Abstract
The kinetics of the thermal decomposition of diazacyclic derivatives of 1,1-diamino-2,2-dinitroethylene (FOX-7) in a dilute solution of nitrobenzene and in the solid phase are studied by manometric and calorimetric methods. The activation energy data obtained for the solution are used for comparison with quantum chemical calculations and for establishing the relationship between the stability of compounds and the structure of the molecules. The decomposition of these derivatives in solution proceeds according to the same mechanism as the decomposition of FOX-7. Their activation energies decrease with the increasing C=C bond length and are in close agreement with the published data on quantum chemical calculations. By the nature of decomposition in the solid state, these compounds differ significantly from FOX-7. This difference is due to the fact that the condensed products of their decomposition are not solid but liquid compounds.
Similar content being viewed by others
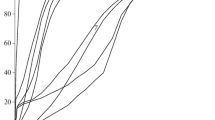
Avoid common mistakes on your manuscript.
INTRODUCTION
Interest in the kinetic study of 2-(dinitromethylene)-1,3-diazacyclopentane (I), 2-(dinitromethylene)-1,3-diazacyclohexane (II) and 2-(dinitromethylene)-1,3-diazacycloheptane (III) (Table 1) is largely due to the fact that all of them are analogs of the well-known explosive 1,1-diamino-2,2-dinitroethylene (FOX-7), the decomposition of which in the solid state revealed a number of patterns that are not characteristic of other nitro compounds [1–5]. It is of great interest to find out to what extent these unusual decay patterns of FOX-7 are preserved in its closest analogs, compounds I–III.
The main feature of FOX-7 is the two-stage nature of its decomposition in the solid phase. This feature is most pronounced when studying the decomposition of FOX-7 by differential scanning calorimetry (DSC) in an inert gas flow. At very different linear heating rates, two peaks of heat release are observed, separated by a temperature interval exceeding 50°C, and both peaks due to the decomposition of FOX-7 itself. It was proved [3] that the first stage is a side process related to the sublimation and rapid decomposition of substance in the gas phase. The uniqueness of the gas-phase reaction lies in the fact that one of its products is a refractory and nonvolatile compound at the temperature of the experiment, which is deposited on the surface of the crystals in the form of a thin film that prevents sublimation. Under the flow’s conditions, the film forms slowly, and up to 40% of the mass of the substance is lost in the region of the first heat release peak. In closed vessels, the first stage ends quickly. The fraction of the substance decomposed in the first stage depends on the ratio of the mass of sample m to free volume V and may be very small. Further decomposition proceeds in the solid phase “under the film,” the destruction of which is accompanied by a sharp increase in the rate.
Unlike all other nitro compounds, the decomposition of FOX-7 proceeds without the formation of a liquid phase. The reaction proceeds according to the “Solid → solid + Gas” type. The solid residue is a powder with nanosized particles, which does not exhibit catalytic activity.
An experimental study of the decomposition of compounds I–III was carried out in [6, 7]. In [6], the study was carried out by DSC in an argon flow. The maximum heat release for I–III occurs at temperatures of 207 to 263°C; i.e., it is in the region of the first peak of the decomposition of FOX-7 (235°C). However, the second heat release peak is absent for all three compounds. It can be concluded that, unlike FOX-7, the decomposition of its analogs in an argon flow proceeds in one stage. The temperature at which the maximum rate is reached decreases in the series
This series does not coincide with the series of the increase in volatility
From this we can conclude that the substances differ not only in volatility but also in the rate of decomposition in the gas phase. Compound I is more volatile than FOX-7 [7], and at the same time, its heat release peak is at a higher temperature than FOX-7. Therefore, a reliable conclusion that can be drawn from [6] is that compound I is more stable in the gas phase than FOX-7.
In [7], the kinetics of heat release was studied in closed vessels. The reaction has an autocatalytic character with a pronounced induction period, the duration of which decreases in the series
It can be noted, however, that this series may not correspond to the order of the change in stability, since the induction period largely depends on the rate of the catalytic process, and not the initial stage.
