Abstract
Purpose
Survival outcomes in children with heart disease after use of either non-emergent extracorporeal membrane oxygenation (ECMO) or cardiopulmonary resuscitation (CPR) onto ECMO (ECPR) are comparable. Concerns remain regarding the impact of CPR duration on survival and neurological outcome.
Methods
Children with cardiac disease requiring ECMO were identified from our database. Demographic, operative and ECMO details were evaluated with respect to survival. In addition, resuscitation details were extracted for the recent subgroup requiring ECPR; these details were evaluated with respect to survival and neurological outcomes at midterm follow-up by univariate analysis and multivariable logistic regression.
Results
There were 126 ECMO runs in 116 children; 61 (53%) received ECPR. Forty-eight (41%) children survived to discharge; survival in the most recent era was 48%. Thirty-seven children underwent ECPR in the most recent era with 14 (38%) surviving to discharge. Duration of cardiopulmonary resuscitation differed significantly between survivors and nonsurvivors (15 vs. 40 min, p = 0.009); children requiring ≥30 min of CPR had 79% reduced odds of hospital survival (OR = 0.21, 95% CI = 0.05–0.96, p = 0.04). Two children died after hospital discharge; with 33% having paediatric cerebral performance category scores ≤2. Poor neurological outcome was associated with longer duration of CPR (32 vs. 17.5 min, p = 0.03).
Conclusions
Despite comparable survival outcomes between ECPR and non-emergent ECMO in children with cardiac disease a significant association between CPR duration and outcome (survival and neurological) was noted in this population.
Similar content being viewed by others
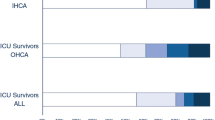
Explore related subjects
Discover the latest articles, news and stories from top researchers in related subjects.Avoid common mistakes on your manuscript.
Introduction
Children after in-hospital cardiac arrest continue to have significant morbidity and mortality [1]. Survival to hospital discharge after in-hospital paediatric cardiac arrest is reported to be between 13 and 27% [1–8] with conventional resuscitation. A large study of cardiac arrest involving 880 children from 156 institutions demonstrated a 27% survival to discharge [1]. Requiring more than 15 min of cardiopulmonary resuscitation (CPR) has been associated with poor outcome [3, 8]. Numerous studies have documented successful use of extracorporeal membrane oxygenation (ECMO) during CPR (ECPR) [9, 10] in children with cardiac disease [11, 12]. Reported survival to discharge with ECPR ranges from 25 to 50% [10, 12–14]; a recent study examining 695 ECPR episodes from the Extracorporeal Life Support Organization (ELSO) registry shows a 38% survival to discharge [12]. Despite laudable ECPR survival outcomes in children, the potential burden of neurological morbidity is cause for concern [15, 16] and is likely to be under-reported in registry data [15].
We reviewed the outcomes of all children with cardiac disease who required veno-arterial (VA) ECMO support at our institution. Our rapid response ECMO program began in 2002 and since then our use of ECPR has increased in this population. Recognizing that these children with cardiac disease may have the poorest neurologic outcomes, we sought to describe these outcomes with attention to predictive factors.
Methods
This study was approved by the Royal Children’s Hospital (RCH) Ethics and Research Committee. We performed a retrospective cohort study of all children with cardiac disease who required ECMO from November 1990 to April 2006. We evaluated potential predictive factors with respect to the primary outcome of interest—survival to hospital discharge.
RCH is a tertiary paediatric hospital with 250 inpatient beds, 20 paediatric intensive care unit (PICU) beds and a cardiac surgical program that performs 400–500 open surgical cases a year. RCH has a mechanical support program performing 20–30 ECMO runs/year. All RCH patients who require ECMO are managed in the PICU. Potential patients were identified through a review of the institutional ECMO database which was created in 1997 and has since been prospectively maintained. Data on ECMO runs prior to 1997 were gathered through an extensive chart review at database inception. We excluded all patients placed on veno-venous ECMO, all patients placed on ECMO for primary respiratory or septic indications and all patients with congenital diaphragmatic hernia. For patients requiring multiple ECMO episodes during a single hospital admission, only the initial ECMO episode was analysed with respect to the outcomes of interest. Patients were assigned a risk score for comparison using the risk adjustment for congenital heart surgery 1 (RACHS-1) score [17].
