Abstract
This study aimed to assess the impact of humic acid application on three safflower genotypes (AC-Sterling, Arack 2811, and C111) cultivated in cadmium contaminated soil with varying levels of salinity (0, 50, 100, and 150 mM NaCl). The experiment showed that the salinity decreased the K/Na ratio, photosynthesis rate, chlorophyll content, root volume as well as shoot and root dry weight, while it increased the cadmium concentration and the activities of catalase, peroxidase and ascorbate peroxidase. Of particular interest, the C111 genotype demonstrated the lowest decline in K/Na ratio and photosynthesis and exhibited the least increase in cadmium absorption. Furthermore, the salinity-induced increase in the antioxidant activity was most pronounced in catalase. The humic acid application led to increased activities of antioxidant enzymes, K/Na ratio, photosynthesis rate, chlorophyll content, root volume, shoot dry weight, and root dry weight. The humic acid application, however, resulted in decreased cadmium concentration in Arak 2811 while it increased cadmium concentration in AC-Sterling and C111. This indicates that the positive effects of humic acid on biomass were more pronounced in the C111 genotype under lower salinity levels and mostly manifested in root growth rather than shoot growth. The superiority of C111 in terms of the response to humic acid was more attributed to catalase and peroxidase activation compared to ascorbate peroxidase. Overall, the results showed a significant variation among safflower genotypes regarding the plant physiological attributes and the cadmium content in response to the salinity and the humic acid application.
Similar content being viewed by others
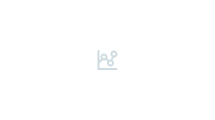
Explore related subjects
Discover the latest articles, news and stories from top researchers in related subjects.Avoid common mistakes on your manuscript.
Introduction
Soil salinity is one of the most serious abiotic stresses restricting crop growth and production (Parihar et al. 2015). While salinization can transpire across all climate zones, it is more common in arid regions (Bacilio et al. 2016). When exposed to heightened salt levels, plants undergo diverse physiological and biochemical reactions (Salem et al. 2014). Nonetheless, the tolerance to salt stress varies significantly among various plant species (Munns and Tester 2008), which could be categorized into tissue resistance and ion depletion (Aliyari Rad et al. 2021).
Soil physical properties may be affected by salinity, causing the deterioration of soil structure and reductions in soil hydraulic conductivity and infiltration rate. It also accelerates surface runoff and soil erosion (Edelstein et al. 2010) and affects soil microorganisms negatively (Yan et al. 2015). Furthermore, as the concentration of salt rises in the tissues, plants experience two main phases of stress: osmotic stress and specific ion (Na+ and Cl−) toxicity (Arzani and Ashraf 2016). These primary issues can trigger secondary stresses like oxidative stress and nutritional imbalances. Consequently, plants may suffer from impaired growth due to disruptions in metabolic and physiological functions. These disruptions include reduced nutrient and water uptake, membrane dysfunction, and disturbances in critical processes such as respiration, photosynthesis, and protein synthesis (Arzani et al. 2023).
Cadmium (Cd) is a toxic and highly mobile heavy metal that penetrates the environment from anthropogenic activities, particularly the use of phosphorous fertilizers containing Cd (Shafi et al. 2010; Khan et al. 2017). Moreover, using biosolids containing heavy metals such as Cd in order to improve saline soils quality in arid and semi-arid regions can also result in soil pollution (Mühling and Läuchli 2003). The toxicity of Cd has carcinogenic effects on various human organs like the kidneys, lungs, heart, and bones (Roberts 2014). Plants absorb Cd through the cortex tissue in their roots, which are then transported to aerial organs via the xylem transport system, alongside water and both macro- and micronutrients from the soil. Ultimately, these elements are stored in various parts of the plant, including leaves, fruits, and grains (Nayeri et al. 2023). Cadmium toxicity in plants leads to a range of detrimental effects, including chlorosis, necrosis, and wilting. It impairs photosynthesis, reduces chlorophyll content and decreases the transpiration rate. Additionally, cadmium exposure results in a decrease in xylem vessel density and leaf relative water content. Furthermore, the toxicity disrupts carbohydrate metabolism, inhibits stomatal opening, diminishes plasma membrane activity, and alters enzyme activities (Dias et al. 2013; Nayeri et al. 2023). To mitigate the harmful effects of heavy metals such as Cd, some plant species have evolved a range of defensive strategies. These adaptations include mechanisms for metal sequestration, compartmentalization within specific cell organelles, exclusion, and inactivation through the release of organic ligands (Nayeri et al. 2023). The extent to which plants uptake Cd depends on the soil Cd concentration, soil pH, soil mineralogy, soil organic matter, and the existence of other metals (Filipović et al. 2018). In addition, it has been shown that the Cd concentration in crops may be increased in saline soils fertilized by phosphorous fertilizers containing Cd (Huang et al. 2007). The combined effect of salinity and Cd stress on plants could cause membrane leakage and a rise in the production of oxygen radicals and H2O2 (Mühling and Läuchli 2003).
