Abstract
Antibacterial properties of honey largely depend on the accumulation of hydrogen peroxide (H2O2), which is generated by glucose oxidase (GOX)-mediated conversion of glucose in diluted honey. However, honeys exhibit considerable variation in their antibacterial activity. Therefore, the aim of the study was to identify the mechanism behind the variation in this activity and in the H2O2 content in honeys associated with the role of GOX in this process. Immunoblots and in situ hybridization analyses demonstrated that gox is solely expressed in the hypopharyngeal glands of worker bees performing various tasks and not in other glands or tissues. Real-time PCR with reference genes selected for worker heads shows that the gox expression progressively increases with ageing of the youngest bees and nurses and reached the highest values in processor bees. Immunoblot analysis of honey samples revealed that GOX is a regular honey component but its content significantly varied among honeys. Neither botanical source nor geographical origin of honeys affected the level of GOX suggesting that some other factors such as honeybee nutrition and/or genetic/epigenetic factors may take part in the observed variation. A strong correlation was found between the content of GOX and the level of generated H2O2 in honeys except honeydew honeys. Total antibacterial activity of most honey samples against Pseudomonas aeruginosa isolate significantly correlated with the H2O2 content. These results demonstrate that the level of GOX can significantly affect the total antibacterial activity of honey. They also support an idea that breeding of novel honeybee lines expressing higher amounts of GOX could help to increase the antibacterial efficacy of the hypopharyngeal gland secretion that could have positive influence on a resistance of colonies against bacterial pathogens.
Similar content being viewed by others
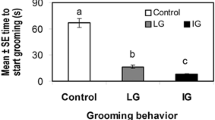
Avoid common mistakes on your manuscript.
Introduction
The vast majority of the population of a honeybee hive consists of workers that perform most tasks in the colony. A particular task is usually performed by workers of a certain age: cell cleaning and capping by the youngest bees, nursing (brood and queen care/feeding) by 4–16-day-old bees, food handling and comb building by middle-aged bees and ventilating, guarding and foraging by older bees between 15 and 30 days (Winston 1987). One of the important tasks is foraging of nectar from flowering plants by forager bees and its transfer to nestmates at the colony. The nectar can be directly used for feeding purposes or processed by regurgitation and passing among processor bees (to mix it with secreted enzymes) until it is placed in comb cells. Here, after final evaporation of water, it is processed into honey that represents a food storage possessing besides nutritional also antibacterial properties (De Grandi-Hoffman and Hagler 2000; Seeley 1992).
The antibacterial activity of honey has been extensively studied, and several honey antibacterial compounds have been identified. Hydrogen peroxide (H2O2) is one of the major antibacterial components and is produced by glucose oxidase (GOX)-mediated conversion of glucose to gluconic acid under aerobic conditions in diluted honey (White et al. 1963). Although H2O2 at concentrations found in honey does not kill bacteria, it is able to interact with bacterial cell proliferative signals, and thus, it affects bacterial growth even at high dilutions of the honey (Brudzynski 2006). Moreover, it has recently been demonstrated that honey H2O2 exerts DNA-degrading activities to bacterial cells (Brudzynski et al. 2011).
GOX (FAD oxidoreductase, EC 1.1.3.4) is an 85-kDa enzyme that is expressed in hypopharyngeal glands of the processor and forager bee and secreted into the nectar during the preparation of honey. It was not detected in hypopharyngeal glands of the nurse bee (Ohashi et al. 1999). On the contrary, recent studies (Li et al. 2008; Santos et al. 2005) showed that GOX is also expressed in the hypopharyngeal glands of the nurse and winter worker bees. Thus, GOX is secreted into the nectar and obviously also larval food where it acts as one means of sterilizing the food. This way, GOX may provide immunological protection at the colony level and prevent some larval diseases (Yang and Cox-Foster 2005). The level of GOX in honey could be one of the factors participating in prevention of bacterial and fungal infections in colonies as well as a mean/marker in selection of honeys with high antibacterial activities.
