Kilinc E, Tore F, Dagistan Y, Bugdayci G (2020b) Thymoquinone inhibits neurogenic inflammation underlying migraine through modulation of calcitonin gene-related peptide release and stabilization of meningeal mast cells in glyceryltrinitrate-induced migraine model in rats. Inflammation 43:264–273
Koyuncu Irmak D, Kilinc E, Tore F (2019) Shared fate of meningeal mast cells and sensory neurons in migraine. Front Cell Neurosci 13:136
Labastida-Ramírez A, Rubio-Beltrán E, Villalón CM, MaassenVanDenBrink A (2019) Gender aspects of CGRP in migraine. Cephalalgia 39:435–444
Levy D, Labastida-Ramirez A, MaassenVanDenBrink A (2019) Current understanding of meningeal and cerebral vascular function underlying migraine headache. Cephalalgia 39:1606–1622
Li XZ, Sui CY, Chen Q, Zhuang YS, Zhang H, Zhou XP (2016) The effects and mechanism of estrogen on rats with Parkinson’s disease in different age groups. Am J Transl Res 8:4134–4146
Masuyama H, Hiramatsu Y (2011) Potential role of estradiol and progesterone in insulin resistance through constitutive androstane receptor. J Mol Endocrinol 47:229–239
Moussaoul S, Duval P, Lenoir V, Garret C, Kerdelhue B (1996) CGRP in the trigeminal nucleus, spinal cord and hypothalamus: effect of gonadal steroids. Neuropeptides 30:546–550
Pavlović JM, Allshouse AA, Santoro NF, Crawford SL, Thurston RC, Neal-Perry GS, Lipton RB, Derby CA (2016) Sex hormones in women with and without migraine: evidence of migraine-specific hormone profiles. Neurology 87:49–56
Puri V, Cui L, Liverman CS, Roby KF, Klein RM, Welch KM, Berman NE (2005) Ovarian steroids regulate neuropeptides in the trigeminal ganglion. Neuropeptides 39:409–417
Puri V, Puri S, Svojanovsky SR, Mathur S, Macgregor RR, Klein RM, Welch KM, Berman NE (2006) Effects of oestrogen on trigeminal ganglia in culture: implications for hormonal effects on migraine. Cephalalgia 26:33–42
Ramachandran R (2018) Neurogenic inflammation and its role in migraine. Semin Immunopathol 40:301–314
Sarajari S, Oblinger MM (2010) Estrogen effects on pain sensitivity and neuropeptide expression in rat sensory neurons. Exp Neurol 224:163–169
Somerville BW (1972) The role of estradiol withdrawal in the etiology of menstrual migraine. Neurology 22:355–365
Valdemarsson S, Edvinsson L, Hedner P, Ekman R (1990) Hormonal influence on calcitonin gene-related peptide in man: effects of sex difference and contraceptive pills. Scand J Clin Lab Invest 50:385–388
van Dongen RM, Zielman R, Noga M, Dekkers OM, Hankemeier T, van den Maagdenberg AM, Terwindt GM, Ferrari MD (2017) Migraine biomarkers in cerebrospinal fluid: a systematic review and meta-analysis. Cephalalgia 37:49–63
Vetvik KG, MacGregor EA (2017) Sex differences in the epidemiology, clinical features, and pathophysiology of migraine. Lancet Neurol 16:76–87
Wang D, Zhao J, Wang J, Li J, Yu S, Guo X (2014) Deficiency of female sex hormones augments PGE2 and CGRP levels within midbrain periaqueductal gray. J Neurol Sci 346:107–111
Wyon Y, Frisk J, Lundeberg T, Theodorsson E, Hammar M (1998) Postmenopausal women with vasomotor symptoms have increased urinary excretion of calcitonin gene-related peptide. Maturitas 30:289–294
Yang Y, Ozawa H, Lu H, Yuri K, Hayashi S, Nihonyanagi K, Kawata M (1998) Immunocytochemical analysis of sex differences in calcitonin gene-related peptide in the rat dorsal root ganglion, with special reference to estrogen and its receptor. Brain Res 791:35–42
Zuloaga KL, O'Connor DT, Handa RJ, Gonzales RJ (2012) Estrogen receptor beta dependent attenuation of cytokine-induced cyclooxygenase-2 by androgens in human brain vascular smooth muscle cells and rat mesenteric arteries. Steroids 77:835–844
Acknowledgements
We declare that there are not any relationships that represent a potential conflict of interest.
Funding
This work was supported by Bolu Abant Izzet Baysal University Scientific Research Fund (Grant number: 2017.08.02.1258).
Author information
Authors and Affiliations
Corresponding author
Additional information
Communicated by Thomas Deller.
Publisher's Note
Springer Nature remains neutral with regard to jurisdictional claims in published maps and institutional affiliations.
Electronic supplementary material
Below is the link to the electronic supplementary material.
221_2020_5923_MOESM1_ESM.pdf
Supplementary Figure 1. The effect of anaesthesia on the plasma CGRP and SP levels. There was no difference between ketamine administered controls and controls with CO2 inhalation in terms of plasma CGRP (A) and SP (B) levels (P>0.05). CGRP: calcitonin gene-related peptide, SP: substance P (PDF 1472 kb)
221_2020_5923_MOESM2_ESM.pdf
Supplementary Figure 2. The four stages of the rat estrous cycle indicating the cytological findings of the vaginal fluid from each stage. (A) Proestrus stage, (B) estrus stage, (C) metestrus stage and (D) diestrus stage. Arrows indicate leukocytes, white arrowheads show nucleated epithelial cells, and black arrowheads indicate cornified epithelial cells (PDF 1701 kb)
Rights and permissions
About this article
Cite this article
Cetinkaya, A., Kilinc, E., Camsari, C. et al. Effects of estrogen and progesterone on the neurogenic inflammatory neuropeptides: implications for gender differences in migraine. Exp Brain Res 238, 2625–2639 (2020). https://doi.org/10.1007/s00221-020-05923-7
Received:
Accepted:
Published:
Issue Date:
DOI: https://doi.org/10.1007/s00221-020-05923-7