Soil organic materials (OM) play a crucial role in enhancing soil fertility via the chemical, physiological, and biological reactions in the soil (Khaled and Fawy 2011). Adding OM provides energy and nutrients for soil microorganisms to grow and function in poor soil conditions (Novair et al. 2024). One of the main soil OMs is humic acid (HA), which is extracted from plant and animal residues decomposition (Fan et al. 2014). Studies on different plants have shown that HA helps them directly and indirectly to grow better, and that the effects vary among plant types (Hemati et al. 2022). HA enriches plant growth indirectly by improving soil properties such as reducing soil compaction, water holding capacity, soil microbial activity, and availability of micro/macro elements. It also enriches the plant growth directly by increasing plant biomass in terms of the uptake of HA substances as well as transporting them to plant tissues (Ampong et al. 2022; Tahir et al. 2011; Hemati et al. 2022). Various studies indicate that the humification process leads to the formation of complex compounds between humic materials and mineral ions. This interaction enhances enzyme activity, resulting in increased respiration intensity, improved photosynthesis, and greater metabolism of nucleic acids (Hemati et al. 2022). In addition, the humic substances containing acidic electrolytes with carboxylic and phenolic hydroxyl (OH) functional groups may reduce the heavy metals toxicity by providing macromolecules that affect the bioavailability and solubility of heavy metals (Khan et al. 2017). Numerous studies have investigated the interactions between salinity stress and humic acid, however, there is a significant gap in the literature concerning the specific responses of safflower cultivars in a cadmium-contaminated soil. Therefore, the present experiment aimed to examine the effect of HA application on alleviating Cd-polluted soil and salinity stress in three safflower genotypes.
Materials and methods
Growth condition
Six seeds of three safflower genotypes, AC-sterling, Arak 111, and C111, were sown in 5-L plastic pots (20.5 cm diameter and 22.5 cm depth). These pots were filled with 5 kg of cadmium-contaminated soil derived from an area surrounding a steel plant in Isfahan, Iran. This soil was classified as a silty loam soil containing 0.99% organic matter, 131 mg kg−1 available K by 1 N acetate, 5.5 mg kg−1 total Cd, 0.85 available Cd by DTPA extraction method; having pH of 8.01 and EC of 0.51 dS−1 in saturated extract. Humic acid (from Biomega company) was applied to the soil before sowing at a rate of control and 1g kg−1 pot soil.
The emerged plants were initially watered with tap water (EC = 1.9 dS m−1) until they were fully established when they had 4 to 6 true leaves. Three weeks after emergence, the seedlings were then irrigated with saline water at varying concentrations. Prior to full establishment, the plants were irrigated every 3 days. Once established, they were irrigated as needed (once or twice a week) until they reached the time of applying salinity treatments at four-leaf stage. To minimize the risk of osmotic shock, the seedlings were gradually exposed to increasing levels of NaCl, beginning with a concentration of 25 mM until reaching final concentrations of either 50, 100, or 150 mM NaCl. The plants were irrigated after 50% of the available soil water had been depleted. Plants in all treatments received the same volume of irrigation water. The volume of irrigation water was calculated using the following formula:
Where Virrig is the irrigation water volume (cm3), θFC is the gravimetric water content (%) at field capacity, θavg the average moisture of soil layer in the root zone, ρb the apparent density of the soil, and Vpot the volume of the pot.
The pots were placed outdoors at the Isfahan University of Technology in Isfahan, Iran to expose them to natural environmental conditions.
Plants were harvested about 60 days after applying salinity treatment, at the beginning of flowering stage. Following the harvest of the shoots, the roots were carefully extracted from the soil and rinsed in a basin of water to remove any remaining soil particles. Subsequently, the roots and aerial organs were washed with distilled water then were dried separately at 65 °C for 72 h before measuring their dry weights.
Ion concentration analysis
The Na and K concentrations were measured using Hamada and El-Enany (1994) method. Each plant’s powdered samples, weighing 0.2 g, were thoroughly burned in an electric oven. Subsequently, 10 mL of HCl 2 N was added to each sample while gently heated. The resultant solution was sieved through Whatman filter paper and the volume was then brought up to 100 mL using distilled water. The flame photometer (model Jenway PFP7 UK) was employed to measure the Na+ and K+ contents from 1:100 diluted root and shoot samples.
For the determination of Cd accumulation in plant tissues, the samples were extracted by using 4 mL of Merck HNO3, kept undisturbed for 24 h, heated in a laboratory oven, and determined by Perkin Elmer 3030 USA atomic absorption spectrophotometer.
Photosynthesis rate
The net photosynthetic rate was measured on three healthy leaves from each experimental unit using a calibrated portable gas exchange system (LCi, ADC Bioscientific Ltd., UK).
Measurement of photosynthetic pigments
Chlorophyll (Chl) contents of safflower genotypes leaves were determined following Lichtenthaler and Wellburn’s method (1983). Leaf tissues chlorophyll was extracted with 10 mL 80% acetone and light absorbance was recorded at 645 and 663 nm by Hitachi U-1800 spectrophotometer for Chl a and Chl b, respectively.
Root volume
The root volume was measured using the water displacement method. First, the roots were submerged in a graduated cylinder filled with water. The water levels in the cylinder were recorded before and after submerging the roots into the cylinder. The volume of roots was calculated as follows:
Determination of antioxidant enzymes activity
To determine the antioxidant enzyme activities (Peroxidase (POX), Catalase (CAT), and Ascorbate peroxidase (APX)), 0.1 g of frozen leaf samples were grounded with liquid nitrogen and homogenized with 1mL of 100mM K2PO4 containing (pH = 7) 0.1 mM Merck Ethylenediaminetetraacetic acid (EDTA) and 1% Merck polyvinyl-pyrrolidone (PVP) on ice. The solution was centrifuged by Hettich Benchtop centrifuge UNIVERSAL 320 at 1400 rpm for 30 min under 4 °C. The supernatant was used for the determination of antioxidant enzyme activities.