Children were placed on VA ECMO for either non-emergent or emergent indications. Non-emergent ECMO indications were predominantly due to failure to separate from cardiopulmonary bypass in the operating room. Other urgent (but non-emergent) indications for cannulation in the PICU included progressive low cardiac output states or hypoxaemia related to shunt obstruction. Emergent indications for ECMO (hereafter called ECPR) consisted of ECMO deployed for refractory cardiac arrest (in our institution, either 3 rounds of resuscitative drugs or maximal duration of 5 min without return of spontaneous circulation). Cardiac arrest was defined as any patient requiring external or internal cardiac massage for 60 s or greater because of a profound low output cardiac state, consistent with the operational definition of cardiac arrest from the American Heart Association National Registry of CPR [18] and the revised Utstein [19] format. Patients denoted as ECPR did not regain return of spontaneous circulation at the terminal portion of the resuscitation, thus requiring continuing CPR for ultimate cannulation on to ECMO. Outcomes were reported as a function of the number of patients (n = 116), not the number of events (n = 126), as recommended by the Utstein reporting template.
Circuit composition, cannulation and ECMO management at RCH Melbourne
RCH uses a compact centrifugal impeller-driven pumphead with minimal priming volumes for rapid deployment. In January 2002, our institution changed the standard ECMO circuit to a Rotaflow (Maquet, Jostra Medizintechnik AG, Hirrlingen, Germany) pumphead and Quadrox D membrane oxygenator (Maquet, Jostra Medizintechnik AG, Hirrlingen, Germany) so that a single system could be utilized for all patients regardless of size or indication. Since this time, one infant circuit (and occasionally a second for patients >15 kg) is always primed and easily accessible to the PICU. These circuits can be quickly mobilized during a cardiac arrest and ECPR initiated by an ECMO rapid response team (RRT) (without the presence of a perfusionist). The circuits are pre-primed with crystalloid (Plasmalyte A; Baxter); surgical equipment and ECMO cannulae are available in an ECMO cart that is always accessible in the PICU. Transthoracic cannulation is preferred in those children who are recently (≤14 days) post cardiotomy; primary neck cannulation is used for all other patients. Haemoconcentration was achieved through pack cell transfusion to target a haematocrit ≥30% and a mixed venous saturation ≥70%; these were continuously monitored through the inflow venous cannula.
ECMO flows are increased to a goal of 100–150 ml/kg/min. Mechanical ventilation is adjusted to “rest” settings when adequate flows are ensured. Sweep gas FiO2 is adjusted to an inspired oxygen concentration of 0.21 and sweep gas flow rates are adjusted to maintain normocapnia. After ECPR, controlled hypothermia is maintained at a core temperature of 33–34°C for 48 h at which point slow rewarming occurs over an additional 24 h to 36–37°C. After an initial bolus of 100 U/kg of heparin at cannulation, heparin infusion is titrated to maintain an activated clotting time (ACT) of 180–220 s. Platelet counts are maintained about 100 × 109/L with transfusions as necessary. Ongoing bleeding is managed with adjustments of heparinization, local control and antifibrinolytics unless surgical re-exploration was deemed necessary.
Decisions regarding further evaluation (echocardiography, cardiac catheterization) and/or reoperation were patient specific and made by the multidisciplinary PICU and cardiac surgery teams. Clinical evaluation and symptomatology dictated the need for neurologic consultation and/or testing. Routine head imaging or continuous electroencephalography (EEG) while on ECMO was not the standard during the study period.