The quantum-chemical calculation of the activation energy for various decay channels [6] showed that for compounds I–III the most profitable channel is isomerization to nitronic acid:

i.e., the mechanism previously established for FOX-7 is retained [3]. The activation energy, and hence the stability, decrease in the series
In this paper, the decomposition reactions of compounds I–III are studied in more detail. The reactions are studied not only in the solid state but also in dilute solutions of inert solvents. Previously, it was found that the general pattern of decomposition reactions of nitroolefins with an amino group in the cis-position consists of the independence of the decomposition reaction rate from the polarity of the solvent. This is also characteristic of FOX-7 [5].
EXPERIMENTAL
The FOX-7 samples used in this study and the equipment for manometric and calorimetric measurements at elevated pressures are described in [3, 4]. The substances were synthesized according to the procedure described in [9]
Decomposition in a solution. As shown in [5, 8], the isomerization reactions of amino-nitroolefins proceed with a slight change in the dipole moment, and therefore their rate does not depend on the polarity of the medium. In relation to this, the decomposition of compounds I–III was studied in only one solvent, nitrobenzene, which is highly stable, inert to the action of NO2, and dissolves I–III well.
Examples of kinetic gas evolution curves for compounds I–III are shown in Fig. 1. It can be seen that compound II has the highest decomposition rate, but the difference between compounds III and II is small. Decomposition I is much slower than the decomposition of compounds II and III. Compound I has the same decomposition rate at 250°C as compound II at 220°C. All reactions are described well by a 1st order equation. The kinetic parameters of the decomposition of compounds I–III in nitrobenzene are presented in Table 2. The same table shows the data for FOX-7 taken from [5].
Examples of kinetic curves for decomposition of compounds I, II, and III in a solution of nitrobenzene at a concentration of 1.0 wt %. Compound, temperature (°C): (1) II, 210; (2) III, 210; (3) II, 200; (4) III, 200; (5) I, 220. The circles correspond to the experimental decomposition points of compound I at 250°C.
Decomposition in the solid phase. The kinetics of the decomposition of compounds I–III are studied by the calorimetric method. The primary kinetic curves in the “heat release rate–time” coordinates at different temperatures for all three compounds are shown in Figs. 2–4.
The initial side reaction of decomposition through the gas phase, which is clearly manifested in the case of FOX-7 [3], is also noticeable for compounds I–III. In all three cases, at the beginning of the decomposition, heat release is observed, which proceeds at a decreasing rate (Fig. 5). After the completion of this reaction, the sample turns dark brown. As with FOX-7, with m/V ∼ 10–2 g/cm3, the conversion of the decomposition which is achieved as a result of the passage of a gas-phase reaction is negligible.
The condensed products that remain after the decomposition of compounds I–III are a black viscous liquid. Based on the example of compound III, it was shown that the addition of this residue to a fresh portion of the substance leads to a sharp increase in the decomposition rate (Fig. 6); i.e., the final decomposition products exhibit high catalytic activity.
In each experiment, the total thermal effect of reaction Q∞ was determined. After that, the kinetic curves were transformed in the usual way to the integral dependence Qcur (t) and to eliminate errors made in determining m/V, they are reduced to the form η(t), where η is the degree of decomposition, equal to the ratio of the current heat to the total thermal effect of the reaction: η = Qcur/Q∞. For compound I these data are shown in Fig. 7. A comparison of the kinetic heat release curves for compounds II and III is shown in Fig. 8. From Figs. 7 and 8, it can be seen that in the solid phase as in a solution, the least stable compound is II. However, the difference between compounds III and II is small, and both of these substances in terms of stability in the solid phase, are significantly inferior to compound I.