The CAT activity was measured using a spectrophotometer (Hitachi U-1800) at 240 nm, following the method described by Aebi (1984), using 3mL of a reaction solution containing 50 mM Na2PO4 (pH = 7), 10 mM H2O2 and 40 μL of the enzyme extract.
The APX activity was determined by the Nakano and Asada (1981) method using 1 mL of a reaction buffer containing 50 mM K2PO4 (pH = 7), 0.5 mM ascorbic acid, 0.1 mM EDTA, 1.25 mM H2O2, and 60 μL of the enzyme extract, at 290 nm in 1 min.
The determination of POX activity followed a procedure similar to that used for CAT, except that the extraction buffer for peroxidase contained an additional 3.35 μL of GUAIACOL per cuvette, in addition to 4.51 μL of H2O2 (30%). Absorbance was measured at 470 nm over a duration of 2 min (Change and Maehly 1955).
Statistical methods
The experiment was set up in a completely randomized design with a factorial arrangement of treatments (3 species, 2 HA levels, 4 NaCl levels, and 3 replications). Analysis of variance was carried out using SAS. Results were analyzed in terms of variance and LSD (at p ≤ 0.050) values were calculated for cultivars, treatments, and their interactions.
Results
Cadmium concentration in shoot and roots
The effects of the interactions between salinity and genotype and HA and genotype were significant on the concentration of Cd in shoot and roots (Table 1). Salinity increased the concentration of Cd in shoot and roots in all tested genotypes (Table 2). At 50, 100, and 150 mM NaCl compared to non-saline control, Cd concentration in the shoot was increased by 27.8, 48.8 and 68.4% in AC-Sterling, 23.1, 39.0, and 67.6 in Arak 2811 and 12.0, 19.4, and 27.3 in C111 genotype, respectively.
The corresponding values for root Cd concentration were 16.2, 35.3, and 62.4 in AC-Sterling, 23.6, 44.7, and 67.1 in Arak 2811 and 12.9, 26.7, and 43.3 in C111 genotype.
The application of HA increased the concentration of Cd in shoot and root by 15.2 and 24.1% in AC-Sterling and by 10.1 and 11.4% in C111, respectively, but it decreased Cd concentration by 16 and 16.6% in Arak 2811 genotype (Table 3).
The effects of the interactions between salinity and cultivar, as well as between HA and genotype, were significant on shoot/root Cd concentration (Table 1).
K/Na ratio in shoot and roots
The effect of the three-way interaction of salinity, cultivar, and HA and cultivar was significant on the K/Na ratio in shoot and roots (Table 1). Salinity decreased the K/Na ratio in shoot and roots of HA applied and non-applied plants of all tested genotypes (Table 4). At 50, 100, and 150 mM NaCl compared to non-saline control, the K/Na ratio in shoots was decreased by 76.9, 91.7, and 96.8% in AC-Sterling, 69.9, 89.8, and 97.1% in Arak 2811 and 63.6, 76.3, and 92.9% in C111 genotype, respectively, under none HA applied control and by 79.5, 94.1, and 97.7% in AC-Sterling, 60.9, 87.9, and 95.0% in Arak 2811 and 45.6, 77.9, and 92.0% in C111, respectively, under HA application.
The corresponding reduction in the K/Na ratio in roots due to salinity were 30.0, 55.4, and 77.8% in AC-Sterling, 54.0, 73.8, and 91.8% in Arak 2811, and 52.5, 75.2, and 90.9% in C111 genotype, respectively, under none HA applied control and by 58.9, 80.8, and 86.6% in AC-Sterling, 55.1, 79.9, and 91.6% in Arak 2811, and 51.9, 76.0, and 82.0% in C111, respectively, under HA application.
The application of HA increased the K/Na ratio in shoots and roots of all tested genotypes under all salinity levels. At 0, 50, 100, and 150 mM NaCl the ratio of K/Na in shoot was increased by 99.3, 77.4, 41.8, and 43.3% in AC-Sterling, 48.6, 92.6, 74.5, and 154% in Arak 2811, and 75.2, 162, 63.2, and 98.2% in C111, respectively.
The corresponding values in the K/Na ratio in roots were 233, 95.8, 43.5, and 101 in AC-Sterling, 64.0, 59.9, 26.1, and 67.8% in Arak 2811, and 42.4, 44.0, 37.8, and 116% in C111, respectively.
Photosynthesis rate
The effect of the three-way interaction of salinity, cultivar, and HA was significant on the photosynthesis rate (Table 1). Salinity decreased the photosynthesis rate of both HA applied and non-applied plants of all tested genotypes (Table 4). At 50, 100, and 150 mM NaCl compared to non-saline control, the photosynthesis rate was decreased by 27.0, 52.1, and 65.8% in AC-Sterling, 35.1, 51.1, and 64.2% in Arak 2811, and 18.1, 45.4 and 66.2% in C111 genotype, respectively, under none HA applied control and by 29.4, 50.8, and 65.9% in AC-Sterling, 47.7, 66.2, and 71.7% in Arak 2811, and 21.3, 40.3 and 67.2% in C111, respectively, under HA application.
The application of HA increased the photosynthesis rate in shoot and roots of all tested genotypes under all salinity levels (Table 4). At 0, 50, 100, and 150 mM NaCl photosynthesis rate was increased due to HA application by 27.7, 23.5, 30.9, and 27.2% in AC-Sterling, 60.8, 29.5, 11.3, and 26.9% in Arak 2811 and 22.1, 17.3, 33.4, and 18.7% in C111, respectively.