Data collection and statistical analysis
Demographic, pre-ECMO, ECMO and outcomes were compared for survivors and nonsurvivors; descriptive statistics were generated for all variables in the dataset with particular emphasis on survival outcomes. Outcomes for the entire cohort were analysed. Comparisons were made between ECMO patients during two eras: before and after creation of the RRT. Outcomes were also assessed for the recent ECPR subgroup for which complete resuscitation and midterm outcome data were available.
To assess global outcome we utilized the pediatric cerebral performance category (PCPC) score [20]. Scores were assigned prospectively to all survivors during follow-up assessment.
For comparisons of continuous data, t tests or the Wilcoxon rank-sum test were used. Comparisons of categorical data were performed with the χ 2 test; the Fisher’s exact test was substituted when the expected cell counts were ≤5. Predictors of survival to hospital discharge were evaluated by univariate analysis. Candidate variables for inclusion in a multivariable logistic regression model were chosen from the initial univariate analysis with the criteria of selection set at p ≤ 0.1. Model validity was evaluated using the Wald statistic for the overall model. Restricted models with fewer parameters were compared with full model initially using the likelihood and Wald statistics and then more definitively using the likelihood ratio test. The fit of the model was evaluated using the Hosmer–Lemeshow statistic.
SAS Version 9.2 (Cary, NC, USA) was used for the analysis. Continuous data are reported as median values with interquartile range (IQR) with categorical data reported as frequency (n) and percentage (%). Statistical significance was set at a p value ≤0.05.
Results
Entire cohort
From 1990 to 2006, 116 children with cardiac disease underwent 126 ECMO runs at our institution (Fig. 1). Baseline characteristics of our ECMO inception cohorts were compared before and after initiation of our ECMO rapid response team (RRT) that occurred in 2002. Age at cannulation was 1.7 (0.4, 15.5) months at a weight of 3.9 (3.0, 8.7) kg. Twenty-seven children (23%) had single-ventricle congenital heart disease, 69 (60%) had two-ventricle congenital heart disease and 20 (17%) had primary myocardial disease (14 with myocarditis/cardiomyopathy and 6 post heart transplant). ECPR was used in 61 (52%) of the patients with more frequent usage after creation of the RRT (40.7 vs. 62.9%, p = 0.01). Patients resuscitated after creation of the RRT were younger (0.8 vs. 3 months, p = 0.02) and had a higher RACHS-1 score (4 vs. 3, p = 0.01). Patients requiring ECPR had a higher peak lactate (13 vs. 4.8 mmol/L, p < 0.0001), lower nadir systolic blood pressure (40 vs. 50 mmHg, p = 0.01) and a lower arterial nadir pH (7.18 vs. 7.36, p = 0.001) when compared with non-emergent ECMO patients. Timing of cannulation (during regular hours vs. on-call hours; p = 0.79), arterial blood gas pH prior to cannulation (p = 0.16) and duration of ECMO support did not change significantly throughout the period of study (p = 0.26). The distribution of RACHS-1 scores in our cohort increased significantly to reflect a higher risk category of patients in the most recent era (from a median RACHS 3–4; p = 0.01) (Table 1). This is also reflected by the fact that 20 of the 27 (74%) children with single-ventricle congenital heart disease were supported in the most recent era (since January 2002).
The overall survival to decannulation, PICU discharge and hospital discharge for the entire cohort was 64 of 116 (55%), 48 of 116 (41%) and 48 of 116 (41%) respectively. There were no significant predictors of survival to hospital discharge. The overall survival to PICU and hospital discharge improved after introduction of the RRT from 33.3 to 48.4%, although this did not achieve statistical significance (p = 0.10). After initiation of the RRT, there was significant improvement in survival to 24 h post decannulation (42.6 to 66.1%, p = 0.01), survival to 7 days post decannulation (37.0 vs. 58.1%, p = 0.02) and survival to 28 days post decannulation (31.5 vs. 51.6%, p = 0.03). Baseline risk stratification (RACHS-1 score) was not different between survivors (median 3, IQR 3–4) and nonsurvivors (median 4, IQR 3–4; p = 0.30).
