Abstract
Background
The aim is to assess the levels of fatty acids (FAs) in pregnancies with small for gestational age (SGA) and adequate for gestational age (AGA) fetuses, constituting an association between FAs and fetal growth; according to the role of FA, lower levels were expected in SGA.
Materials and Methods
This was an analytical cross-sectional study including pregnant women with gestational ages of 26–36 weeks with AGA and constitutionally SGA fetuses diagnosed by ultrasonography. The levels of saturated, trans, monounsaturated, and polyunsaturated fatty acids were measured using centrifugation and liquid chromatography. Student’s t test and general linear model using gestational age as covariant were used to compare the levels of FAs and the groups (AGA and SGA). Chi-square was used to evaluate the association between groups and studied variables. Pearson correlation coefficient and linear regression were used to evaluate the correlation between the levels of FAs and gestational age.
Results
Peripheral blood was collected from 67 pregnant women, 3 of whom were excluded from the study. No significant statistical differences were observed between SGA (n = 40) and AGA (n = 24) in relation to saturated, trans, monounsaturated, and polyunsaturated fatty acids (p > 0.05). There was not significant correlation between saturated, trans, monounsaturated, and polyunsaturated FAs and gestational age (p > 0.05).
Conclusion
The levels of saturated, trans, monounsaturated, and polyunsaturated FAs were similar in constitutionally SGA and AGA fetuses.
Similar content being viewed by others
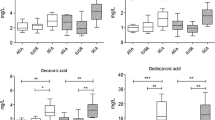
Avoid common mistakes on your manuscript.
Introduction
Fetal growth restriction (FGR) is defined as an estimated fetal weight (EFW) < 10th percentile, which occurs in 5–10% of pregnancies [1] and is the second leading cause of perinatal mortality [2, 3]. There is currently no effective intervention therapy that can reverse, or at least halt, the progressive course of placental insufficiency. Follow-up includes optimizing care and determining the appropriate timing of delivery, weighing the risks inherent to prematurity against those from acidemia resulting from the longer time spent in the uterus [4]. In this context, supplementation with fatty acids (FAs) during pregnancy has been presented as a therapeutic option to promote fetal development and growth.
Omega (ω) 3 is a polyunsaturated carboxylic acid wherein the carbon is linked to the first double bond in position 3. These FAs are produced when fats are broken down and are carboxylic acids with an aliphatic chain. FAs are classified into monounsaturated, polyunsaturated, or saturated [5, 6].
Several benefits of ω-3 polyunsaturated fatty acids include decreasing the levels of triglycerides and LDL cholesterol, increasing the levels of HDL, playing a significant role in allergies and inflammatory processes, increasing immune system protection, decreasing blood pressure and coronary diseases, providing benefits to the skin, and causing improvements in depression cognitive abilities. In the field of obstetrics, it is associated with the prevention of preterm delivery and the promotion of intellectual development [7].
Supplementation of ω-3 in the maternal diet during pregnancy in the animal model reduces oxidative damage to the placenta and increases placental levels of pro-resolution mediators, which are effects associated with fetal growth [8]. However, a systematic review does not support the routine supplementation of ω-3 during gestation as a means of reducing obstetric complications such as FGR [9].
The objective of this study was to assess the blood levels of FAs in pregnant women in constitutionally small for gestational age (SGA) and adequate for gestational age (AGA) fetuses, constituting an association between FAs and fetal growth.
Materials and Methods
This analytical cross-sectional study was conducted between February 2017 and May 2021. This study was approved by the Research Ethics Committee of the Federal University of São Paulo (UNIFESP), and all participants signed an informed consent form. During this period, FAs were analyzed in mother divided into two groups: (1) AGA (control)—estimated fetal weight (EFW) between 10 and 90th percentiles, according to the table proposed by Hadlock et al. [10]; and (2) SGA—EFW < 10th percentile [10] and which do not meet the Delphi criteria [11] for FGR. The Delphi criteria for FGR are the following: EFW < 3rd percentile or EFW between 3rd and 10th percentiles [10] and pulsatility index (PI) of the umbilical artery Doppler > 95th percentile [12] and/or cerebroplacental ratio (CPR) < 1 [13].
