Abstract
The aim of this study was to evaluate the probiotic characteristics and safety of seven Enterococcus faecalis isolates from fecal samples of healthy Chinese infants. We evaluated the isolates’ tolerance to low pH, survival in bile salts and NaCl, adhesion ability, biofilm formation, antimicrobial activity, toxin gene distribution, hemolysis, gelatinase activity, antibiotic resistance, and virulence to Galleria mellonella. All strains survived at pH 5.0, in 7.0% NaCl, and in 3% bile salt. Adhesion to Caco-2 cells was above 10%. Strain A3-1 had higher adhesion ability toward mucin, collagen, and BSA in vitro, better antibacterial activity, and the strongest biofilm production. We detected seven virulence genes with a distribution of asa1 (100%), cylA (71.4%), esp (85.7%), hyl (14.3%), gelE (85.7%), ace (42.9%), and agg (71.4%). Although all strains were γ-hemolytic, none showed gelatinase activity based on physiological activity detection. All isolates were susceptible to benzylpenicillin, ampicillin, ciprofloxacin, levofloxacin, moxifloxacin, tigecycline, nitrofurantoin, linezolid, and vancomycin; they were not susceptible to erythromycin, quinupristin/dalofopine, and clindamycin. The virulence test of G. mellonella showed that, except for strains 106-1 and 113-1, the other strains had toxicity lower than 10%. Strain A3-1 may have the greatest potential to be developed as a probiotic.
Similar content being viewed by others
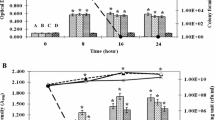
Avoid common mistakes on your manuscript.
Introduction
Enterococci are Gram-positive, normal flora of human and animal gastrointestinal tracts. They belong to the class of facultative anaerobic lactic acid bacteria (LAB). Enterococci are distributed widely and can be isolated from the environment, food, and human and animal gastrointestinal tracts [1, 2]. Enterococci have probiotic properties, such as high resistance to acids and bile salts [3,4,5], improving immunity [2], antioxidant and free radical scavenging activity [5], intestinal adhesion and localization [6, 7], enhancing apoptosis of human cancer cells [8], antibacterial activity [8, 9], and regulating inflammation [10]. Because of these special properties, enterococci are used in supplementation of animal feed to prevent disease, relieve animal diarrhea symptoms, stimulation of immune system to and improve animal intestinal microbiota [1, 2, 11]. Importantly, as probiotics, enterococci need to reach and colonize the host intestines so that their resistance and adhesion functions will have a competitive advantage.
According to FAO/WHO, probiotic microorganisms must be non-pathogenic and non-toxic and should be alive with sufficient amount in the target niche where it exerts the beneficial effect [12]. Some enterococci are also opportunistic pathogens and potential infectious agents. Mainly due to the presence of antibiotic resistance and virulence factors, enterococci can cause infection and diseases, usually nosocomial infections such as endocarditis and urinary tract infection [13, 14]. Interestingly, biofilm formation in enterococci enhances their tolerance to antibiotics and also contributes in genetic exchange and dissemination of resistance properties [12]. The antibiotic resistance and the virulence factors of clinical isolates may increase the difficulty of treating enterococcus infections [15]. However, we cannot completely eliminate the possibility of enterococcus infection by food transmission, with consequent potential hazards to public health [1, 16, 17]. In addition, the antibiotic resistance genes of enterococci may be horizontally transferred and acquired [18]. Pathogenic enterococci may cause concerns about the safe using of probiotics, so to screen the potential probiotic enterococci, assessing and evaluating the safety is necessary and priority. Potential probiotic enterococci isolates can be applied to biotechnology development, and broader applications can be obtained by strain improvement. The aim of this study was to evaluate the probiotic characteristics and safety of seven Ent. faecalis strains isolated from fecal samples of healthy infants and to evaluate the potential of these isolates for probiotic development and application.
Material and Methods
Bacterial Strains and Cell Culture
Enterococcus faecalis strains were isolated from fecal samples by the Microbiology Lab of the Nutrition and Food Safety Engineering Research Center of Shaanxi province, Xi’an, China. Fecal samples were collected from 12 healthy infants from the Maternal and Child Health Hospital of Bin County of Shaanxi province, China. All infants were 1-7 days old, and they had no history of antimicrobial treatment after birth. Mothers were made aware of the nature of the study, and they provided personal information. This study was reviewed and approved by the Ethics Committee of the Health Science Center, Xi’an Jiaotong University, Xi’an, China (No.2016-114).
Ent. faecalis strains were cultured in MRS medium (CM187, Beijing Land Bridge Technology Co., Ltd., Beijing, China), and incubated aerobically at 37 °C for 18-24 h. For the streak-plating growth, Ent. faecalis strains were inoculated at MRS agar used (MRS broth with 15 g/L agar) and incubated for 18 h. Lactobacillus rhamnosus GG BL379 were cultured in MRS broth (without shaking) or MRS agar medium at 37 °C for 18-24 h.
Caco-2 cells were grown in Dulbecco’s modified Eagle’s minimal essential medium (DMEM, Hyclone) supplemented with 10% fetal bovine serum (FBS, Hyclone), 10,000 U/ml penicillin, and 10,000 μg/ml streptomycin (Pen-Strep, Gibco). The cells were kept at 37 °C in an atmosphere containing 5% CO2.