The levels of H2O2 in honey may differ from honey to honey without relation to its botanical and geographical origin (Brudzynski et al. 2011). The following factors have been proposed to affect the total concentration of H2O2 in honey: (i) inactivation of GOX due to exposure to excess heat or light (White et al. 1963), (ii) chemical scavenging of H2O2 (White et al. 1963), (iii) destruction of H2O2 by catalase occurring in nectar and pollen (Brudzynski 2006), (iv) modification of GOX by methylglyoxal (Majtan et al. 2014) and (v) autooxidation of polyphenols/flavonoids (Brudzynski 2006). Another plausible explanation for the variation in H2O2 content in honey could be differences either in levels of bee-derived GOX or GOX enzymatic activity that could be significantly affected by compounds present in honey or honeybee protein diets (Alaux et al. 2010).
The aims of this study were to (i) evaluate simultaneously the content of bee GOX and H2O2 in honey samples of different botanical and geographical origins; (ii) localize the gox expression in honeybee workers performing different tasks: cleaner, nurse, processor and forager bee; (iii) examine gox expression in workers during their behavioural development; and (iv) characterize the antibacterial activity of honey samples against Gram-negative bacteria (Pseudomonas aeruginosa).
Materials and methods
Honeybee samples
Apis mellifera carnica workers of particular ages (2–30 days) for qPCR were obtained from two naturally mated queen colonies in the apiary of the Institute of Apiculture in Liptovský Hrádok, Slovakia, in July of 2012. Experimental bees were 11–19 h old when were colour-marked on their thorax to denote their age.
For in situ hybridization, A. mellifera carnica cleaner, nurse, processor and forager bees were obtained from naturally mated queen colonies in the apiary of Mr. Dedinsky (Bratislava, Slovakia) between June and September of 2013.
Honey samples
Honey samples (n = 20) were received from beekeepers throughout several regions of Slovakia between May and August of 2013 (Table 1). Honey samples were stored in glass or plastic containers at room temperature in the dark. Identification of the floral source of the honey was performed by the beekeepers based on the availability of flora for nectar foraging, location of the apiary and organoleptic characteristics of the honey.
Microorganisms
The antibacterial activity of honey samples was assessed against isolate of P. aeruginosa. Bacterial clinical isolate from non-healing wound was collected from the Department of Clinical Microbiology of the Hospital Čadca (Čadca, Slovakia) and transported to the Department of Medical Microbiology, Slovak Medical University (Bratislava, Slovakia).
The reason to use Gram-negative bacteria for testing antibacterial activity of honeys is based on fact that defensin-1, a bee-derived antibacterial peptide in honey, is effective solely against Gram-positive bacteria. Thus, we can evaluate the antibacterial activity of generated H2O2 in honey samples without contribution of defensin-1.
Ultrafiltration of honey samples
Each honey sample (2.5 g) was dissolved with deionized water to a final volume of 5 ml until completely fluid. The liquid solution obtained was filtered through a 0.22-μm PES filter (Millipore, MA, USA) and then concentrated by centrifugation at 5,000 g at room temperature in a Vivaspin 6 concentrator tube (Sartorius, Germany), with an exclusion limit of 10 kDa, to a final volume of 1 ml. The Vivaspin retentate was subsequently stored at −20 °C.
Anti-honeybee GOX polyclonal antibody
An affinity-purified polyclonal anti-honeybee GOX antibody was purchased from GenCust Europe (Dudelnag, Luxembourg). Two New Zealand rabbits immunized with a synthetic peptide corresponding to the C terminus of bee GOX (CDEFVEDSDDYWN) provided antibodies that were then affinity-purified using this peptide.
Detection of GOX in honeys and honeybee gland extracts by immunoblotting
The retentates of the honey samples from ultrafiltration and gland extracts of unspecified hive bees (5 glands/200 μl of PBS) were electrophoresed on SDS-PAGE gels using a Mini-Protean II electrophoresis cell (Bio-Rad, CA, USA). The proteins were transferred onto a 0.22-μm nitrocellulose Advantec® membrane (Sigma-Aldrich, UK) in 48 mM Tris, 39 mM glycin and 20 % methanol using the semi-dry blotting procedure. The membrane was blocked for 1 h in a TBST buffer (50 mM Tris–HCl, pH 7.5, 200 mM NaCl, 0.05 % Tween 20) containing 5 % non-fat dried milk and then incubated overnight with a rabbit polyclonal antibody against honeybee GOX diluted 1:2,000 in TBST-blocking buffer. After washing with TBST, the membrane was incubated for 2 h in blocking buffer containing goat anti-rabbit HRP-linked antibodies (Promega, WI, USA) diluted 1:2,500. Immunoreactive bands were detected in solution containing dissolved SigmaFast 3,3-diaminobenzidine tablets (Sigma-Aldrich, UK). In the case of honey samples, the signal intensity of GOX bands was quantified by densitometry (Quantity One, Bio-Rad, USA) and expressed as the relative volume intensity (intensity*mm2).