Chlorophyll content
The effects of the interactions between salinity and cultivar, HA and cultivar as well as salinity and HA were significant on leaf chlorophyll content (Table 1). Salinity decreased the content of chlorophyll in all tested genotypes (Table 2). At 50, 100, and 150 mM NaCl salinity levels compared to non-saline control, the content of chlorophyll was decreased by 21.8, 45.0, and 66.2% in AC-Sterling, 41.3, 53.1, and 59.7% in Arak 2811, and 25.8, 38.4, and 65.9% in C111, respectively.
Regardless of the salinity level, the application of HA increased the concentration of chlorophyll by 16.5, 9.2, and 48% in AC-Sterling, Arak 2811, and C111 cultivars, respectively (Table 3). On the other hand, irrespective of cultivar, HA application increased chlorophyll content by 22.8, 26.2, 20.3, and 26.5%, respectively, under 0, 50, 100, and 150 mM NaCl salinity levels (Table 5).
Root volume
The effect of the three-way interaction of salinity, cultivar and HA was significant on root volume (Table 6). Salinity decreased root volume of both HA applied and non-applied plants of all tested genotypes (Table 4). At 50, 100, and 150 mM NaCl compared to non-saline control, root volume was decreased by 72.7, 76.3, and 89.1% in AC-Sterling, 47.1, 57.2, and 61.4% in Arak 2811 and 37.5, 65.5, and 83.7% in C111 genotype, respectively, under none HA applied control and by 66.7, 78.3, and 91.1% in AC-Sterling, 16.7, 37.8, and 68.9% in Arak 2811, and 33.3, 63.3, and 70.0% in C111, respectively, under HA application.
The application of HA increased the root volume of all tested genotypes under all salinity levels. At 0, 50, 100, and 150 mM NaCl root volume was increased due to HA application by 63.6, 100, 49.8, and 33.0% in AC-Sterling, 28.5, 103, 86.6, and 3.8% in Arak 2811, and 11.1, 20.0, 10.0, and 107% in C111, respectively.
The activities of antioxidant enzymes
The effect of the three-way interaction of salinity, cultivar and HA was significant on the activities of CAT and POX (Table 6). Salinity increased CAT activity in both HA applied and non-applied plants of all tested genotypes (Table 4). At 50, 100, and 150 mM NaCl compared to non-saline control, CAT activity was increased by 46.0, 75.0, and 201% in AC-Sterling, 101, 267, and 417% in Arak 2811, and 32.8, 62.4, and 213% in C111 genotype, respectively, under none HA applied control and by 93.6, 306 and 543% in AC-Sterling, 53.8, 212 and 351% in Arak 2811, and 17.8, 64.3, and 138% in C111, respectively, under HA application. The corresponding increases in POX activity due to salinity were by 22.0, 55.3, and 108% in AC-Sterling, 7.10, 16.9, and 24.8% in Arak 2811, and 27.4, 55.2, and 129% in C111 genotype, respectively, under none HA applied control and by 156, 223 and, 313% in AC-Sterling, 48.1, 75.9 and 193% in Arak 2811, and 30.4, 51.9, and 87.4% in C111, respectively, under HA application (Table 4).
The application of HA increased the activities of CAT and POX in all tested genotypes under all salinity levels. At 0, 50, 100, and 150 mM NaCl CAT activity was increased due to HA application by 14.8, 52.1, 167 and 145% in AC-Sterling, 85.9, 42.2, 58.1, and 61.9% in Arak 2811, and 196, 163, 200, and 125% in C111, respectively and POX activity was increased by 116, 354, 349, and 330% in AC-Sterling, 83.4, 153, 176, and 330% in Arak 2811, and 421, 433, 410, and 326% in C111, respectively.
The effects of the interactions between salinity and cultivar and HA and cultivar were significant on the activity of APX (Table 6). Salinity increased APX activity in all tested genotypes (Table 2). At 50, 100 and 150 mM NaCl compared to non-saline control, APX activity increased by 12.3, 27.7 and 99.4% in AC-Sterling, 37.1, 57.1, and 77.6% in Arak 2811, and 22.8, 40.9, and 71.7% in C111 genotype, respectively.
Regardless of salinity level, the application of HA increased the activity of APX on average by 15.5, 43.5, and 42.0% in AC-Sterling, Arak 2811, and C111.
Shoot and root weights
The effects of the interactions between salinity and HA and also cultivar and HA were significant on shoot weight and the effects of the interactions between salinity and HA were significant on root weight (Table 6). Salinity, regardless of cultivar, decreased shoot and root weights. The significant interactions between salinity and HA showed that at 50, 100, and 150 mM NaCl compared to non-saline control, shoot weight was decreased by 23.4, 36.2, and 49.7% under non-applied HA and by 23.4, 40.4, and 51.6% under HA application (Table 5). The corresponding reductions in root weight were 32.2, 50.8, and 71.2% under non-applied HA and 33.1, 51.7, and 68.9% under HA application.
Humic acid application, regardless of cultivar, increased shoot dry weight by 20.9, 20.8, 12.9, and 16.4%, while it increased the root dry weight by 28.0, 26.3, 25.9, and 38.2% under 0, 50, 100, and 150 mM NaCl salinity treatments.
The significant interactions between cultivar and HA showed that regardless of salinity level, the application of HA increased shoot weight on average by 13.4, 14.0, and 28.0% in AC-Sterling, Arak 2811, and C111 (Table 3).