Isolation and Identification of Enterococcus Isolates
Ent. faecalis strains were isolated by the streak-plate method on MRS agar (MRS broth with 15 g/L agar) and incubated at 37 °C for 18 h. Single colonies were picked out for the Gram staining and microscopic observation, and catalase, oxidase production, and nitrate reduction tests. For further confirmation, 16S rRNA gene sequence (1.4 kb) was amplified and sequenced by Sangon Biotech Co., Ltd. (Shanghai, China). Primers used were 16S-27F: 5′AGAGTTTGATCCTGGCTCAG3′, 16S-1492R: 5′GGTTACCTTGTTACGACTT3′. Multiple alignments with sequences of most close similarity were analyzed using CLUSTAL W, and a phylogenetic tree was constructed by using the neighbor-joining method.
Acid, NaCl, and Bile Salt Tolerance
Ent. faecalis strains were inoculated into MRS broth and incubated at 37 °C overnight. The cells were washed with sterilized 0.01 M phosphate buffer saline (PBS, pH 7.2) and re-suspended in fresh MRS broth supplemented separately with different concentrations of bile salts (0.5%, 1%, 2%, 3%) and NaCl (1.75%, 3.5%, 7%); MRS medium at pH 3, 4, and 5 was also used (pH of MRS broth was adjusted by HCl to pH 3, 4, 5 separately before sterilization). Growth was monitored by optical density at 600 nm every 30 min at 37 °C for 20 h in a microtiter plate reader (PolarStar, BMG Labtech, GER) [4]. At least three independent replicates of each growth curve were obtained. Maximal growth, the lag phase duration, and the increment in OD values were considered by using the Gompertz growth analysis mode of non-linear regression in GraphPad Prism 5.
In Vitro Binding to BSA, Mucin, and Collagen
All strains were assayed for binding to different substrates immobilized on 96-well immunoplates [19]. Plates were covered with the different substrates overnight at 4 °C. Mucin (500 μg/ml, porcine stomach, Sigma-Aldrich, St. Louis, MO, USA), BSA (500 μg/ml, Sigma-Aldrich, St. Louis, MO, USA), and collagen (50 μg/ml, type I, Roche, Mannheim, GER) were added in the 96-well MaxiSorp plates (black, Nunc). After immobilization, wells were washed three times with PBS (0.01 M, pH 7.2) and dried at room temperature. Then, 100 μl of each strain was labeled by addition of 3.5 mg/ml cFDA (5-(6-)-carboxyfluorescein diacetate, Sigma-Aldrich, St. Louis, MO, USA) to the plate wells. The cFDA-labeled Enterococcus cells were incubated separately with the immobilized mucin, BSA, and collagen at 4 °C overnight and kept away from light. Non-adhered cells were removed by washing three times with PBS and dried at room temperature; then, 100 μl of PBS was added to each well. The 100 μl cFDA-labeled Ent. faecalis cells were added into the well without any immobilized substrates, and used as control. Fluorescence intensity of the well plate was measured using a microplate reader, and the degree of adhesion of Enterococcus cells to mucin, collagen, and BSA was calculated according to the following formula.
Adhesion (%) = (fluorescence intensity of tested wells)/(the free cFDA-labeled Enterococcus cells) × 100
Lactobacillus rhamnosus GG BL379 was used as a positive control; its percent adhesion was set as 100%, and the relative adhesion of other Ent. faecalis strains to BL379 was calculated.
Adhesion Ability to Caco-2 Cells
Adhesion ability to Caco-2 was evaluated as reported previously [8, 10]. Briefly, high-glucose DMEM, supplemented with 10% FBS, was used to culture the cells. Caco-2 cells were seeded in 96-well plates and incubated at 37 °C until confluent. Overnight bacterial cultures were washed in PBS twice and re-suspended in 1ml cell culture medium (without antibiotic) at a final concentration of 107 CFU/ml. The 100 μl of this bacterial suspension was added into Caco-2 culture plate wells and incubated at 37 °C for 2 h; the Caco-2 cells were washed three times with PBS to remove the un-adhering bacteria, and then 50 μl 0.25% Trypsin-EDTA was added to lyse those cells. Finally, the mixture of each well was plated on MRS agar plates to count the adhered bacteria. The adhesion (%) = ((CFU/ml) adhered bacteria/(CFU/ml) added bacteria) × 100.
Quantitative Assessment of Biofilm Formation
It was carried out as described previously by Zhang et al. [20]. Shortly, Ent. faecalis cells were collected from fresh overnight cultures, washed twice with sterilized PBS, and adjusted to OD600 = 1. Then, 50 μl of cell suspension and 150μl fresh MRS broth were transferred to sterile 96-well plates; 200 μl MRS broth without cells served as negative control. The 96-well plates were incubated for 24 h at 37 °C. After incubation, the content of each well was gently removed, and the wells were washed three times with PBS to remove free-floating planktonic bacteria, then air dried. The biofilm forming bacterial cells were then fixed with 200 μl methanol for 15 min and stained with 200 μl of 1% crystal violet for 15 min. The wells were washed three times with sterile water and rinsed thoroughly with 200 μl ethanol. Optical density of stained adherent cells was determined at 595 nm with an automatic microplate reader (Tecan Infinite M200, CHE). Experiments for each strain were performed in triplicate. The cut-off OD (ODC) was defined as three standard deviations above the mean OD of the negative control. The ability to form biofilm was determined as follows: OD ≤ ODC as non-biofilm-producer (0), ODC < OD ≤ 2ODC as weak biofilm producer (+), 2ODC < OD ≤ 4ODC as moderate biofilm producer (++), and OD > 4ODC as strong biofilm producer (+++).