Determination of antibacterial activity
The antibacterial efficacy of various honey samples analyzed was evaluated by the minimum inhibitory concentration (MIC) assay. Briefly, one bacterial colony was suspended in PBS buffer, pH 7.2, and the turbidity of the suspension was adjusted to 108 colony forming units (CFU)/ml and diluted with Mueller-Hinton broth (MHB) medium to a final concentration of 106 CFU/ml. Ten-microlitre aliquots of suspension were inoculated into each well of sterile 96-well polystyrene plates (Sarstedt, Germany). The final volume in each well was 100 μl, consisting of 90 μl of sterile medium or diluted honey and 10 μl of bacterial suspension. After 18 h of incubation at 37 °C, bacterial growth inhibition was determined by monitoring the optical density at 490 nm. The MIC was defined as the lowest concentration of honey inhibiting bacterial growth. All tests were performed in triplicate and were repeated three times.
A serial twofold dilutions of each honey were prepared from 40 to 50 % (w/v) honey solution, resulting in a final concentrations of 50, 40, 25, 20, 12.5, 10, 6.25, 5, 3.12, 2.5 and 1.25 %.
Determination of H2O2 concentration in honey
The maximum levels of accumulated H2O2 that occurred in honey solutions were found in solutions diluted to concentrations between 30 and 50 % (Bang et al. 2003). Therefore, the levels of H2O2 were determined in 40 % honey solutions. Each honey sample (0.8 g) was dissolved with 1.2 g of distilled water until completely fluid. The 40 % (w/w) liquid solutions obtained were incubated at 37 °C for 4 h. Hydrogen peroxide concentrations in the honey solutions after each indicated time of incubation were determined using a hydrogen peroxide/peroxidase fluorimetric kit (Cell Biolabs Inc, CA, USA) according to the manufacturer’s instructions. The fluorescence of the formed product, resorufin, was measured at an excitation wavelength of 530 nm using a 590-nm emission line with a Synergy HT microplate reader (BioTek Instruments, VT, USA). Each honey sample and standard was tested in duplicate.
In order to prove that GOX, a heat-labile enzyme, is responsible for H2O2 generation, 40 % honey solutions were heat-treated at 95 °C for 5 min and incubated at 37 °C for 4 h. Hydrogen peroxide concentrations in heat-treated honey samples were determined as described above. Changes in H2O2 concentrations in untreated and heat-treated honey samples were expressed as a percentage in reduction of H2O2 levels.
Comparison of gox transcript levels in heads of workers by qPCR
RNA isolation and cDNA synthesis
Total RNA and complementary DNA (cDNA) from pooled head samples were prepared as previously described (Kohutova et al. 2013). Briefly, each sample was prepared from the heads of four workers of a particular age. RNA was isolated by guanidine thiocyanate-acid phenol extraction followed by spin column purification. Three sets of samples from bees in the age range of 2–30 days were prepared from a colony. First-strand cDNA was synthesized from 1.6 μg of total RNA in a 20-μl reaction using oligo (dT)18 primer.
Reference gene selection
Reference genes for studying expression in heads of workers performing various tasks were selected from among five candidate reference genes (Table 2) we have recently found to be stably expressed in the heads of nurses (Kohutova et al. 2013). The most suitable reference genes were selected on the basis of their expression stabilities evaluated in pooled head samples of bees aged 2–30 days (for particular ages, see Fig. 3) in two colonies. This was accomplished employing the programs geNormPLUS (part of qbasePLUS software, version 2.5.1) (Hellemans et al. 2007; Vandesompele et al. 2002) and NormFinder, version 0.953 (Andersen et al. 2004). Input data used for these programs were generated previously from qPCR expression analyses of the genes (Kohutova et al. 2013). The data used for geNormPLUS were raw Cq values of genes, and the data used for NormFinder were relative quantities of genes, calculated from Cq values by the ΔCq method.