Following the diagnosis of SGA, mothers were assessed at the Fetal Growth Restriction Outpatient Clinic of the UNIFESP Obstetrics Department. The inclusion criterion was singleton pregnancy with gestational age of 26–37 weeks and 6 days confirmed by ultrasonography performed up to the 13th week. The exclusion criteria were pregnant women in labor and fetuses with structural malformations.
Women were divided into the following two groups: those with SGA and those with AGA fetuses (control). The following parameters were measured: (1) saturated fatty acids (SFAs): myristic, palmitic, and stearic acids; (2) trans fatty acids (TFAs): elaidic acid; (3) monounsaturated fatty acids: palmitoleic and oleic acids; (4) ω-6 polyunsaturated fatty acids (PUFAs): linoleic, dihomo-gamma linoleic, arachidonic, and gamma-linoleic acids; (5) ω-3 polyunsaturated fatty acids (PUFAs): alpha-linoleic, eicosapentaenoic, and docosahexaenoic acids.
Blood samples were collected, centrifuged, and sent for laboratory analysis within 24 h. Levels of FAs were assessed according to the methodology proposed by Kolarovic and Fournier [14] for sample centrifugation and Agren et al. [15] and Lepage et al. [16] for liquid chromatography analysis.
To evaluate the effect of FGR and AGA fetuses on the levels of FAs, a power analysis was performed to calculate the sample size on the basis of the Cohen effect of 0.7 to achieve a power of 80% and an alpha of 5% to detect the differences in the evaluated parameters [17]. Using the software G 3.1, the results suggested a total sample size of 62 pregnant women.
Data were collected in an Excel 2007 spreadsheet (Microsoft Corp., Redmond, WA, USA) and analyzed using statistical software SPSS version 15.0 (SPSS Inc., Chicago, IL, USA) and Prisma GraphPad version 7.0 (GraphPad Software, San Diego, CA, USA). The non-normal distribution variables were presented as medians and interquartile ranges. The normal distribution variables were presented as mean and standard deviation. Categorical variables were described as absolute and percentage frequencies and represented in tables. To compare the levels of FAs between the groups (AGA and SGA), the Student’s t test and general linear model using gestational age as covariant were used for repetitive measurements. The correlation between the levels of FAs and gestational age was performed using the Pearson correlation coefficient. To study the difference between categorical variables and their proportions, Chi-square test was used. Linear regression was performed to assess the ability of gestational age to predict the level of FAs in maternal blood. The level of significance (p) < 0.05 was applied in all statistical tests.
Results
Peripheral blood samples were collected from 67 mothers of whom three were excluded from the analysis because of blood coagulation (n = 2) and gestational age beyond the time period (n = 1). The results regarding the maternal characteristics such as weight, height, body mass index (BMI), maternal age, and gestational age on the day of maternal blood collection sample are explained in Table 1. There was a significant effect of the studied group on gestational age during the maternal blood sampling collect (p = 0.025). The mean of gestational age was higher in SGA than AGA group (33.0 vs. 31.3 weeks, respectively, p = 0.025) (Table 1).
Table 2 shows the comparison of the levels of saturated, trans, and monounsaturated FAs in SGA and AGA fetuses. There were no statistically significant differences between the two groups (p > 0.05). There was no significant correlation between the levels of saturated, trans, and monounsaturated FAs and gestational age, considering all participants in the study (Fig. 1).
Tables 3 and 4 show the comparison of the levels of ω-6 and -3 PUFAs, respectively, between SGA and AGA fetuses. There were no statistically significant differences between the two groups (p > 0.05). There was no significant correlation between the levels of the levels of ω-6 (Fig. 2) and -3 PUFAs (Fig. 3) and gestational age, considering all participants in the study.
Discussion
The FAs (fatty acids) are absorbed from fetal circulation and stored in fetal adipose tissue. As a result, toward the end of the pregnancy, their levels are several times higher in fetal adipose tissue than in the maternal adipose tissue [18]. FAs are critical for normal fetal growth and development and have several functions, including acting as energy sources and structural components of cell membranes. Chassen et al. [19] have observed that the expression of FAs transport proteins by the placental membrane of FGR fetuses is reduced in relation to AGA fetuses.