Recent cohort (2002–2006)
In our recent experience, ECPR was used in 37 children with cardiac disease at an age of 22 (8, 150) days, weight of 3.4 (2.8, 4.8) kg, peak lactate of 12.2 (5.5, 14.3) mmol/L, nadir arterial pH of 7.14 (7.00, 7.36) and nadir pre-ECMO systolic blood pressure of 41 (29, 50) mmHg. Twenty patients (54%) were male. The duration of CPR and time to establishment of ECMO flows were 30 (15, 50) and 60 (40, 95) min respectively. The duration of ECMO support, PICU and hospital stay in this population was 4.3 (2.9, 6.9), 9.6 (16, 33) and 11 (23, 46) days respectively; 23 (62%) patients survived for at least 24 h after decannulation with 14 (38%) patients surviving to PICU and hospital discharge (Table 2). Two patients died after hospital discharge at a mean time of 2.5 months post hospital discharge; both had sudden cardiac arrests precipitated by an aspiration event. The remaining 12 patients were reviewed at a post discharge follow-up of 2.1 (1.1, 4.0) years. Four patients had a good neurological outcome as defined by a PCPC of ≤2. Of all risk factors examined only a longer duration of CPR prior to successful ECMO cannulation (Fig. 2) was significantly associated with hospital mortality (40 vs. 15 min, p = 0.009). This model was adjusted for RACHS-1 classification as a confounding variable. This model likelihood ratio and Wald statistic were both statistically significant (χ 2 = 15.8, p = 0.001 and χ 2 = 7.9, p = 0.04). The Hosmer–Lemeshow goodness of fit test was consistent with an acceptable model (chi-squared statistic 10.1, p = 0.38). After adjusting for RACHS-1 score, need for more than 30 min of CPR reduced the odds of hospital survival by 79% (OR = 0.21, 95% CI = 0.05–0.96, p = 0.04). Longer duration of CPR was also associated with a lower likelihood of a good neurological outcome (32 vs. 17.5 min, p = 0.02; OR = 0.6, 95% CI = 0.36–1.05, p = 0.07 per 5-min increment of CPR).
Interventions and complications during ECMO
The frequency and type of complications on ECMO are a function of the circuit technology, patient population [21] and perhaps duration of support [14]. Overall, the incidence of ECMO complications in ECPR survivors and nonsurvivors is shown in Table 3. The incidence of circuit complications, re-exploration for bleeding, blood product utilization and end-organ injury was comparable in both groups. There was a trend towards greater reintervention on ECMO in survivors (10 of 14) compared with nonsurvivors (7 of 23; p = 0.08) driven predominantly by a greater frequency of shunt revisions in survivors (5 vs. 1; p = 0.02). Survivors were more likely to have recovery of cardiac function. Discontinuation of extracorporeal support was more likely to be prompted by poor prognostic factors (lack of cardiovascular recovery, neurologic devastation or multiorgan injury) in the nonsurvivors.
Discussion
The use of ECMO resulted in a 41% survival of children with cardiac disease who may have otherwise died at our institution; this survival rate increased to 48% in our most recent experience. The use of ECPR has increased in our institution with a survival rate in our recent cohort of 38%. In contradistinction to other series [10, 13, 14], we have demonstrated that duration of CPR prior to ECMO may be a risk factor for hospital survival and may potentially affect the quality of neurological outcome.
Survival outcomes after ECMO may be influenced by a number of issues including patient factors (genetic predisposition, cardiac diagnosis), operative factors, decision making while on ECMO and complications related to the ECMO run. Noncardiac structural and chromosomal anomalies increased the odds of ECPR mortality by a factor of 3 in the multivariable model generated by Kane et al. [14]. Technical issues related to shunt and coronary artery anastomoses may predispose certain groups to cardiac arrest [22]. ECPR combined with timely identification and elimination of residual lesions can lead to superior survival and functional outcomes in this patient group [22]. This was demonstrated by our significant association between need for shunt revision after ECPR and survival; this has been reported by other groups [23, 24].