Antimicrobial Activity
Antimicrobial activity was determined by the minimum inhibitory concentration (MIC) method. The antimicrobial activity was tested with the following strains: Enterococcus faecalis ATCC29212, Staphylococcus aureus ATCC25923, Enterobacter hormaechei ATCC700323, Pseudomonas aeruginosa ATCC27853, Escherichia coli ATCC35218, Salmonella typhimurium ATCC14028, Pseudomonas aeruginosa PA01. Ent. faecalis was inoculated into MRS broth and incubated at 37 °C for 24 h. The cells were removed by centrifugation at 9710g for 2 min at 4 °C. The supernatant was filter sterilized. The indicator bacteria were inoculated in MRS broth (Ent. faecalis ATCC29212) or LB broth (remaining tested bacteria) overnight, and the cells were collected by centrifugation at 9710g for 2 min at 4 °C, washed twice with sterile PBS, and re-suspended in sterile water. The Ent. faecalis supernatants of each isolate were added to 96-well plates at 0, 25, 50, 100, 150, and 200 μl, made up to 200 μl with fresh medium. Then, each test pathogens was added to the well at a final concentration of OD600 = 0.1. Growth of test pathogens was monitored every 30 min by optical density at 600 nm with an automatic microplate reader for 12 h at 37 °C.
Antibiotic Susceptibility
The antimicrobial susceptibility of the Ent. faecalis strains was accessed using a VITEK 2 Compact with AST-GP67 (REF 22226, bioMererieux, FRA), which included benzylpenicillin (BPEN, 0.125–64 μg/ml), ampicillin (AMP, 0.5–32 μg/ml), high-level gentamicin (synergistic) (HLG, 500 μg/ml; GEN, 8–64 μg/ml), high-level streptomycin (HLS, 1000 μg/ml), ciprofloxacin (CIP, 1–4 μg/ml), levofloxacin (LVX, 0.25–8 μg/ml), moxifloxacin (MXF, 0.25–8 μg/ml), erythromycin (ERY, 0.25–2 μg/ml), clindamycin (CLI, 0.15–2 μg/ml), quinupristin/dalofopine (QDA, 0.25–2 μg/ml), linezolid (LZD, 0.15–2 μg/ml), vancomycin (VAN, 1–16 μg/ml), tetracycline (TET, 0.15–2 μg/ml), tigecycline (TGC, 0.25–1 μg/ml), and nitrofurantoin (NIT, 16–64 μg/ml). Quality control for Vitek 2 Compact was performed as detailed in the manufacturer’s handbook [21]. The interpretation of susceptibility data was performed using Advanced Expert System following the Clinical Laboratory Standard Institute criteria (CLSI M100 S27).
Hemolysis
All the enterococcus strains were inoculated in MRS broth overnight at 37 °C to reach the stationary growth stage. Hemolytic activity was tested by incubating bacteria on Columbia agar supplemented with 5% (v/v) sheep blood [22]. After incubating for 48 h at 37 °C, hemolysis was classified as α-hemolysis, β-hemolysis, and γ-hemolysis. The α-hemolysis or β-hemolysis indicated positive hemolysis activity; the γ-hemolysis was taken as negative hemolysis activity. The overnight culture was then diluted to an OD600 of 1.0 with cold sterilized PBS, and the supernatants were collected by filter sterilization. A 100-μl aliquot of supernatant was gently added into a tube and mixed with 850 μl of PBS and 50-μl sheep red blood cells, then incubated at 37 °C for 30 min. The mixtures were centrifuged at 800g for 1 min, and the optical density of the supernatant was measured at 543 nm. Sterile water was used as positive control, and PBS was used as negative control. The hemolysis activity was calculated as (%) = (OD543 of sample − OD543 of negative control)/(OD543 of positive control − OD543 of negative control). Staphylococcus aureus ATCC25923 was used as a reference strains, and all tests were triplicated.
Gelatinase Activity
Overnight culture of Ent. faecalis was inoculated (1%, piercing 5 mm below the surface of medium) into gelatin medium (120 g/l gelatin, 5 g/l peptone, 3 g/l beef extract, pH 6.8 ± 0.2) and incubated at 37 °C for 48 h. The tubes were then placed at 4 °C for 1 h, and if there was liquid in the tube, it meant the gelatin had been hydrolyzed by expressed gelatinase.
Detection of Virulence Genes
Enterococci genomic DNA was extracted using a bacterial genomic DNA extraction kit (Omega Bio-Tek, Doeaville, GA, USA). All strains were investigated by PCR for the presence of virulence genes involved in expression of gelatinase (gelE), cytolysin (cylA), hyaluronidase (hyl), aggregation substance (asa1, agg), enterococcal surface protein (esp), endocarditis antigen (efaA), and collagen adhesion (ace, acm). The PCR primers are listed in Table 1. And the procedure of PCR included 35 cycles of denaturation at 94 °C for 4 min, annealing at 58-48 °C for 30 s (touch-down PCR), and elongation at 72 °C for 30 s at Veriti 96W Thermal cycler (ABI, Foster, CA, USA). PCR products were analyzed by 1.5% agarose gel electrophoresis, stained with ethidium bromide, and visualized under UV light [23].