GeNormPLUS evaluated the expression stability of all tested genes as high (criterion M value ≤0.5) and recommended usage of two reference targets (ranks 1 and 2) for each colony. NormFinder ranked the genes equally or similarly as geNormPLUS in the colonies A and B, respectively, and determined the most suitable combinations of two reference genes to be cyclophilin-gapdh in the colony A and cyclophilin-psa1 in the colony B (Table 2). As a normalization factor suitable for both colonies, the geometric mean of three genes with the best overall ranking (considering both colonies)—cyclophilin, psa1 and gapdh—was selected.
qPCR and data analysis
The qPCR amplification strategy and amplification conditions were the same as described by Kohutova et al. (2013). Briefly, each sample was analyzed in duplicate in a reaction of 15 μl containing 5 μl of diluted cDNA (1:50 in water) and 0.4 μM primers (Table 3). The PCR reactions were performed with Hot FirePol EvaGreen qPCR mix plus (no ROX) (Solis BioDyne, Estonia) on a CFX96 thermocycler (Bio-Rad, USA) using the following conditions: 95 °C for 15 min and 40 cycles at 95 °C for 15 s, 58 °C for 20 s, and 69 °C for 20 s. The specificity of amplification was monitored by melting curve analysis, electrophoretic determination of amplicon sizes and sequencing of the PCR products. No-reverse transcriptase and no-template control reactions showed none or not significant contaminations. The Biorad CFX Manager 3.0 software was employed for the determination of Cq values and the estimation of relative normalized transcriptional levels of gox gene in samples of all sets of a colony related to the lowest value.
In situ hybridization
Primers for the synthesis of a digoxigenin (Dig)-labelled probe were designed based on the honeybee gox cDNA sequence as follows: sense primer, 5′-TACCCGACCTTCAACCAGAC-3′; antisense primer, 5′-ATTCTCCCCAATCCTCCTTG-3′. The 706-bp amplicon was re-amplified using the PCR Dig Probe synthesis kit (Roche Applied Science, Germany) to synthesize the Dig-labelled antisense DNA probe. The Dig-labelled probe was purified using a PCR Purification kit (Promega, WI, USA) and stored at −20 °C. The hypopharyngeal glands, salivary glands and mandibular glands, fat bodies and guts were dissected from honeybees performing a particular task, fixed in 4 % paraformaldehyde (w/v) at 4 °C overnight and subjected to the whole-mount in situ hybridization procedure as described elsewhere (Kim et al. 2006).
Statistical analysis
One-way ANOVA was used for evaluation of data from qPCR analysis. The Pearson correlation test was used for correlation analysis between antibacterial activity/relative content of GOX and H2O2 production in honeys. The data are expressed as mean values with the standard error of the mean (SEM). Data with P values smaller than 0.05 were considered as statistically significant. All statistical analyses were performed using GraphPad Prism (GraphPad Software Inc., La Jolla, CA, USA).
Results
Localization of gox expression in workers performing distinct tasks
Extracts of mandibular, hypopharyngeal, head salivary and thorax salivary glands were separated on SDS-PAGE, blotted onto nitrocellulose and immunostained with anti-honeybee GOX antibody. A specific immunoreactive band with a size of around 85 kDa was detected only in extracts of hypopharyngeal glands suggesting that the antibody has high specificity, and there was no cross-reaction with other proteins in the extracts (Fig. 1).
Immunospecificity of an anti-GOX polyclonal antibody evaluated by immunoblot assays. Gland extracts of unspecified hive bees (5 glands/200 μl of PBS) were electrophoresed on a 12 % SDS-PAGE gel a stained with Coomassie Blue R or b immunoblotted with a purified rabbit polyclonal antibody against honeybee GOX. MG mandibular glands, HG hypopharyngeal glands, HSG head salivary glands, TSG thorax salivary glands
Using the specific cDNA probe, we detected strong expression of gox mRNA in the hypopharyngeal glands of all selected bees (Fig. 2). The expression level in hypopharyngeal glands remained strong throughout the behavioural development of the worker bees from cleaners to foragers. No expression of gox was detected in mandibular, head salivary and thorax salivary glands of the worker bees. Similarly, no gox expression was detected in the fat body and gut (data not shown).