Conventional resuscitative efforts are often limited to 15–20 min based on data showing poor outcome beyond this threshold [1, 3, 8]. Recent findings from a large single institution experience of ECPR demonstrated no difference in CPR duration between survivors and nonsurvivors [14]. These findings and the recognition that successful outcomes with ECPR after long (>60 min) CPR duration can occur [25] have tended to result in liberal use of ECMO in this scenario. Our data demonstrate a survival after 30 min of CPR of less than 25%. These data are sobering and reaffirm the need to identify patients unlikely to benefit from ECPR. At this point however, we believe that families of patients in whom ECPR has been instituted after a long duration (>50–60 min) of CPR should be given a guarded prognosis. In addition, if such patients have persistent metabolic acidosis, slow lactate clearance or development of multiorgan dysfunction during ECMO, then the family should be advised that survival is unlikely and potential morbidity is high.
Because of the organization at our particular institution, the duration from when the ECMO call was initiated to when flows were established was about 60 min; this did not differ between ECPR survivors and nonsurvivors. In addition, ECMO cannulation during regular hours or on-call hours did not affect survival outcome. Our institutional organization likely prompted early calls for the ECMO team prior to cardiac arrest; however it is clear from the discrepancy between the times to cannulation and the duration of CPR that many of these deteriorated to ECPR cannulations.
Our data also demonstrated some late mortality and significant morbidity in the hospital survivors of ECPR. Two survivors died at a mean of 2.5 months after discharge. Both children had primary aspiration events that secondarily led to their terminal cardiac arrest. In addition, 8 of 12 (67%) of our late survivors had PCPC scores ≤3 (with only 4 patients having PCPC scores of ≤2). Good neurological outcome as defined by a PCPC score ≤2 was significantly associated with a lower duration of CPR prior to ECMO. This relationship between duration of CPR and neurological outcome had been demonstrated with conventional resuscitation by de Mos et al. [3]. Huang et al. [26] also showed this relationship with ECPR, however with some important differences in study methodology. Huang assumed that CPR duration was equivalent to the time from activation of ECPR to cannulation; such an assumption may not be valid given that the median cannulation time was more than twice the median CPR duration (65 vs. 30 min, p < 0.0001) in our study. In addition, the median age of 4 years in their study is not generalizable to most paediatric cardiac ECPR programs [26]. The degree of neurological injury in our survivors is different than that reported by Kane et al. [14], where 79% had PCPC scores ≤2. This difference may be explained by their group’s faster deployment by an in-house surgical team, shorter CPR times and higher utilization of ECPR standby during catheterization procedures. The neurological outcomes in our cohort are in keeping with more detailed systematic developmental follow-up reported by Lequier et al. [16] and other groups [15, 27].
Our study has a number of important limitations. Two studies had previously demonstrated a significant association between mortality and complications while on ECMO [12, 14]; specifically neurological and renal complications. Although we found no such association, our low complication rates were likely driven by a small sample size and an absence of systematic screening with head imaging and EEG while on ECMO. In addition, decision making regarding day-to-day management while on ECMO was difficult to capture at the individual patient level; there was not a systematic approach to timing of investigations (echocardiography, catheterization) during the study period.
In conclusion, ECMO plays an important role in the optimal management of critically ill children with heart disease. In the current study, survival outcomes after ECPR were comparable with non-emergent ECMO; this is in keeping with other published data. However, we have noted a significant association between duration of CPR and hospital mortality. Despite a good neurological outcome in 33% of survivors, longer duration of CPR in our population was associated with poor neurological outcome.