Virulence Activity Assay by Galleria mellonella
The test in wax moth (Galleria mellonella) larvae was used to access virulence [24]. Larvae weighing about 300 mg (purchased from Tianjin Huiyude Biotech Company, Tianjin, China) were maintained on woodchips in the dark at 15 °C. Overnight cultures of Ent. faecalis were adjusted to 1 × 108 CFU/ml and 1 × 109 CFU/ml. Ten randomly selected insects weighing 250–350 mg were used for each isolate. Larvae were inoculated with bacterial suspension (1 × 106 CFU/larva and 1 × 107 CFU/larva) via the rear left proleg using a 10 μl Hamilton animal syringe. MRS medium and saline were injected as negative controls. The insects were cultured at 37 °C every 12 h for 3 days, and survival was recorded.
Statistical Analysis
Statistical analyses were performed using the t test and analysis of variance (ANOVA), JMP pro (SAS Institute Inc., NC, USA), STAMP10, and SPSS V20.0 (IBM Inc., IL, USA). Data were presented as means ± SEM. Significant differences were considered at P < 0.05.
Results
Characterization and Identification of Isolates
We selected seven Gram-positive and cocci-shaped bacteria from 12 fecal samples. Their colonies (0.5-1 μm in diameter) had a sticky, translucent, white, and mucoid appearance on MRS agar. Identification of Ent. faecalis isolates was carried out by observation of colony characteristics and cell morphology, Gram staining (G+), catalase (negative) and oxidase production (negative), and nitrate reduction test (negative), and they were confirmed by 16S rRNA sequence analysis. They were grown aerobically and anaerobically from 25 to 45 °C; the optimal temperature was 37 °C. The strains were identified as Ent. faecalis by 16S rRNA gene sequencing. The strain sequences had the following NCBI accession numbers: A3-1 (MH385351), A9-1 (MH385354), 67-2 (MH385355), 106-1 (MH385347), 107-1 (MH385348), 113-1 (MH385349), and 117-1 (MH385350). Comparison by BLAST analysis of the 16S rDNA gene sequences with other bacteria from GenBank showed that the closest strains (99% similarity) were Ent. faecalis. The N-J tree (Fig. 1) also grouped those strains into the Ent. faecalis cluster.
The analysis of the phylogeny of the isolated Enterococcus strains alignment of the 16S rDNA sequences was performed with the sequences of different Enterococcus and Lactobacillus species which were submitted in NCBI database. The NCBI accession number of Enterococcus faecalis ATCC 19433 (NR_115765.1), Enterococcus faecalis DSM20478T (LN681572.1), Enterococcus saccharolyticus ATCC43076 (NR_041707.1), Enterococcus faecium ATCC 19434 (NR_115764.1), Enterococcus durans CECT411T (AJ420801.1), Enterococcus durans DSM20633 (AJ276354.1), Bacillus mycoides ATCC6462 (NR_115993.1), Lactobacillus brevis ATCC14869 (NR_044704.2), Streptococcus thermophilus ATCC 19258 (NR_042778.1)
Acid, NaCl, and Bile Salt Tolerance
We used a stimulated gastric juice (pH 3, 4, 5) to assess the acid tolerance of enterococcus isolates (Supplementary material 1). The seven strains survived at pH 5.0 in MRS medium, and the maximum cell density reached about 50% of the density in normal MRS. Except for strain 67-2, the other six stains showed great tolerance to bile salt and NaCl; they grew to the same density as the control (P > 0.05). In addition, 3% bile salt stimulated growth of strain 117-1, and 7% NaCl stimulated growth of strains 107-1, 113-1, and 117-1 (Table 2).
Antimicrobial Activity
The seven Ent. faecalis isolates showed inhibitory activity against the indicator strains (Fig. 2). The supernatants of strains had different antimicrobial activities to the indicator microorganism. Compared with Gram-positive bacteria, the Ent. faecalis isolates had stronger antibacterial activity against Gram-negative bacteria. Interestingly, the supernatant of strain 67-2 lacked antibacterial activity to S. aureus ATCC25923, and strain 106-1 lacked antibacterial activity to Ent. faecalis ATCC29212.
Adhesion Ability
We determined the adhesion capacity of the bacteria to the human colon carcinoma cell line Caco-2 (Fig. 3a). Adhesion capacity varied significantly, ranging from 54.9 to 10.6%. The highest adherence capacity belonged to isolates A9-1 (54.9%) and 106-1 (48.9%); strain 67-2 had the lowest adherence (10.6%).
In the host intestinal tract, the surfaces of intestinal epithelial cells are covered with a layer of mucus composed of glycoproteins such as collagen and mucin. These glycoproteins can be used as an in vitro interaction model to assess the adhesion of probiotics. Compared with L. rhamnosus GG BL379 (positive control), strains A3-1, 107-1, 113-1, and 117-1 showed higher adhesion to mucin (P < 0.05). Strains A3-1, A9-1, 67-2, 107-1, 113-1, and 117-1 showed higher adhesion to collagen (P < 0.05); strains A3-1, 113-1, and 117-1 had 11.2-, 2.3-, and 3.3-fold higher collagen binding ability compared with the control. Only strain A3-1 showed higher adhesion to BSA (P < 0.01) (Fig. 3b).