The decomposition of compounds I–III in the solid phase is characterized by strong acceleration. The rate of the catalytic reaction strongly depends on the value of the ratio m/V. At the same time, in the initial sections, as can be seen from Fig. 2, which shows the results of experiments at 198°C for two values m/V, the effect of the ratio m/V is not observed at the initial stage. The values of the rate constants of the initial stage, k1, were determined by extrapolating the specific rate dη/dt(1 – η) to the value t = 0. The data obtained are presented in Table 1 and in Fig. 9.
From a comparison of Fig. 1 with Figs. 7 and 8, it follows that the decomposition rate in the acceleration stage in the solid phase is much higher than the reaction rate in a solution. The rate constant describing the stage of accelerated decomposition of, for example, substance I, is hundreds of times greater than k1. In addition, it should be noted that the maximum decomposition rate in the solid phase is observed at the end of the reaction.
DISCUSSION OF THE RESULTS
The independence of k1 from the polarity of the solvent makes it possible to use the experimental data obtained in a solution for comparison with the quantum chemical calculations of the activation energy performed for the reaction in the gas phase. The corresponding data are given in Table 3.
From Table 3 it can be seen that compound I has the smallest experimental value k1. At 200°C k1 increases in the series
and the difference in the k1 values between FOX-7 and compound III is small. The most noticeable increase in the activation energy and, accordingly, the decrease in the rate is observed for compound I. The same conclusion follows from consideration of the calculated data of [6] on the activation energy for the reaction of the transfer of the H atom to the oxygen atom of the nitro group, i.e., for reaction (1). Note that series (e) does not coincide with series (a), (с), or (d), constructed according to the data of [6, 7]. At the same time, sequence (e) seems to most correctly reflect the relationship between stability and the molecular structure. At least it is better correlated than other series with an important structural characteristic of the molecule such as the length of the C=C bond (lC=C).
Reaction (1) is a coordinated process that goes through a six-membered transition state, in which, together with the formation of new bonds, the N–H bond and the π-bond between carbon atoms are simultaneously broken. As shown in [6, 11], the length and, consequently, the strength of the C=C bond strongly depend on the structure of the molecules. In turn, a decrease in the strength of the C=C bond leads to a decrease in the activation energy of the decomposition.
The lC=C bond length in FOX-7 and its analogs has an intermediate value between lC=C in ethylene (1.33 Å) and in ethane (1.54 Å); i.e., the C=C bond length in these compounds is close to one-and-a half. The reason for the lengthening of the C=C bond is the conjugation (overlapping) of the p-orbitals of carbon atoms with an undivided pair of electrons, which occupies one of the sp3-orbitals of nitrogen atoms in both amino and nitro groups. At the same time, the sp3-orbital in the amino group carrying an undivided pair of electrons is located at the top of the trigonal pyramid and is tilted relative to the vertical line of the p-orbitals of the C atoms, which complicates the interaction of the electronic orbitals of the C and N atoms. When the diazacycle is flattened, the conjugation between the amino group and the C=C bond will increase. In the nitro group, the undivided pair of electrons forms a donor-acceptor bond with oxygen atoms. Its orbital spans both oxygen atoms and has the shape of a disk lying in the plane of the nitro group. With a perpendicular arrangement of this plane with respect to the direction of the p-orbitals of carbon atoms, the conjugation of the π-bond with the nitro group is impossible. For this conjugation to occur, it is necessary that the planes of the nitro groups rotate relative to each other or the nitro groups rotate relative to the C=C bond.
According to [11], in 1,1-diiodine-2,2-dinitroethylene, which has a flat structure, lC=C = 1.33 Å; i.e., in this compound, the effect of nitro groups on lC=C is missing. In compound I the nitro groups also practically do not leave the plane perpendicular to the direction of the p-orbitals of carbon atoms. Angle φ, defined as the angle between the CNN planes of the 1,1- and 2,2-substituents, is only 2°, as is the analogous angle in the diiodo derivative. However, due to the conjugation of the double bond with the amino groups, lC=C in compound I is significantly increased. In compounds II and III, nitro groups are fixed in positions that correspond to an increase in the angle φ to 79° and 89°. With such a rotation of the nitro groups, it appears that the conditions are created for their conjugation with the C=C bond. Compared with compound I, lC=C in these compounds increases, although not very strongly. The rotation of nitro groups itself can be stimulated by the formation of strong intramolecular hydrogen bonds [12]. The strength of these bonds depends on the distance between the hydrogen atoms of the amino groups and the oxygen atoms of the nitro groups. The largest distance between H and O and, accordingly, the weakest hydrogen bond are observed in compound I [12].