The metabolic pathway and the evaluation of the effects of FAs at molecular level are critical key points in the difficult interpretation of the phenomenon occurring at placental site. FAs are desaturated to form arachidonic acid (AA) that cyclooxygenase (COX) enzyme further converts into prostaglandins (PG), thromboxanes (TXs), and leukotrienes while lipoxygenase enzyme synthetize proinflammatory mediators called oxylipins [20, 21]. In turn, PG and TXs may stimulate uterine contractions enhancing the risk of preterm delivery and the risk of FGR [21, 22]. An imbalance between a reduced production of prostacyclin, another AA metabolite and increased level of TXs may cause placental vasoconstriction and ischemia leading to preeclampsia (PE) and secondary FGR [22,23,24].
Because ω-3 and ω-6 PUFAs have antioxidant activity, dietary supplementation of these substances during pregnancy has the potential to prevent or control placental disorders and promote fetal growth [25]. In their experimental study, Jones et al. [26] observed that supplemental ω-3 increased antioxidant defenses in the labyrinthine zone of the placenta. However, it did not prevent the restriction of fetal growth resulting from placental ischemia. In a systematic review involving three randomized clinical trials with 575 single pregnancies in women with previous FGR pregnancies, supplementation with ω-3 did not prevent the recurrence of FGR; however, it was associated with prolongation of pregnancy [27].
Bobiński et al. [28] assessed the maternal diet regarding to AGA, preterm birth and SGA fetuses. The diet components were assessed by dietary questionnaire. The authors conclude that for AGA the predicting factor is a higher content of short and medium chain FA in a woman’s diet. Mani et al. [29] assessed the fat and FA intakes in early pregnancy and birth outcomes in an apparent healthy normal South Indian population. This was a prospective observational cohort with 1838 pregnant women conducted. The results showed that consumption of low saturated FAs was associated with decreased birthweight and an increased risk of SGA fetuses. Similar results were seen with polyunsaturated FA low intakes of α-linolenic and low intakes of long-chain PUFAs were associated with increased risk of SGA.
Smits et al. [30] have demonstrated a higher risk of lower birth weight and SGA in women with low EPA (eicosapentaenoic acid), DHA (docosahexaenoic acid), and DGLA (dihomo-γ-linolenic acid) during early pregnancy while high levels of AA were associated with an increased risk of SGA fetuses (in keeping with the results by Van Eijsden et al. [31]).
Fetal growth is dependent by multiple patterns, such as maternal factors and placental function. Placental function is upregulated by epigenetic processes of DNA methylation and microRNAs (miRNA) that influence gene expression involved in the placental development. It has been postulated that FAs levels (ligand of the peroxisome proliferator-activated receptor (PPAR) may play a role in miRNA regulation of PPAR gene in the placenta of mothers with SGA fetuses [32].
In the present study, although the sample size was small, we did not observe any significant statistical differences in the levels of FAs between women with constitutionally SGA vs. AGA fetuses. This outcome may be partly explained by the fact that in our series, SGA were constitutionally normal, exhibiting an estimated weight < 10th percentile but showing a normal placental function (as documented by absent PI Doppler abnormalities on the umbilical artery) and normal perinatal outcomes [33]. It may be postulated that the absence of differences in FAs levels in SGA fetuses at a time when ischemic hypoxia processes had not already occurred at cellular–molecular level may prevent an unbalance concentration of FAs when assessed on maternal blood.
The limitation of the present study was the use a convenient sampling and size may be too small to draw definitive conclusions. Other limitation, we did not assess a group with FGR which shows different grades of placental insufficiency which could interfere in the maternal–fetal placental transport of FAs. As strengths, the level of ω-3 and ω-6 PUFAs was similar in pregnant women of both SGA and AGA and the pregnant women with SGA did not show deficient of ω-3 and ω-6 PUFAs.
Further studies are needed to better define if maternal supplementation in FAs during pregnancy will result in beneficial effects on placental function thus preventing FGR and other complications such as preterm delivery. In summary, the levels of saturated, trans, monounsaturated, and polyunsaturated FAs were similar between constitutionally SGA and AGA fetuses.