Distribution of Virulence Genes and Production of Hemolysin, Gelatinase, and Biofilm
We detected seven genes encoding known virulence factors in the enterococcus isolates: asa1 (100%), cylA (71.4%), esp (85.7%), hyl (14.3%), gelE (85.7%), ace (42.9%), and agg (71.4%), and there had no detection of acm and efaA. Interestingly, six gelE-positive strains did not show any gelatinase activity, and five cylA-positive strains showed significant lower hemolysis activity compared with Staphylococcus aureus ATCC25923 (2.48% vs 83.6%, P < 0.01). Table 3 shows the hemolytic and gelatinase activities of the isolates. All the isolates showed γ-hemolytic activity. No strains produced gelatinase. None of the Ent. faecalis isolates were identified as strong biofilm producers; Ent. faecalis 107-1 was identified as a weak biofilm producer (Table 3).
Antibiotic Susceptibility
Table 4 shows the resistance of enterococcus isolates against 15 antibiotics. Overall, all the isolates were resistant to quinupristin/dalofopine and clindamycin, whereas all the isolates were susceptible to benzylpenicillin, ampicillin, ciprofloxacin, levofloxacin, moxifloxacin, tigecycline, nitrofurantoin, linezolid, and vancomycin. The effect of other antibiotics against the isolates varied from susceptible to resistant. Enterococcus isolates 106-1, 107-1, and 113-1 were susceptible to all the antibiotics except erythromycin, quinupristin/dalofopine, and clindamycin.
Virulence Activity Assay by G. mellonella
We used G. mellonella (wax moth) larvae to determine the virulence of the Enterococcus isolates (Fig. 4). We infected the larvae with 10 μl of 1 × 106 CFU/larva, and 1 × 107 CFU/larva. After 72 h, larvae infected with strains 107-1 and 117-1 had 90% survival; all larvae had 100% survival when they were infected by 1 × 106 CFU/larvae of the other Ent. faecalis strains. However, in the 1 × 107 CFU infection group, 100% survival was exhibited only after infection with strains A9-1; a 90% survival was observed for larvae infected with strains A3-1, 67-2, 106-1, 107-1, and 117-1 and a 70% survival was observed for infection with strain 113-1. All larvae in the control groups survived. We analyzed the survival curves statistically using Log-rank (Mantel-Cox) Test; in the 1 × 107 CFU groups, there had no statistical difference among strains except 113-1 (P = 0.735). In the 1 × 106 CFU groups, we did not find any statistical difference among the seven isolates (P = 0.682), which meant that they all lacked virulence activity. Apparently, Ent. faecalis strains A3-1, A9-1, 67-2, 106-1, 107-1, and 117-1 could be considered safe.
Discussions
Ent. faecalis and Ent. faecium are the most common enterococci, which existed in the gut of healthy host [4]. It was reported that enterococci had colonized in the gut of healthy infants immediately after birth, and there had 7.2% of Enterococcus in the fecal samples collected from healthy Chinese infants, which preceded only by Enterobacter (19.0%) and Bifidobacterium (10.9%) (Unpublished data). Enterococcus have attracted much attention due to the probiotic and pathogenic properties [20]. Our study here was aimed to assess the potential probiotic and the safety of the 7 enterococci which were isolated and identified as Ent. faecalis from the healthy Chinese infants.
Before using an organism as a probiotic, properties including strain origin, acid and bile tolerance, adhesion to the intestinal mucus, production of antimicrobial substances, antibiotic resistance or sensitivity, and toxicity should be evaluated [12]. Microbial growth is inhibited at low pH or in high bile salt concentrations. Only strains with genetic makeup to tolerate those conditions survive and proliferate [25]. A probiotic microorganism may have an opportunity to function in the host if it can colonize the gastrointestinal area. In our study, all seven strains survived in MRS medium at pH 5.0, and they grew well during the entire growth period. Moreover, 3.5% NaCl stimulated growth of all six strains except 67-2. At 7% NaCl, strains 107-1, 113-1, and 117-1 even showed stimulated growth. Similarly, we found that 3% bile salt stimulated growth of strain 117-1, and all strains except 67-2 survived above 70% compared with the normal MRS medium. Tolerance to bile salts is of great importance for potential probiotic bacteria, it was established a limit of 0.3% bile for selecting strains, that is, when the growth percentage exceeds 50% compared with the control medium, the tested strain can be considered to show good bile tolerance [20]. Thus, our strains had the high ability to tolerate those stresses, survive, and colonize the host gastrointestinal environment. And in some extent, those stresses could stimulate the bacterial growth, while it could help us to optimize the growth conditions of the bacteria and easily enrich cells.
During the bacteria growth, enterococci can produce organic acids, hydrogen peroxide, enterocin (class II bacteriocin), and other bacteriostatic substances. The supernatant of their growth medium has a broad spectrum of inhibitory effects toward some pathogens. The antibacterial substances produced by probiotics have different degrees of inhibition of pathogens [8]. In our study, all the Ent. faecalis isolates had stronger antibacterial activity against Gram-negative bacteria (especially P. aeruginosa PA01) than that of Gram-positive bacteria. And this antibacterial activity could be associated with the biological characteristics because the indicators Ent. faecalis ATCC29212 and S. aureus ATCC25923 are Gram-positive bacteria and have a cell structure similar to the tested Ent. faecalis strains. On the other hand, enterocins have the potential to inhibit a wide spectrum of the organisms [9], Gram-negative bacteria are generally mostly resistant to bacteriocins from lactic acid bacteria because of their outer membrane providing a barrier to permeability.