When decomposing compounds I–III in the solid phase, the order of the change in the reaction rate in their series remains the same as in solution. However, when these compounds are compared with FOX-7, significant differences are observed. The ratio of constants ksol/ks for compounds I–III does not exceed 10, while for FOX-7 it is 100. The decomposition rate of I in a solution is two orders of magnitude lower than in the case of FOX-7, and in the solid state, this difference is reduced to a factor of two. Usually, the activation energy in the solid phase (Es) is equal to or slightly higher than in a liquid (Eliq), and in the case of compound I, vice versa, Es < Eliq. In this case, the experimental error is much smaller than the observed difference in activation energies.
All these anomalies, which appear in the solid phase, are due to the fact that, unlike FOX-7, the condensed products formed during the decomposition of compounds I–III are not solid but liquid (even at room temperature) compounds. Interacting with these products, the original substances themselves turn into a liquid state. In addition, the liquid products exhibit high catalytic activity.
In accordance with the detailed reaction mechanism established in [3], in the decomposition products of compounds I–III, the primary amino compounds of the aliphatic series should appear. Being sufficiently strong bases, these substances can interact with gem-dinitroolefins with the formation of thermally unstable molecular complexes of the donor-acceptor type, the limiting form of which are complexes with charge transfer. It can be expected, therefore, that the catalysis of the decomposition of compounds I –III is acid-base in nature. The absence of autocatalysis in the case of FOX-7 is most likely due to the fact that its decomposition products are solid high-melting compounds, which form free-flowing mixtures with small FOX-7 crystals with a weak contact between particles.
During the decomposition of solid compounds I–III, as in the case of FOX-7, a rapid side process of sublimation and decomposition in the gas phase is observed. When the experiments are carried out in sealed vessels, part of the products of the gas-phase decomposition condenses on the surface of the crystals and suppresses sublimation. The fact that these products are liquid compounds, does not prevent the rapid formation of a protective film. As a result, the side reaction during the decomposition of compounds I–III is suppressed no less effectively than in the case of FOX-7. According to [6], when studying the decomposition of compounds I–III In the mode of linear heating in an argon flow, retardation of the first side stage is not observed. This may be due to the high volatility of the low-melting decomposition products, which are blown away by the argon flow from the crystal surface.
Due to the transition of the substance to a liquid state and the autocatalytic action of the products, the decomposition of the initially solid compounds I–III ends much faster than it does in a solution. When the ratio of the rate constants of the catalytic and initial stages exceeds two orders of magnitude, the contribution of the catalytic decomposition to the reaction rate can become noticeable at a conversion of less than 1%, and at the moment of warming up, the observed decomposition rate can significantly exceed the rate of the initial solid-phase reaction. The latter circumstance may be the reason for the relatively weak retarding effect of the solid phase found for compounds I–III.
After the complete dissolution of the starting material in the products, the decomposition kinetics should follow the laws of liquid-phase autocatalytic reactions with burnout [13]. In these reactions, the catalyst’s concentration additionally increases due to the decrease in the volume of the liquid phase. The maximum decomposition rate in such processes is observed at the end of the reaction, which is observed experimentally for compounds I–III.