Localization of gox expression in different glands of workers specialized in different tasks performed within a colony at different ages. The expression was localized in glands by in situ hybridization through a microscope with × 100 magnification. Workers performing the following tasks were used in the study: cleaner, nurse, processor and forager. MG mandibular glands, HG hypopharyngeal glands, HSG head salivary glands, TSG thorax salivary glands. Note that nurse and processor bees contain larger acines in the HG as compared to cleaner and forager bees
Gox expression in heads of workers of various ages
Transcript levels of gox using cyclophilin, psa1 and gapdh as reference genes were compared in three sets of pooled head samples from bees of different ages in two colonies (A and B). In our previous work, we found that these colonies differed in age-related division of labour (Kohutova et al. 2013). The comparisons of transcription levels of gox in the heads of workers of colonies A and B normalized to the geometric means of the reference genes are shown in Fig. 3.
Relative transcription levels of gox in pooled head samples of workers of various ages from colony A (a) and colony B (b). Three reference genes (cyclophilin, psa1 and gapdh) were used for normalization. Data are expressed as mean values ± standard error of the mean (SEM). qPCR analyses were performed on three independent experiments, with each qPCR performed in duplicate
In both colonies, gox expression showed similar patterns in relation to the assumed division of labour: The expression was progressively increasing with ageing of the youngest bees including cleaners and nurses and reached the highest levels in nectar processors/foragers bee (normalized transcription levels 3.6–4.5 times higher than those in 2-day-old bees). Between the examined colonies, partial differences can be observed in gox expression levels among workers assumed to deal with nectar processing and foraging.
Comparison of GOX content and H2O2 production in honey samples
The content of GOX in the honey samples was examined in their ultrafiltration retentates in which only substances with molecular weights (MWs) of more than 10 kDa including GOX should be present. Bands of GOX with sizes of around 85 kDa were detected on the immunoblots using an anti-honeybee GOX antibody for all honey samples (data not shown). The retentates of the 20 honey samples analyzed, with various botanical and geographical origins, showed differences in their GOX content (Fig. 4a). Differences in the GOX content were observed among the honey samples of the different and also the same botanical origin. They were also observed among honey samples of different geographical origin and harvesting time. These observations indicate that the amount of GOX in honey depends on the GOX production capacity of honeybees in colonies.
Content of GOX and H2O2 production in the honey samples. GOX levels in equal volumes of honey retentates (15 μl) were determined by immunobloting using a polyclonal antibody against GOX. a The signal intensity of immunoreactive bands was quantified by densitometry and expressed as a relative volume intensity (intensity*mm2). Accumulation of H2O2 was determined in 40 % (w/w) honey solutions after a 4-h incubation at 37 °C using a hydrogen peroxide/peroxidase fluorimetric kit. Data are expressed as mean values ± SEMs. b Reduction of H2O2 generation in heat-treated 40 % honey samples. c Relationship between H2O2 and GOX content in natural honeys expressed as a relative volume intensity (intensity*mm2). A Pearson correlation test was used for correlation analysis
The intensity of GOX bands and the level of generated H2O2 showed a significant correlation (n = 20, r = 0.745, P = 0.0002) almost in all honey samples (Fig. 4c). Two samples (No. 12 and 13) of honeydew honeys contained a relatively low level of GOX but were able to produce a high level of H2O2 (~200 μM) (Fig. 4a). Levels of H2O2 in heat-treated honey samples were significantly reduced in all honeys (Fig. 4b) suggesting that GOX is a principal factor responsible for H2O2 generation.
Antibacterial activity of honey samples
The MIC values of 20 natural honeys against the tested bacterial strain are shown in Fig. 5a. Honey samples were differentially effective against a Gram-negative P. aeruginosa bacterial isolate. The MICs of honeys for P. aeruginosa ranged from 5 to 40 %. Honey samples Nos. 17, 18 and 19 with an unspecified mixed floral origin possessed the strongest antibacterial activity against tested bacteria.
Antibacterial activity of honeys (n = 20) against Pseudomonas aeruginosa isolate. a The activity was determined by a minimum inhibitory concentration (MIC) assay. The MIC was defined as the lowest concentration of honey solution in percent inhibiting bacterial growth. b Relationship between H2O2 content and antibacterial activity of natural honeys against P. aeruginosa. A Pearson correlation test was used for correlation analysis
The results of the Pearson r correlation test comparing the level of accumulated H2O2 and antibacterial activity of each honey sample revealed that there was a significant concentration-dependent correlation between the production of H2O2 and antibacterial activity against P. aeruginosa (n = 20, r = −0.673, P = 0.0012) (Fig. 5b).