Higher ability to adhere to epithelial cells is more able to colonize the host intestine for the potential probiotic bacteria screening, which enables them to compete with pathogenic bacteria for colonization sites [20]. We used the epithelial-like Caco-2 cell line as an intestinal epithelial cell model. In our study, all seven strains showed an ability from 10.6 to 54.9% to adhere to Caco-2 cells. Furthermore, the adhesins of enterococcus bind to the extracellular matrix proteins such as collagen and mucin. Adhesive properties of potential probiotics to the intestinal mucus, which is mediated by collagen-binding protein, mucin-binding protein, is considered important for transient colonization, antagonism against pathogens, modulation of the immune system, and enhanced healing of the damaged gastric mucosa [26]. Strain A3-1 had the highest adherence to mucin, collagen, and BSA in vitro, even higher than that of the probiotic L. rhamnosus GG. While, for the adhesion to Caco-2 cells, A3-1 was not the highest one, which may suggest that different regulations and mechanisms in adhesion in vitro and in vivo [3].Toxicity of bacteria is assessed by genotype and phenotype together [7]. The common virulence factors of enterococci are gelatinase (gelE) and cytolysin (cylA) [17, 27]. The 5 cly-positive strains showed γ-hemolytic activity, and 6 gelE-positive exhibited no gelatinase activity. It has been reported that gelatinase expression is lost in certain growth conditions and environments [15]. Expression of gelE is regulated by the frs operon (frsA, frsB, frsC); deletion or mutation of frs could cause failure of cells to synthesize gelatinase [28]. Biofilm formation is a recognized virulence factor and thought to be associated with presence of esp gene (enterococcal surface protein) and gelatinase production before [29,30,31], while recent study showed esp gene or gelatinase was not compulsorily required to produce biofilm but when present may enhance the biofilm formation, and help Ent. faecalis and Ent. faecium colonize and persist in host tissue [13]. In our study, only strain 113-1 was esp-negative, and it showed moderate biofilm-producing ability. Strain A3-1 showed the highest biofilm-producing ability with esp-positive detection. The aggregation substance (asa1, agg) and collagen adhesion (ace, acm) were associated with adhesion in general [32, 33]. And adhesion properties, a desirable characteristic of probiotic strains, not only biofilm formation, can help bacteria to colonize and stay on the surface of the host mucosa. The Ent. durans LAB18s showed a strong capacity of biofilm formation and associated adhesion ability, this capacity was not associated with the virulence-associated genes [25]. Some studies have suggested that many probiotic enterococci isolates harbor some virulence traits, while none of these antigens should be considered true virulence genes rather than auxiliary factors associated with colonizing ability for their survival in gastrointestinal system [36].
G. mellonella larvae have been used as a model to assess the microbial virulence, which had advantages over other invertebrate models in that they can be incubated at human body temperature, and the inoculum can be delivered directly to the host body [24, 34]. For the survival rate analysis of G. mellonella larvae infected by the tested Ent. faecalis cells, the infection of G. mellonella larvae was stable, where all strains had higher survival with 1 × 106 CFU injection, and there was no difference within different bacterial groups. Strain 106-1 showed higher toxicity at infection dose of 1 × 107 CFU. Thus, the number of injected bacteria may cause differences in virulence, and each strain has a characteristic virulence.
Antibiotic-resistant enterococci have risen in recent years and antibiotic sensitivity is as an important factor in evaluating enterococci, especially resistance to vancomycin [29, 30] Enterococci may be intrinsically resistant or they may acquire resistance. The β-lactams (penicillin, ampicillin) antibiotics are often the first choice for treatment of enterococci infections, which may make the strains more resistant to this type of antibiotics [35]. None of our strains were resistant to β-lactams (benzylpenicillin, ampicillin), fluoroquinolone (ciprofloxacin, levofloxacin, moxifloxacin) antibiotics, nitrofurantoin, oxazolidinone, and vancomycin. Here our strains had the higher resistance to erythromycin (4/7). Similarly, 20 out of 38 enterococcus isolates from healthy Chinese infants were resistant to erythromycin [20]. And there are no broad-spectrum-resistant enterococci isolates, which also proved that our isolates were relatively safe.
Evaluation of beneficial characters and safety is necessary in screening and development of potential probiotics. In our study, we evaluated the probiotic traits and safety properties of seven Ent. faecalis isolates from meconium of healthy infants. We found that strain A3-1 had better stress resistance and probiotic characters, and is considerably safe, with negative hemolysis, no gelatinase activity, non-virulence to G. mellonella larvae, and low antibiotic resistance, which provided a strong impetus of its application in gut research. For development, careful and cautious analysis of virulence potential should be performed by whole genome sequencing and transcriptome profiling, followed by testing in animals.