References
Nadkarni VM, Larkin GL, Peberdy MA, Carey SM, Kaye W, Mancini ME, Nichol G, Lane-Truitt T, Potts J, Ornato JP, Berg RA (2006) First documented rhythm and clinical outcome from in-hospital cardiac arrest among children and adults. JAMA 295:50–57
Akcay A, Baysal SU, Yavuz T (2006) Factors influencing outcome of inpatient pediatric resuscitation. Turk J Pediatr 48:313–322
de Mos N, van Litsenburg RR, McCrindle B, Bohn DJ, Parshuram CS (2006) Pediatric in-intensive-care-unit cardiac arrest: incidence, survival, and predictive factors. Crit Care Med 34:1209–1215
Donoghue A, Berg RA, Hazinski MF, Praestgaard AH, Roberts K, Nadkarni VM (2009) Cardiopulmonary resuscitation for bradycardia with poor perfusion versus pulseless cardiac arrest. Pediatrics 124:1541–1548
Lopez-Herce J, Garcia C, Dominguez P, Carrillo A, Rodriguez-Nunez A, Calvo C, Delgado MA (2004) Characteristics and outcome of cardiorespiratory arrest in children. Resuscitation 63:311–320
Meert KL, Donaldson A, Nadkarni V, Tieves KS, Schleien CL, Brilli RJ, Clark RS, Shaffner DH, Levy F, Statler K, Dalton HJ, van der Jagt EW, Hackbarth R, Pretzlaff R, Hernan L, Dean JM, Moler FW (2009) Multicenter cohort study of in-hospital pediatric cardiac arrest. Pediatr Crit Care Med 10:544–553
Lopez-Herce J, Garcia C, Rodriguez-Nunez A, Dominguez P, Carrillo A, Calvo C, Delgado MA (2005) Long-term outcome of paediatric cardiorespiratory arrest in Spain. Resuscitation 64:79–85
Slonim AD, Patel KM, Ruttimann UE, Pollack MM (1997) Cardiopulmonary resuscitation in pediatric intensive care units. Crit Care Med 25:1951–1955
Alsoufi B, Al-Radi OO, Nazer RI, Gruenwald C, Foreman C, Williams WG, Coles JG, Caldarone CA, Bohn DG, Van Arsdell GS (2007) Survival outcomes after rescue extracorporeal cardiopulmonary resuscitation in pediatric patients with refractory cardiac arrest. J Thorac Cardiovasc Surg 134:952–959, e952
Morris MC, Wernovsky G, Nadkarni VM (2004) Survival outcomes after extracorporeal cardiopulmonary resuscitation instituted during active chest compressions following refractory in-hospital pediatric cardiac arrest. Pediatr Crit Care Med 5:440–446
Dalton HJ, Siewers RD, Fuhrman BP, Del Nido P, Thompson AE, Shaver MG, Dowhy M (1993) Extracorporeal membrane oxygenation for cardiac rescue in children with severe myocardial dysfunction. Crit Care Med 21:1020–1028
Thiagarajan RR, Laussen PC, Rycus PT, Bartlett RH, Bratton SL (2007) Extracorporeal membrane oxygenation to aid cardiopulmonary resuscitation in infants and children. Circulation 116:1693–1700
del Nido PJ, Dalton HJ, Thompson AE, Siewers RD (1992) Extracorporeal membrane oxygenator rescue in children during cardiac arrest after cardiac surgery. Circulation 86:II300–II304
Kane DA, Thiagarajan RR, Wypij D, Scheurer MA, Fynn-Thompson F, Emani S, del Nido PJ, Betit P, Laussen PC (2010) Rapid-response extracorporeal membrane oxygenation to support cardiopulmonary resuscitation in children with cardiac disease. Circulation 122:S241–S248
Barrett CS, Bratton SL, Salvin JW, Laussen PC, Rycus PT, Thiagarajan RR (2009) Neurological injury after extracorporeal membrane oxygenation use to aid pediatric cardiopulmonary resuscitation. Pediatr Crit Care Med 10:445–451