Discussion
In this work, we demonstrated that gox is constitutively and solely expressed in the hypopharyngeal glands of workers performing various tasks. The least expression is in cleaners, and the highest and high amounts occurred in processors and foragers, respectively, which both secrete GOX into the nectar during its processing into honey. Thus, our results indicate that GOX is secreted by nurses into larval jelly and by processors and foragers into honey. Further, we present data confirming that GOX is a regular component of natural honeys and show that the amount of GOX and its enzymatic activity determined as the amount of generated H2O2 in diluted honeys varied significantly in the different honeys examined. Our results correspond with the results of others who demonstrated the presence of GOX in the hypopharyngeal glands of foragers (Ohashi et al. 1999), nurses and winter worker bees (Li et al. 2008; Santos et al. 2005) and expression of gox in the heads of nurses and foragers collecting pollen (Liu et al. 2011).
Analyses of honey samples revealed that the amount of GOX varied significantly from honey to honey and that this variation seems to have a multifactorial character. The content of GOX varied between honeys of different floral sources but also within the same floral source from different geographical origins. Interestingly, the greatest amount of GOX was found in honeys with an unspecified mixed botanical source. One of the possible explanations is that the content of GOX in honey was affected by pollen nutrition in the honeybee colony. It has been shown that polyfloral pollen diets enhanced some immune functions compared with monofloral diets, in particular GOX activity in honeybee heads, suggesting that diversity in floral resources can have a direct effect on the expression of immune and antibacterial factors including GOX (Alaux et al. 2010). On the other hand, the variation in GOX content may be associated with genetic diversity of honeybees (various genotypes). Our previous studies documented that the levels of bee antimicrobial peptide defensin-1 vary in larval jelly and honey samples, and the obtained results indicated that the variations are determined by genetic factors (Klaudiny et al. 2012; Majtan et al. 2012). Variations in the amount of major royal jelly protein 1 in natural honeys have also been described (Bilikova and Simuth 2010). It is likely that the levels of more bee-derived proteins/peptides in honey, including those contributing to honey antibacterial activity (e.g. GOX), could be influenced by honeybee genetic/epigenetic factors.
Another factor affecting the level of H2O2 might be catalase, an enzyme which efficiently hydrolyzes H2O2 to oxygen and water. Although the total concentration of catalase depends on the amount of pollen grains in honey (Brudzynski et al. 2011), no data are available regarding the exact concentration of catalase in honey. In addition, it has been shown that the amount of catalase necessary to destroy the antibacterial activity of honey was found to be unexpectedly high (White et al. 1963). In present study, we showed a significant strong correlation between the relative amount of GOX and generated H2O2. Therefore, we assumed that the natural content of catalase in honey did not significantly interfere with the presentation of hydrogen peroxide in honeys. According to our findings, H2O2 accumulated in every honey upon dilution, which correlates with the constitutive presence of GOX in honeys. However, not all diluted honeys (e.g. manuka honey) are able to form H2O2 (Kwakman et al. 2011). The reason manuka honey, a pronounced medical-grade honey, does accumulate only negligible amounts of H2O2 has been revealed just recently (Majtan et al. 2014). We documented that methylglyoxal, a major antibacterial factor of manuka honey, induces a modification of significant proteinaceous components (e.g. GOX and defensin-1) of honey resulting in a loss of their biological activities (Majtan et al. 2014; Majtan et al. 2012).
Until now, a very few studies have attempted to determine the concentration of H2O2 in different honeys and to evaluate its effect on the overall antibacterial activity of honeys (Brudzynski 2006; Chen et al. 2012). Brudzinsky and co-workers (2006) tested 42 Canadian natural honeys and found that all honeys exhibited antibacterial activity, with the higher efficacy against Gram-negative Escherichia coli than Gram-positive Bacillus subtilis, and that these antibacterial activities were correlated with H2O2 production in the honeys. Similarly, other study by Chen et al. (2012) showed a greater correlation between antibacterial activity against Staphylococcus aureus and amount of H2O2 in Australian honey samples. These findings are in agreement with our results where a significant correlation between antibacterial activity and H2O2 production was observed. However, Slovak natural honeys were more effective against Gram-positive bacteria than Gram-negative P. aeruginosa (data not shown). In our previous work (Majtan et al. 2012), antibacterial bee-derived peptide defensin-1 was shown to be a regular component of each honey, and it significantly contributes to the overall antibacterial activity against Gram-positive bacteria, but it is ineffective against Gram-negative bacteria.