References
Gomes BC, Esteves CT, Palazzo ICV, Darini AL, Felis GE, Sechi LA, Franco BD, de Martinis EC (2008) Prevalence and characterization of Enterococcus spp. isolated from Brazilian foods. Food Microbiol 25:668–675. https://doi.org/10.1016/j.fm.2008.03.008
Starke IC, Zentek J, Vahjen W (2015) Effects of the probiotic Enterococcus faecium NCIMB 10415 on selected lactic acid bacteria and enterobacteria in co-culture. Benef Microbes 6:345–352. https://doi.org/10.3920/BM2014.0052
Strompfová V, Lauková A, Ouwehand AC (2004) Selection of enterococci for potential canine probiotic additives. Vet Microbiol 100:107–114. https://doi.org/10.1016/j.vetmic.2004.02.002
Gu R, Yang Z, Li Z, Chen S, Luo Z (2008) Probiotic properties of lactic acid bacteria isolated from stool samples of longevous people in regions of Hotan, Xinjiang and Bama, Guangxi, China. Anaerobe 14:313–317. https://doi.org/10.1016/j.anaerobe.2008.06.001
Pieniz S, Andreazza R, Anghinoni T, Camargo F, Brandelli A (2014) Probiotic potential, antimicrobial and antioxidant activities of Enterococcus durans strain LAB18s. Food Control 37:251–256. https://doi.org/10.1016/j.foodcont.2013.09.055
Marciňáková M, Klingberg TD, Lauková A, Budde BB (2010) The effect of pH, bile and calcium on the adhesion ability of probiotic enterococci of animal origin to the porcine jejunal epithelial cell line IPEC-J2. Anaerobe 16:120–124. https://doi.org/10.1016/j.anaerobe.2009.05.001
Li B, Zhan M, Evivie SE et al (2018) Evaluating the safety of potential probiotic Enterococcus durans KLDS6.0930 using whole genome sequencing and oral toxicity study. Front Microbiol 9. https://doi.org/10.3389/fmicb.2018.01943
Nami Y, Abdullah N, Haghshenas B, Radiah D, Rosli R, Yari Khosroushahi A (2014) A newly isolated probiotic Enterococcus faecalis strain from vagina microbiota. J Appl Microbiol 117:498–508. https://doi.org/10.1111/jam.12531
Arokiyaraj S, Hairul Islam VI, Bharanidharan R, Raveendar S, Lee J, Kim DH, Oh YK, Kim EK, Kim KH (2014) Antibacterial, anti-inflammatory and probiotic potential of Enterococcus hirae isolated from the rumen of Bos primigenius. World J Microbiol Biotechnol 30:2111–2118. https://doi.org/10.1007/s11274-014-1625-0
Popović N, Djokić J, Brdarić E et al (2019) The influence of heat-killed Enterococcus faecium BGPAS1-3 on the tight junction protein expression and immune function in differentiated Caco-2 cells infected with Listeria monocytogenes ATCC 19111. Front Microbiol 10. https://doi.org/10.3389/fmicb.2019.00412
Torres-Henderson C, Summers S, Suchodolski J, Lappin MR (2017) Effect of Enterococcus Faecium strain SF68 on gastrointestinal signs and fecal microbiome in cats administered amoxicillin-clavulanate. Top Companion Anim Med 32:104–108. https://doi.org/10.1053/j.tcam.2017.11.002
Morelli L, Capurso L (2012) FAO/WHO guidelines on probiotics: 10 years later. J Clin Gastroenterol 46:S1–S2. https://doi.org/10.1097/MCG.0b013e318269fdd5
Rathnayake IU, Hargreaves M, Huygens F (2012) Antibiotic resistance and virulence traits in clinical and environmental Enterococcus faecalis and Enterococcus faecium isolates. Syst Appl Microbiol 35:326–333. https://doi.org/10.1016/j.syapm.2012.05.004
Kafil HS, Mobarez AM (2015) Assessment of biofilm formation by enterococci isolates from urinary tract infections with different virulence profiles. J King Saud Univ Sci 27:312–317. https://doi.org/10.1016/j.jksus.2014.12.007
Macovei L, Ghosh A, Thomas VC, Hancock LE, Mahmood S, Zurek L (2009) Enterococcus faecalis with the gelatinase phenotype regulated by the fsr operon and with biofilm-forming capacity are common in the agricultural environment. Environ Microbiol 11:1540–1547. https://doi.org/10.1111/j.1462-2920.2009.01881.x
Templer SP, Rohner P, Baumgartner A (2008) Relation of Enterococcus faecalis and Enterococcus faecium isolates from foods and clinical specimens. J Food Prot 71:2100–2104. https://doi.org/10.1089/cmb.2008.C002
Togay SO, Keskin AC, Acik L, Temiz A (2010) Virulence genes, antibiotic resistance and plasmid profiles of Enterococcus faecalis and Enterococcus faecium from naturally fermented Turkish foods. J Appl Microbiol 109:1084–1092. https://doi.org/10.1111/j.1365-2672.2010.04763.x
Marinho AR, Martins PD, Ditmer EM, d’Azevedo PA, Frazzon J, van der Sand S, Frazzon AP (2013) Biofilm formation on polystyrene under different temperatures by antibiotic resistant Enterococcus faecalis and Enterococcus faecium isolated from food. Braz J Microbiol 44:423–426. https://doi.org/10.1590/S1517-83822013005000045
Diego MP, Marta L, María A et al (2009) Adhesion properties of Lactobacillus casei strains to resected intestinal fragments and components of the extracellular matrix. Arch Microbiol 191:153–161. https://doi.org/10.1007/s00203-008-0436-9