Although the interactions between salinity and cultivar were not statistically significant on shoot weight, the reduction in shoot weight at 50, 100, and 150 mM NaCl compared to non-saline control were 23.5, 37.1, and 48.6% in AC-Sterling, 22.8, 40.6, and 53.0% in Arak 2811, and 21.7, 34.4, and 49.9% in C111.
Discussion
This study demonstrates that the concentration of Cd in the shoots and roots of all tested genotypes was elevated due to increased salinity. Cd phytoextraction increase by soil salinization has also been reported in tobacco and maize (López-Chuken et al. 2012), wheat (Abbas et al. 2018), bean (Rady 2011), castor, and mustard (Bauddh and Singh 2012). Studies have shown that soil salinity may increase the mobility and phyto-availability of heavy metals such as Cd by forming chloric complexes and even by altering root system functions (Ashrafi et al. 2015; Filipović et al. 2018). In fact, the increment of chloride ions in Cd-contaminated soils alters free Cd2+ to Cd-Cl complexes in soil solution, and as a result, thereby facilitating the transfer of Cd from the soil to the plant (Filipović et al. 2018).
However, the safflower genotypes exhibited variations in shoot Cd concentration and also their increased Cd concentration under saline conditions. AC-Sterling displayed a lower shoot Cd concentration (22.6 mg/kg DW) compared to Arak 2811 (24.1 mg) and C111 (26.0 mg). Interestingly, the extent of Cd accumulation in response to salinity was smaller in C111 (19.6%) in contrast to AC-Sterling (48.2%) and Arak 2811 (43.2%; an average of 50, 100, and 150 mM NaCl). The absorption and translocation of Cd in plants and the tolerance to Cd toxicity differ among plant species, and even different genotypes of the same species (Pourghasemian et al. 2013) depending on the genes and gene networks that involve in physiological and molecular mechanisms (Benavides et al. 2005). Studies on wheat (Lu et al. 2021), maize (Wu et al. 2023), cotton (Wu et al. 2004), rapeseed (Mwamba et al. 2020), sunflower (Tan et al. 2023), and safflower (Pourghasemian et al. 2013; Pourghasemian et al. 2019) demonstrates the variation in Cd uptake and tolerance among plant genotypes.
The interaction effect of salinity × cultivar was not statistically significant, indicating that the reduction in shoot weight followed similar trends across the three cultivars when exposed to varying NaCl concentrations. Additionally, C111 exhibited lower reductions in K/Na ratio (74.7%) and photosynthesis rate (43.1%) due to salinity when compared to AC-Sterling (89.5% and 48.5%, respectively) and Arak 2811 (83.4% and 56.0%). These findings may indicate that C111 is a more tolerant genotype when it exposes to salt stress. Studies indicate that the accumulation of salt in the soil causes plant growth reduction by osmotic stress, ion toxicity, and unbalancing mineral uptake and translocation (Hussain et al. 2016). In line with our findings, genotypic variation in salinity tolerance has been reported in safflower by other researchers (Yeilaghi et al. 2015; Thoday-Kennedy et al. 2021; Badran et al. 2022). For example, a study on 200 safflower genotypes showed at least four different genotypic salt tolerance classes for safflower in which the salt tolerance in safflower genotypes depended on Na+ entry prevention to shoots and K+ uptake preservation (Thoday-Kennedy et al. 2021).
In the current study, the impact of salinity stress on antioxidant enzymes was examined, revealing an elevation in their activities. Remarkably, among the enzymes studied, catalase (CAT) demonstrated the highest increase as compared to POX and APX. All biotic and abiotic stresses in plants alter the metabolism of reactive oxygen species (ROS), and as a result, to detoxify aggressive ROS, antioxidant enzymes activities such as superoxide dismutase, ascorbate peroxidase, and catalase would be increased (Munns and Tester 2008; Chaki et al. 2020). Despite the less negative salinity effects on physiological properties of the C111 genotype, it showed less increase in antioxidant enzymes activity under different saline conditions than the other two genotypes. It seems that this genotype may possess alternative mechanisms for tolerating salinity.
This study also illustrates that HA application improved nutritional status, growth attributes, and the activities of antioxidant enzymes in all tested genotypes. Several studies have shown that HA’s hormone-like activity and chelating capacity affect plant functions and development (Ampong et al. 2022). Macro and micro nutrients, plant height, leaf area, dry matter, photosynthetic efficiency, protein content, antioxidant enzymes activity, and plant yield enhancement due to HA application have been observed in wheat, rice, maize, faba bean, pea and rapeseed (Abou-Aly and Mady 2009; García et al. 2012; Jannin et al. 2012; Osman and Rady 2012; Radwan et al. 2015; Lotfi et al. 2018; Dawood et al. 2019; Liu et al. 2019). Plant morphological and physiological properties are affected by HA due to its structural characteristics (Tavares et al. 2021).
The application of HA resulted in significantly greater increases in shoot weight in C111 (28%) compared to AC-Sterling (13.4%) and Arak 2811 (14%). Additionally, C111 showed a greater increase in chlorophyll content (48%) compared to AC-Sterling (16.5%) and Arak 2811 (9.2%) due to the application of HA. Furthermore, the increases in shoot weight were accompanied by higher increases in CAT and POX in C111 (159.3% and 384.8% respectively) compared to AC-Sterling (113.6% and 302.6%) and Arak 2811 (59.4% and 203.3%). These findings suggest that while all three antioxidant enzymes played a role in improving safflower biomass production through HA application, CAT and POX had a much greater influence. Besides, the stimulating activities of these two enzymes had an important role in the superiority of C111 in response to HA application in terms of biomass production. Some other studies illustrated the different effects of HA on wheat (Abbas et al. 2022), maize (Shahryari et al. 2011), and sorghum (Kandil et al. 2017) genotypes.