CONCLUSIONS
Using the well-known method of carrying out the reaction in a dilute solution of an inert solvent, the values of the rate constants k1 and activation energy of the first, noncatalytic stage of decomposition of compounds I–III, were determined. According to the decomposition mechanism, these compounds do not differ from FOX-7. The rate constant k1 increases in the series I \( \ll \) FOX-7< III ≈ II and is correlated with the increase in the C=C bond length. The latter depends on the degree of conjugation of the C=C double bond with the amino and nitro groups. Compound I has the greatest stability, in which the C=C bond is the strongest.
According to the nature of the decomposition in the solid phase, compounds I–III are significantly different from FOX-7. The decomposition products I–III are liquid compounds (even at room temperature) rather than solid compounds like FOX-7. In addition, these products strongly catalyze the decomposition reaction. Autocatalysis appears at the earliest stages of decomposition, which makes it difficult to measure the true rate constants of decomposition in the solid phase.
REFERENCES
A. J. Bellamy, Struct. Bonding 125, 1 (2007). https://doi.org/10.1007/430_2006_054
U. Ticmanis, M. Kaiser, G. Pantel, et al., in Proceedings of the 35th International Annual Conference ICT (ICT, Karlsruhe, 2004), p. 70/1.
G. M. Nazin, V. V. Dubikhin, T. K. Goncharov, A. I. Kazakov, A. V. Nabatova, and A. V. Shastin, Russ. J. Phys. Chem. B 15, 483 (2021).
G. M. Nazin, V. V. Dubikhin, T. K. Goncharov, A. I. Kazakov, A. V. Nabatova and A. V. Shastin, Russ. J. Phys. Chem. B 15, 604 (2021).
G. M. Nazin, V. V. Dubikhin, T. K. Goncharov, et al., Russ. J. Phys. Chem. B 16 (2022, in press).
B. E. Krisyuk, V. V. Zakarov, N. V. Chukanov, et al., Centr. Eur. J. Energ. Mater. 17 (1), 20 (2020). https://doi.org/10.22211/cejem/118513
N. N. Volkova, A. I. Kazakov, A. V. Danilchik, et al., in New Trends in Research of Energetic Materials, Proceedings of the 21st Seminar (Univ. Pardubice, Czech Republic, 2018), p. 1143.
G. M. Nazin, V. V. Dubikhin, I. L. Dalinger, A. I. Kazakov, and A. V. Nabatova, Russ. J. Phys. Chem. B 15, 74 (2021).
A. V. Shastin, B. L. Korsunskii, and V. P. Lodygina, Russ. J. Appl. Chem. 82, 1802 (2009). https://doi.org/10.1134/S1070427209100103
M.-J. Crawford, J. Evans, M. Cöbel, et al., Propell. Explos. Pyrotech. 32, 478 (2007). https://doi.org/10.1002/prep.200700240
K. Baum, S. S. Bigelow, N. V. Nguyen, et al., Org. Chem. 57, 235 (1992). https://doi.org/10.1021/jo00027a042
B. E. Krisyuk, Russ. J. Phys. Chem. B 14, 1 (2020). https://doi.org/10.1134/S1990793120010054
F. I. Dubovitskii, G. B. Manelis, and A. G. Merzhanov, Dokl. Akad. Nauk SSSR 121, 668 (1958).
Funding
This study was funded by the Institute of Problems of Chemical Physics, Russian Academy of Sciences as part of government assignments (registration numbers АААА-А19-119101690058-9 and АААА-А19-119120690042-9).
Author information
Authors and Affiliations
Corresponding author
Rights and permissions
About this article
Cite this article
Nazin, G.M., Dubikhin, V.V., Kazakov, A.I. et al. Kinetics of the Decomposition of 1,1-Diamino-2,2-Dinitroethylene (FOX-7). Part 4: Comparison of the Decomposition Reactions of FOX-7 and Its Diazacyclic Derivatives. Russ. J. Phys. Chem. B 16, 308–315 (2022). https://doi.org/10.1134/S1990793122020208
Received:
Revised:
Accepted:
Published:
Issue Date:
DOI: https://doi.org/10.1134/S1990793122020208