References
Frøen JF, Gardosi JO, Thurmann A, Francis A, Stray-Pedersen B. Restricted fetal growth in sudden intrauterine unexplained death. Acta Obstet Gynecol Scand. 2004;83(9):801–7.
Barker DJ, Gluckman PD, Godfrey KM, Harding JE, Owens JA, Robinson JS. Fetal nutrition and cardiovascular disease in adult life. Lancet. 1993;341(8850):938–41.
Mandruzzato GP, Bogatti P, Fisher L, Gigli C. The clinical significance of absent or reverse end diastolic flow in the fetal aorta and umbilical artery. Ultrasound Obstet Gynecol. 1991;1(3):192–6.
Lees C, Marlow N, Arabin B, et al. Perinatal morbidity and mortality in early-onset fetal growth restriction: cohort outcomes of the trial of randomized umbilical and fetal flow in Europe (TRUFFLE). Ultrasound Obstet Gynecol. 2013;42(4):400–8.
Waitzberg DL, Garla P. Contribution of omega-3 fatty acids for memory and cognitive function. Nutr Hosp. 2014;30(3):467–77.
Agostoni C, Marangoni F, Stival G, et al. Whole blood fatty acid composition differs in term versus mildly preterm infants: small versus matched appropriate for gestational age. Pediatr Res. 2008;64(3):298–302.
Brantsæter AL, Birgisdottir BE, Meltzer HM, et al. Maternal seafood consumption and infant birth weight, length and head circumference in the Norwegian Mother and Child Cohort Study. Br J Nutr. 2012;107(3):436–44.
Jones ML, Mark PJ, Waddell BJ. Maternal dietary omega-3 fatty acids and placental function. Reproduction. 2014;147(5):R143–52.
Saccone G, Saccone I, Berghella V. Omega-3 long-chain polyunsaturated fatty acids and fish oil supplementation during pregnancy: which evidence? J Matern Fetal Neonatal Med. 2016;29(15):2389–97.
Hadlock FP, Harrist RB, Martinez-Poyer J. In utero analysis of fetal growth: a sonographic weight standard. Radiology. 1991;181(1):129–33.
Gordijn SJ, Beune IM, Thilaganathan B, et al. Consensus definition of fetal growth restriction: a Delphi procedure. Ultrasound Obstet Gynecol. 2016;48(3):333–9.
Arduini D, Rizzo G. Normal values of pulsatility index from fetal vessels: a cross-sectional study on 1556 healthy fetuses. J Perinat Med. 1990;18(3):165–72.
Arias F. Accuracy of the middle-cerebral-to-umbilical-artery resistance index ratio in the prediction of neonatal outcome in patients at high risk for fetal and neonatal complications. Am J Obstet Gynecol. 1994;171(6):1541–5.
Kolarovic L, Fournier NC. A comparison of extraction methods for the isolation of phospholipids from biological sources. Anal Biochem. 1986;156(1):244–50.
Agren JJ, Julkunen A, Penttila I. Rapid separation of serum lipids for fatty acid analysis by a single aminopropyl column. J Lipid Res. 1992;33(12):1871–6.
Lepage G, Levy E, Ronco N, et al. Direct transesterification of plasma fatty acids for the diagnosis of essential fatty acid deficiency in cystic fibrosis. J Lipid Res. 1989;30(10):1483–90.
Cohen J. Statistical power analysis for the behavioral sciences. New York: Routledge Academic; 1988.
Bobiński R, Mikulska M. The ins and outs of maternal-fetal fatty acid metabolism. Acta Biochim Pol. 2015;62(3):499–507.
Chassen SS, Ferchaud-Roucher V, Gupta MB, et al. Alterations in placental long chain polyunsaturated fatty acid metabolism in human intrauterine growth restriction. Clin Sci (Lond). 2018;132(5):595–607.
Innes JK, Calder PC. Omega-6 fatty acids and inflammation. Prostaglandins Leukot Essent Fat Acids. 2018;132:41–8.
Szczuko M, Kikut J, Komorniak N, et al. The role of arachidonic and linoleic acid derivatives in pathological pregnancies and the human reproduction process. Int J Mol Sci. 2020;21(24):9628.