The positive effects of HA application were found to be even more pronounced on roots, with a significant increase of 29.6%, compared to a slightly lower increase of 17.8% on shoots. This may clarify the improvement of plant nutritional status under HA application. These results are in agreement with those of Rosa et al. (2018), which similarly observed a more noticeable impact of HA on wheat roots.
HA application had greater positive effects on biomass production under no-saline and low salinity treatments. Different results were obtained by (Jarošová et al. 2016), as in their study the application of HA had a greater positive effect on biomass production and growth attributes of barley under higher salinity levels. These incompatible results may be described by different HA levels and/or by different genotypes used in the two experiments. Nevertheless, in the current study, the larger effects of HA on roots under higher salinity levels were well-matched with the results reported by others.
Conclusion
Variation, in this study, existed among safflower genotypes in terms of Cd concentration and the response to salinity and HA application. Among the three genotypes, C111 stood out as superior in terms of having lower increases in Cd levels and less decrease in K/Na ratio and photosynthesis under salinity stress. Additionally, C111 exhibited the most positive response to HA application. The activities of antioxidant enzymes CAT, POX, and APX provided a significant role in differentiation responses of safflower genotypes to both salinity and HA application. Notably, HA application proved to be more effective in promoting biomass production under lower salinity levels.
Data availability
Data will be made available on request.
Abbreviations
- APX:
-
Ascorbate peroxidase
- CAT:
-
Catalase
- Cd:
-
Cadmium
- Chl:
-
Chlorophyll
- EDTA:
-
Ethylenediaminetetraacetic acid
- HA:
-
Humic acid
- OM:
-
Organic materials
- PVP:
-
Polyvinyl-pyrrolidone
References
Abbas T, Rizwan M, Ali S, Adrees M, Zia-ur-Rehman M, Qayyum MF, Ok YS, Murtaza G (2018) Effect of biochar on alleviation of cadmium toxicity in wheat (Triticum aestivum L.) grown on Cd-contaminated saline soil. Environ Sci Pollut Res 25:25668–25680
Abbas G, Rehman S, Siddiqui MH, Ali HM, Farooq MA, Chen Y (2022) Potassium and humic acid synergistically increase salt tolerance and nutrient uptake in contrasting wheat genotypes through ionic homeostasis and activation of antioxidant enzymes. Plants 11:263
Abou-Aly HE, Mady MA (2009) Complemented effect of humic acid and biofertilizers on wheat (Triticum aestivum L.) productivity. Annals Agric Sci Moshtohor 47:1–12
Aebi H (1984) Catalase in vitro. Methods Enzymol 105:121–126
Aliyari Rad S, Dehghanian Z, Asgari Lajayer B, Nobaharan K, Astatkie T (2021) Mitochondrial respiration and energy production under some abiotic stresses. J Plant Growth Regul 41:3285–3299
Ampong K, Thilakaranthna MS, Gorim LY (2022) Understanding the role of humic acids on crop performance and soil health. Front Agron 4:848621
Arzani A, Ashraf M (2016) Smart engineering of genetic resources for enhanced salinity tolerance in crop plants. Crit Rev Plant Sci 35:146–189
Arzani A, Kumar S, Mansour MMF (2023) Salt tolerance in plants: molecular and functional adaptations. Front Plant Sci 14:1280788
Ashrafi A, Zahedi M, Soleimani M (2015) Effect of co-planted purslane (Portulaca Oleracea L.) on Cd accumulation by sunflower in different levels of Cd contamination and salinity: a pot study. Int J Phytoremediation 17:853–860
Bacilio M, Moreno M, Bashan Y (2016) Mitigation of negative effects of progressive soil salinity gradients by application of humic acids and inoculation with Pseudomonas stutzeri in a salt-tolerant and a salt-susceptible pepper. Appl Soil Ecol 107:394–404
Badran AE, Khalil RM, Mostafa E (2022) Genetic evaluation of some safflower genotypes under salinity stress. J Plant Mol Breed 10:12–21
Bauddh K, Singh RP (2012) Growth, tolerance efficiency and phytoremediation potential of Ricinus communis (L.) and Brassica juncea (L.) in salinity and drought affected cadmium contaminated soil. Ecotoxicol Environ Saf 85:13–22
Benavides MP, Gallego SM, Tomaro ML (2005) Cadmium toxicity in plants. Braz J Plant Physiol 17:21–34
Chaki M, Begara-Morales JC, Barroso JB (2020) Oxidative stress in plants. Antioxidants 9:481
Change B, Maehly AC (1955) Assay of catalases and peroxidases. Methods Enzymol 2:764–775
Dawood MG, Abdel-Baky YR, El-Awadi MES, Bakhoum GS (2019) Enhancement quality and quantity of faba bean plants grown under sandy soil conditions by nicotinamide and/or humic acid application. Bull Natl Res Cent 43:1–8
Dias MC, Monteiro C, Moutinho-Pereira J, Correia C, Gonçalves B, Santos C (2013) Cadmium toxicity affects photosynthesis and plant growth at different levels. Acta Physiol Plant 35:1281–1289