Two samples of honeydew honey tested in this study showed a high capacity of H2O2 production (over 180 μM) even though the amount of GOX in these samples was low. Honeydew honey is known for the high content of phenolic acids and flavonoids possessing antioxidant and pro-oxidant properties (Alvarez-Suarez et al. 2013). In the presence of transitional metal, polyphenols have been shown to be involved in the generation of substantial amounts of H2O2 (Akagawa et al. 2003; Long et al. 2010). Therefore, specific plant-derived polyphenols in honey could be an additional source of H2O2 as documented for our two honey samples. Thus, honeydew honey might be an ideal candidate for clinical applications (Cernak et al. 2012; Vlcekova et al. 2012).
In conclusion, our data constitute the first direct evidence that gox is constitutively expressed in hypopharyngeal glands of workers performing various tasks, and GOX is a regular but quantitatively variable component of natural honeys. Expression of GOX seems to progressively increase in the hypopharyngeal glands with the age of worker bees, reaching the highest levels in processor bees that are responsible for the processing of nectar into honey. We showed that the level of GOX in honeys positively correlated with H2O2 accumulation and, thus, that GOX affects the total antibacterial activity of honey. The assumed dependency of GOX levels in honeys, and also larval jellies from bee genetic/epigenetic factors, needs to be investigated and confirmed. Breeding of novel honeybee lines expressing higher amounts of GOX could be employed in such research. Such lines should contain workers producing secretions of hypopharyngeal glands with increased antibacterial efficacy that could have a positive effect on a resistance of colonies against bacterial pathogens.
References
Akagawa M, Shigemitsu T, Suyama K (2003) Production of hydrogen peroxide by polyphenols and polyphenol-rich beverages under quasi-physiological conditions. Biosci Biotechnol Biochem 67:2632–2640
Alaux C, Ducloz F, Crauser D, Le Conte Y (2010) Diet effects on honeybee immunocompetence. Biol Lett 6:562–565
Alvarez-Suarez JM, Giampieri F, Battino M (2013) Honey as a source of dietary antioxidants: structures, bioavailability and evidence of protective effects against human chronic diseases. Curr Med Chem 20:621–638
Andersen CL, Jensen JL, Ørntoft TF (2004) Normalization of real-time quantitative reverse transcription-PCR data: a model-based variance estimation approach to identify genes suited for normalization, applied to bladder and colon cancer data sets. Cancer Res 64:5245–5250
Bang LM, Bantting C, Molan PC (2003) The effects of dilution rate on hydrogen peroxide production in honey and its implications for wound healing. J Altern Complement Med 9:267–273
Bilikova K, Simuth J (2010) New criterion for evaluation of honey: quantification of royal jelly protein apalbumin 1 in honey by ELISA. J Agric Food Chem 58:8776–8781
Brudzynski K (2006) Effect of hydrogen peroxide on antibacterial activities of Canadian honeys. Can J Microbiol 52:1228–1237
Brudzynski K, Abubaker A, St-Martin L, Castle A (2011) Re-examining the role of hydrogen peroxide in bacteriostatic and bactericidal activities of honey. Front Microbiol 2:1–9
Cernak M, Majtanova N, Cernak A, Majtan J (2012) Honey prophylaxis reduces the risk of endophthalmitis during perioperative period of eye surgery. Phytother Res 26:613–616
Chen C, Campbell LT, Blair SE, Carter DA (2012) The effect of standard heat and filtration processing procedures on antimicrobial activity and hydrogen peroxide levels in honey. Front Microbiol 3:1–8
De Grandi-Hoffman G, Hagler J (2000) The flow of incoming nectar through a honey bee (Apis mellifera L.) colony as revealed by a protein marker. Insectes Soc 47:302–306
Hellemans J, Mortier G, De Paepe A, Speleman F, Vandesompele J (2007) qBase relative quantification framework and software for management and automated analysis of real-time quantitative PCR data. Genome Biol 8:R19
Kim YJ, Zitnan D, Cho KH, Mizoguchi A, Schooley D, Adams ME (2006) Endocrine programming of an innate behavior through central release of peptide cotransmitters. Proc Natl Acad Sci U S A 103:14211–14216