Vrachnis N, Karavolos S, Iliodromiti Z, et al. Impact of mediators present in amniotic fluid on preterm labour. In Vivo. 2012;26(5):799–812.
Nadeau-Vallée M, Obari D, Palacios J, et al. Sterile inflammation and pregnancy complications: a review. Reproduction. 2016;152(6):R277–92.
Walsh SW. Eicosanoids in preeclampsia. Prostaglandins Leukot Essent Fat Acids. 2004;70:223–32.
Larqué E, Gil-Sánchez A, Prieto-Sánchez MT, et al. Omega 3 fatty acids, gestation and pregnancy outcomes. Br J Nutr. 2012;107(Suppl 2):S77-84.
Jones ML, Mark PJ, Waddell BJ. Maternal omega-3 fatty acid intake increases placental labyrinthine antioxidant capacity but does not protect against fetal growth restriction induced by placental ischaemia-reperfusion injury. Reproduction. 2013;146(6):539–47.
Saccone G, Berghella V, Maruotti GM, et al. Omega-3 supplementation during pregnancy to prevent recurrent intrauterine growth restriction: systematic review and meta-analysis of randomized controlled trials. Ultrasound Obstet Gynecol. 2015;46(6):659–64.
Bobiński R, Mikulska M, Mojska H, et al. Assessment of the diet components of pregnant women as predictors of risk of preterm birth and born baby with low birth weight. Ginekol Pol. 2015;86(4):292–9.
Mani I, Dwarkanath P, Thomas T, et al. Maternal fat and fatty acid intake and birth outcomes in a South Indian population. Int J Epidemiol. 2016;45(2):523–31.
Smits JM, Elzenga HM, Reinoud Gemke JBJ, et al. The association between interpregnancy interval and birth weight: what is the role of maternal polyunsaturated fatty acid status? BMC Pregnancy Childbirth. 2013;13:23.
Van Eijsden M, Hornstra G, Wal MF, et al. Maternal n-3, n-6, and trans fatty acid profile early in pregnancy and term birth weight: a prospective cohort study. Am J Clin Nutr. 2008;87(4):887–95.
Sundrani DP, Karkhanis AR, Joshi SR. Peroxisome Proliferator-Activated Receptors (PPAR), fatty acids and microRNAs: Implications in women delivering low birth weight babies. Syst Biol Reprod Med. 2021;67(1):24–41.
Figueras F, Gratacós E. Update on the diagnosis and classification of fetal growth restriction and proposal of a stage-based management protocol. Fetal Diagn Ther. 2014;36(2):86–98.
Funding
This study was not funded.
Author information
Authors and Affiliations
Corresponding author
Ethics declarations
Conflicts of interest
The authors declare no conflict of interest.
Ethical Approval
This study was approved by the Research Ethics Committee of the Federal University of São Paulo (UNIFESP). All procedures performed in studies involving human participants were in accordance with the ethical standards of the institutional and/or national research committee and with the 1964 Helsinki declaration and its later amendments or comparable ethical standards.
Informed Consent
Informed consent was obtained from all individual participants included in the study.
Additional information
Publisher's Note
Springer Nature remains neutral with regard to jurisdictional claims in published maps and institutional affiliations.
Raquel Margiotte Grohmann is a Medical Assistant Isabela César Corazza - Gynecologist / Obstetrician Alberto. Borges Peixoto is an Adjunct Professor. Vivian Macedo Gomes Marçal is a Gynecologist / Obstetrician. Edward Araujo Júnior is an Associate Professor. Gabriele Tonni is a Full Professor Luciano Marcondes Machado Nardozza.
Rights and permissions
About this article
Cite this article
Grohmann, R.M., Corazza, I.C., Peixoto, A.B. et al. Maternal Blood Fatty Acid Levels in Small and Adequate for Gestational Age Pregnancies. J Obstet Gynecol India 72 (Suppl 1), 217–223 (2022). https://doi.org/10.1007/s13224-022-01632-z
Received:
Accepted:
Published:
Issue Date:
DOI: https://doi.org/10.1007/s13224-022-01632-z