Lequier L, Joffe AR, Robertson CM, Dinu IA, Wongswadiwat Y, Anton NR, Ross DB, Rebeyka IM (2008) Two-year survival, mental, and motor outcomes after cardiac extracorporeal life support at less than five years of age. J Thorac Cardiovasc Surg 136:976–983 e973
Jenkins KJ, Gauvreau K, Newburger JW, Spray TL, Moller JH, Iezzoni LI (2002) Consensus-based method for risk adjustment for surgery for congenital heart disease. J Thorac Cardiovasc Surg 123:110–118
Reis AG, Nadkarni V, Perondi MB, Grisi S, Berg RA (2002) A prospective investigation into the epidemiology of in-hospital pediatric cardiopulmonary resuscitation using the international Utstein reporting style. Pediatrics 109:200–209
Suominen P, Olkkola KT, Voipio V, Korpela R, Palo R, Rasanen J (2000) Utstein style reporting of in-hospital paediatric cardiopulmonary resuscitation. Resuscitation 45:17–25
Fiser DH, Long N, Roberson PK, Hefley G, Zolten K, Brodie-Fowler M (2000) Relationship of pediatric overall performance category and pediatric cerebral performance category scores at pediatric intensive care unit discharge with outcome measures collected at hospital discharge and 1- and 6-month follow-up assessments. Crit Care Med 28:2616–2620
Sivarajan VB, Best D, Brizard CP, Shekerdemian LS, D’Udekem Y, Horton SB, Butt W (2010) Improved outcomes of paediatric extracorporeal support associated with technology change. Interact Cardiovasc Thorac Surg 11:400–405
Booth KL, Roth SJ, Perry SB, del Nido PJ, Wessel DL, Laussen PC (2002) Cardiac catheterization of patients supported by extracorporeal membrane oxygenation. J Am Coll Cardiol 40:1681–1686
Allan CK, Thiagarajan RR, del Nido PJ, Roth SJ, Almodovar MC, Laussen PC (2007) Indication for initiation of mechanical circulatory support impacts survival of infants with shunted single-ventricle circulation supported with extracorporeal membrane oxygenation. J Thorac Cardiovasc Surg 133:660–667
Ravishankar C, Dominguez TE, Kreutzer J, Wernovsky G, Marino BS, Godinez R, Priestley MA, Gruber PJ, Gaynor WJ, Nicolson SC, Spray TL, Tabbutt S (2006) Extracorporeal membrane oxygenation after stage I reconstruction for hypoplastic left heart syndrome. Pediatr Crit Care Med 7:319–323
Ghez O, Fouilloux V, Charpentier A, Fesquet P, Lion F, Lebrun L, Commandeur M, Fraisse A, Metras D, Kreitmann B (2007) Absence of rapid deployment extracorporeal membrane oxygenation (ECMO) team does not preclude resuscitation ECMO in pediatric cardiac patients with good results. ASAIO J 53:692–695
Huang SC, Wu ET, Chen YS, Chang CI, Chiu IS, Wang SS, Lin FY, Ko WJ (2008) Extracorporeal membrane oxygenation rescue for cardiopulmonary resuscitation in pediatric patients. Crit Care Med 36:1607–1613
Chow G, Koirala B, Armstrong D, McCrindle B, Bohn D, Edgell D, Coles J, de Veber G (2004) Predictors of mortality and neurological morbidity in children undergoing extracorporeal life support for cardiac disease. Eur J Cardiothorac Surg 26:38–43
Author information
Authors and Affiliations
Corresponding author
Rights and permissions
About this article
Cite this article
Sivarajan, V.B., Best, D., Brizard, C.P. et al. Duration of resuscitation prior to rescue extracorporeal membrane oxygenation impacts outcome in children with heart disease. Intensive Care Med 37, 853–860 (2011). https://doi.org/10.1007/s00134-011-2168-6
Received:
Accepted:
Published:
Issue Date:
DOI: https://doi.org/10.1007/s00134-011-2168-6