Klaudiny J, Bachanova K, Kohutova L, Dzurova M, Kopernicky J, Majtan J (2012) Expression of larval jelly antimicrobial peptide defensin1 in Apis mellifera colonies. Biologia 67:200–211
Kohutova L, Klaudiny J, Nadasdy R, Sediva M, Kopernicky J, Majtan J (2013) Identification and validation of reference genes for real time PCR expression studies in heads of nurse honeybees. Biologia 68:1211–1220
Kwakman PH, Te Velde AA, De Boer L, Vandenbroucke-Grauls CM, Zaat SA (2011) Two major medicinal honeys have different mechanisms of bactericidal activity. PLoS ONE 6:e17709
Li J, Feng M, Zhang Z, Pan Y (2008) Identification of the proteome complement of hypopharyngeal glands from two strains of honeybees (Apis mellifera). Apidologie 39:199–214
Liu F, Li W, Li Z, Zhang S, Chen S, Su S (2011) High-abundance mRNAs in Apis mellifera: comparison between nurses and foragers. J Insect Physiol 57:274–279
Long LH, Hoi A, Halliwell B (2010) Instability of, and generation of hydrogen peroxide by, phenolic compounds in cell culture media. Arch Biochem Biophys 501:162–169
Majtan J et al (2012) Methylglyoxal-induced modifications of significant honeybee proteinous components in manuka honey: possible therapeutic implications. Fitoterapia 83:671–677
Majtan J, Bohova J, Prochazka E, Klaudiny J (2014) Methylglyoxal may affect hydrogen peroxide accumulation in manuka honey through the inhibition of glucose oxidase. J Med Food 17:290–293
Ohashi K, Natori S, Kubo T (1999) Expression of amylase and glucose oxidase in the hypopharyngeal gland with an age-dependent role change of the worker honeybee (Apis mellifera L.). Eur J Biochem 265:127–133
Santos KS, Delazari Dos Santos L, Anita Mendes M, Monson De Souza B, Malaspina O, Palma MS (2005) Profiling the proteome complement of the secretion from hypopharyngeal gland of Africanized nurse-honeybees (Apis mellifera L.). Insect Biochem Mol Biol 35:85–91
Seeley TD (1992) The tremble dance of the honey bee: message and meanings. Behav Ecol Sociobiol 47:311–316
Vandesompele J, De Preter K, Pattyn F, Poppe B, van Roy N, De Paepe A, Speleman F (2002) Accurate normalization of real-time quantitative RT-PCR data by geometric averaging of multiple internal control genes. Genome Biol 3:R34
Vlcekova P, Krutakova B, Takac P, Kozanek M, Salus J, Majtan J (2012) Alternative treatment of gluteofemoral fistulas using honey: a case report. Int Wound J 9:100–103
White JW, Subers MH, Schepartz AI (1963) The identification of inhibine, the antibacterial factor in honey, as hydrogenperoxide and its origin in a honey glucose-oxidase system. Biochim Biophys Acta 73:57–70
Winston ML (1987) The biology of honey bees. Harvard University Press, Cambridge
Yang X, Cox-Foster DL (2005) Impact of an ectoparasite on the immunity and pathology of an invertebrate: evidence for host immunosuppression and viral amplification. Proc Natl Acad Sci U S A 102:7470–7475
Acknowledgments
We would like to thank Mr. Dusan Dedinsky and Dr. Robert Nadasdy as well as Dr. Jan Kopernicky for help at collecting of workers of particular tasks and defined ages, respectively. This work was funded by the Operational Program Research and Development and co-financed by the European Fund for Regional Development (EFRD) via grant ITMS 26240220030—“Research and development of new biotherapeutic methods and its application in some illnesses treatment”—and by the Scientific Grant Agency of the Ministry of Education of the Slovak Republic and the Slovak Academy of Sciences VEGA 2/0178/12.
Author information
Authors and Affiliations
Corresponding author
Additional information
Communicated by: Sven Thatje
Rights and permissions
About this article
Cite this article
Bucekova, M., Valachova, I., Kohutova, L. et al. Honeybee glucose oxidase—its expression in honeybee workers and comparative analyses of its content and H2O2-mediated antibacterial activity in natural honeys. Naturwissenschaften 101, 661–670 (2014). https://doi.org/10.1007/s00114-014-1205-z
Received:
Revised:
Accepted:
Published:
Issue Date:
DOI: https://doi.org/10.1007/s00114-014-1205-z