Edelstein M, Ben-Hur M, Plaut Z (2010) Water salinity and sodicity effects on soil structure and hydraulic properties. Adv Hort Sci 24:154–160
Fan HM, Wang XW, Sun X, Li YY, Sun XZ, Zheng CS (2014) Effects of humic acid derived from sediments on growth, photosynthesis and chloroplast ultrastructure in chrysanthemum. Sci Hortic 177:118–123
Filipović L, Romić M, Romić D, Filipović V, Ondrašek G (2018) Organic matter and salinity modify cadmium soil (phyto) availability. Ecotoxicol Environ Saf 147:824–831
García AC, Santos LA, Izquierdo FG, Sperandio MVL, Castro RN, Berbara RLL (2012) Vermicompost humic acids as an ecological pathway to protect rice plant against oxidative stress. Ecol Eng 47:203–208
Hamada AM, El-Enany AE (1994) Effect of NaCl salinity on growth, pigment and mineral element contents, and gas exchange of broad bean and pea plants. Biol Plant 36:75–81
Hemati A, Alikhani HA, Ajdanian L, Babaei M, Asgari Lajayer B, van Hullebusch ED (2022) Effect of different enriched vermicomposts, humic acid extract and indole-3-acetic acid amendments on the growth of Brassica napus. Plants 11:227
Huang YZ, Wei K, Yang J, Dai F, Zhang GP (2007) Interaction of salinity and cadmium stresses on mineral nutrients, sodium, and cadmium accumulation in four barley genotypes. J Zhejiang Univ Sci B 8:476–485
Hussain MI, Lyra DA, Farooq M, Nikoloudakis N, Khalid N (2016) Salt and drought stresses in safflower: a review. Agron Sustain Dev 36:1–31
Jannin L, Arkoun M, Ourry A, Laîné P, Goux D, Garnica M, Fuentes M, Francisco SS, Baigorri R, Cruz F, Houdusse F (2012) Microarray analysis of humic acid effects on Brassica napus growth: involvement of N, C and S metabolisms. Plant Soil 359:297–319
Jarošová M, Klejdus B, Kováčik J, Babula P, Hedbavny J (2016) Humic acid protects barley against salinity. Acta Physiol Plant 38:1–9
Kandil AA, Sharief AE, El Badry DE (2017) Seedling parameters as affected by soaking in humic acid, salinity stress and grain sorghum genotypes. Int J Agric Environ Biotechnol 2:3213–3223
Khaled H, Fawy HA (2011) Effect of different levels of humic acids on the nutrient content, plant growth, and soil properties under conditions of salinity. Soil Water Res 6:21–29
Khan KY, Ali B, Cui X, Feng Y, Stoffella PJ, Tang L, Yang X (2017) Effect of humic acid amendment on cadmium bioavailability and accumulation by pak choi (Brassica rapa ssp. chinensis L.) to alleviate dietary toxicity risk. Arch Agron Soil Sci 63:1431–1442
Lichtenthaler HK, Wellburn AR (1983) Determinations of total carotenoids and chlorophylls a and b of leaf extracts in different solvents. Biochem Soc Trans 11:591–592
Liu M, Wang C, Wang F, Xie Y (2019) Maize (Zea mays) growth and nutrient uptake following integrated improvement of vermicompost and humic acid fertilizer on coastal saline soil. Appl Soil Ecol 142:147–154
López-Chuken UJ, López-Domínguez U, Parra-Saldivar R, Moreno-Jiménez E, Hinojosa-Reyes L, Guzmán-Mar JL, Olivares-Sáenz E (2012) Implications of chloride-enhanced cadmium uptake in saline agriculture: modeling cadmium uptake by maize and tobacco. Int J Environ Sci Technol 9:69–77
Lotfi R, Kalaji HM, Valizadeh GR, Khalilvand Behrozyar E, Hemati A, Gharavi-Kochebagh P, Ghassemi A (2018) Effects of humic acid on photosynthetic efficiency of rapeseed plants growing under different watering conditions. Photosynthetica 56:962–970
Lu M, Yu S, Lian J, Wang Q, He Z, Feng Y, Yang X (2021) Physiological and metabolomics responses of two wheat (Triticum aestivum L.) genotypes differing in grain cadmium accumulation. Sci Total Environ 769:145345
Mühling KH, Läuchli A (2003) Interaction of NaCl and Cd stress on compartmentation pattern of cations, antioxidant enzymes and proteins in leaves of two wheat genotypes differing in salt tolerance. Plant Soil 253:219–231
Munns R, Tester M (2008) Mechanisms of salinity tolerance. Annu Rev Plant Biol 59:651–681
Mwamba TM, Islam F, Ali B, Lwalaba JLW, Gill RA, Zhang F, Farooq MA, Ali S, Ulhassan Z, Huang Q, Zhou W (2020) Comparative metabolomic responses of low-and high-cadmium accumulating genotypes reveal the cadmium adaptive mechanism in Brassica napus. Chemosphere 250:126308
Nakano Y, Asada K (1981) Hydrogen peroxide is scavenged by ascorbate-specific peroxidase in spinach chloroplasts. Plant Cell Physiol 22:867–880
Nayeri S, Dehghanian Z, Lajayer BA, Thomson A, Astatkie T, Price GW (2023) CRISPR/Cas9-Mediated genetically edited ornamental and aromatic plants: a promising technology in phytoremediation of heavy metals. J Clean Prod 428:139512
Novair SB, Hosseini HM, Etesami H, Pirmoradian N, Lajayer BA, Price GW (2024) Straw application improved soil biological properties and the growth of rice plant under low water irrigation. Environ Res 255:119138
Osman AS, Rady MM (2012) Ameliorative effects of sulphur and humic acid on the growth, anti-oxidant levels, and yields of pea (Pisum sativum L.) plants grown in reclaimed saline soil. Hortic Sci Biotechnol 87:626–632
Parihar P, Singh S, Singh R, Singh VP, Prasad SM (2015) Effect of salinity stress on plants and its tolerance strategies: a review. Environ Sci Pollut Res 22:4056–4075
Pourghasemian N, Ehsanzadeh P, Greger M (2013) Genotypic variation in safflower (Carthamus spp.) cadmium accumulation and tolerance affected by temperature and cadmium levels. Environ Exp Bot 87:218–226
Pourghasemian N, Landberg T, Ehsanzadeh P, Greger M (2019) Different response to Cd stress in domesticated and wild safflower (Carthamus spp.). Ecotoxicol Environ Saf 171:321–328
Radwan FI, Gomaa MA, Rehab IF, Samera IA (2015) Impact of humic acid application, foliar micronutrients and biofertilization on growth, productivity and quality of wheat (Triticum aestivum L.). Middle East J Agric Res 4:130–140
Rady MM (2011) Effect of 24-epibrassinolide on growth, yield, antioxidant system and cadmium content of bean (Phaseolus vulgaris L.) plants under salinity and cadmium stress. Sci Hort 129:232–237
Roberts TL (2014) Cadmium and phosphorous fertilizers: the issues and the science. Procedia Eng 83:52–59
Rosa SD, Silva CA, Maluf HJGM (2018) Wheat nutrition and growth as affected by humic acid-phosphate interaction. J Plant Nutr Soil Sci 181:870–877
Salem N, Msaada K, Dhifi W, Limam F, Marzouk B (2014) Effect of salinity on plant growth and biological activities of Carthamus tinctorius L. extracts at two flowering stages. Acta Physiol Plant 36:433–445
Shafi M, Zhang G, Bakht J, Khan MA, Islam UE, Khan MD, Raziuddin GZ (2010) Effect of cadmium and salinity stresses on root morphology of wheat. Pak J Bot 42:2747–2754
Shahryari R, Khayatnezhad M, Moghanlou BS, Khaneghah AM, Gholamin R (2011) Response of maize genotypes to changes in chlorophyll content at presence of two types humic substances. Adv Environ Biol 5:154–156
Tahir MM, Khurshid M, Khan MZ, Abbasi MK, Kazmi MH (2011) Lignite-derived humic acid effect on growth of wheat plants in different soils. Pedosphere 21:124–131
Tan D, Zhang L, Zhang S, Cui B (2023) Physiological response of four widely cultivated sunflower cultivars to cadmium stress. Horticulturae 9:320
Tavares OCH, Santos LA, Filho DF, Ferreira LM, García AC, Castro TAVT, Zonta E, Pereira MG, Fernandes MS (2021) Response surface modeling of humic acid stimulation of the rice (Oryza sativa L.) root system. Arch Agron Soil Sci 67:1046–1059
Thoday-Kennedy E, Joshi S, Daetwyler HD, Hayden M, Hudson D, Spangenberg G, Kant S (2021) Digital phenotyping to delineate salinity response in safflower genotypes. Front Plant Sci 12:662498
Wu F, Wu H, Zhang G, Bachir DM (2004) Differences in growth and yield in response to cadmium toxicity in cotton genotypes. J Plant Nutr Soil Sci 167:85–90
Wu Y, An T, Gao Y, Kuang Q, Liu S, Liang L, Xu B, Zhang S, Deng X, Chen Y (2023) Genotypic variation in the tolerance to moderate cadmium toxicity among 20 maize genotypes with contrasting root systems. J Sci Food Agric 103:2618–2630
Yan N, Marschner P, Cao W, Zuo C, Qin W (2015) Influence of salinity and water content on soil microorganisms. Int Soil Water Conserv Res 3:316–323
Yeilaghi H, Arzani A, Ghaderian M (2015) Evaluating the contribution of ionic and agronomic components toward salinity tolerance in safflower. Agron J 107:2205–2212
Acknowledgements
The authors would like to express their gratitude to the Isfahan University of Technology for providing the necessary facilities to conduct this research. We also thank Firoozeh Fayaz for her technical help and advice.
Funding
The research leading to these results received funding from Isfahan University of Technology (IUT), Isfahan, Iran.
Author information
Authors and Affiliations
Contributions
Conceptualization and Supervision, Morteza Zahedi; Material preparation, data collection and analysis: Nahid Amoozad, Writing—original draft, Nahid Amoozad; Writing—review and editing, Morteza Zahedi.
Corresponding author
Ethics declarations
Conflict of interest
The authors report there are no competing interests to declare.
Additional information
Publisher's Note
Springer Nature remains neutral with regard to jurisdictional claims in published maps and institutional affiliations.
Rights and permissions
Springer Nature or its licensor (e.g. a society or other partner) holds exclusive rights to this article under a publishing agreement with the author(s) or other rightsholder(s); author self-archiving of the accepted manuscript version of this article is solely governed by the terms of such publishing agreement and applicable law.
About this article
Cite this article
Amoozad, N., Zahedi, M. Effects of humic acid application on physiological and biochemical characteristics of safflower cultivars under salinity and cadmium contamination. J. Crop Sci. Biotechnol. (2024). https://doi.org/10.1007/s12892-024-00258-z
Accepted:
Published:
DOI: https://doi.org/10.1007/s12892-024-00258-z