Zhang F, Jiang M, Wan C, Chen X, Chen X, Tao X, Shah NP, Wei H (2016) Screening probiotic strains for safety: evaluation of virulence and antimicrobial susceptibility of enterococci from healthy Chinese infants. J Dairy Sci 99:4282–4290. https://doi.org/10.3168/jds.2015-10690
Bobenchik AM, Hindler JA, Giltner CL, Saeki S, Humphries RM (2014) Performance of Vitek 2 for antimicrobial susceptibility testing of Staphylococcus spp. and Enterococcus spp. J Clin Microbiol 52:392–397. https://doi.org/10.1128/JCM.02432-13
Maturana P, Martinez M, Noguera ME et al (2017) Lipid selectivity in novel antimicrobial peptides: implication on antimicrobial and hemolytic activity. Colloid Surface B 153:152–159. https://doi.org/10.1016/j.colsurfb.2017.02.003
Vidana R, Rashid MU, Özenci V, Weintraub A, Lund B (2016) The origin of endodontic Enterococcus faecalis explored by comparison of virulence factor pattern s and antibiotic resistance to that of isolates from stool samples, blood cultures and food. Int Endod J 49:343–351. https://doi.org/10.1111/iej.12464
Martino GP, Espariz M, Gallina Nizo G, Esteban L, Blancato VS, Magni C (2018) Safety assessment and functional properties of four enterococci strains isolated from regional Argentinean cheese. Int J Food Microbiol 277:1–9. https://doi.org/10.1016/j.ijfoodmicro.2018.04.012
Pieniz S, de Moura TM, Cassenego APV et al (2015) Evaluation of resistance genes and virulence factors in a food isolated Enterococcus durans with potential probiotic effect. Food Control 51:49–54. https://doi.org/10.1016/j.foodcont.2014.11.012
Jankowska A, Laubitz D, Antushevich H, Zabielski R, Grzesiuk E (2008) Competition of Lactobacillus paracasei with Salmonella enterica for adhesion to Caco-2 cells. J Biomed Biotechnol 2008:1–6. https://doi.org/10.1155/2008/357964
Barbosa J, Gibbs PA, Teixeira P (2010) Virulence factors among enterococci isolated from traditional fermented meat products produced in the North of Portugal. Food Control 21:651–656. https://doi.org/10.1016/j.foodcont.2009.10.002
Sauvage E, Kerff F, Fonzé E, Herman R, Schoot B, Marquette JP, Taburet Y, Prevost D, Dumas J, Leonard G, Stefanic P, Coyette J, Charlier P (2002) The 2.4-Å crystal structure of the penicillin-resistant penicillin-binding protein pbp5fm from Enterococcus faecium in complex with benzylpenicillin. Cell Mol Life Sci 59:1223–1232. https://doi.org/10.1007/s00018-002-8500-0
Elhadidy M, Elsayyad A (2013) Uncommitted role of enterococcal surface protein, Esp, and origin of isolates on biofilm production by Enterococcus faecalis isolated from bovine mastitis. J Microbiol Immunol Infect 46:80–84. https://doi.org/10.1016/j.jmii.2012.02.002
Sieńko A, Wieczorek P, Majewski P et al (2015) Comparison of antibiotic resistance and virulence between biofilm-producing and non-producing clinical isolates of Enterococcus faecium. Acta Biochim Pol 62:859–866. https://doi.org/10.18388/abp.2015_1147
Garg S, Mohan B, Taneja N (2017) Biofilm formation capability of enterococcal strains causing urinary tract infection vis-a-vis colonization and correlation with enterococcal surface protein gene. Indian J Med Microbiol 35:48–52. https://doi.org/10.4103/ijmm.IJMM_16_102
Franz CMAP, Huch M, Abriouel H, Holzapfel W, Gálvez A (2011) Enterococci as probiotics and their implications in food safety. Int J Food Microbiol 151:125–140. https://doi.org/10.1016/j.ijfoodmicro.2011.08.014
Vankerckhoven V, Van Autgaerden T, Vael C et al (2004) Development of a multiplex PCR for the detection of asa1, gelE, cylA, esp, and hyl genes in enterococci and survey for virulence determinants among european hospital isolates of Enterococcus faecium. J Clin Microbiol 42:4473–4479. https://doi.org/10.1128/JCM.42.10.4473-4479.2004
Mishra AK, Ghosh AR (2018) Characterization of functional, safety, and probiotic properties of Enterococcus faecalis AG5 isolated from Wistar rat, demonstrating adherence to HCT116 cells and gastrointestinal survivability. 10:435–445. https://doi.org/10.1007/s12602-018-9387-x
Peleg AY, Jara S, Monga D, Eliopoulos GM, Moellering RC, Mylonakis E (2009) Galleria mellonella as a model system to study Acinetobacter baumannii pathogenesis and therapeutics. Antimicrob Agents Chemother 53:2605–2609. https://doi.org/10.1128/AAC.01533-08
Tsai H, Liao C, Chen Y, Lu PL, Huang CH, Lu CT, Chuang YC, Tsao SM, Chen YS, Liu YC, Chen WY, Jang TN, Lin HC, Chen CM, Shi ZY, Pan SC, Yang JL, Kung HC, Liu CE, Cheng YJ, Liu JW, Sun W, Wang LS, Ko WC, Yu KW, Chiang PC, Lee MH, Lee CM, Hsu GJ, Hsueh PR (2012) Trends in susceptibility of vancomycin-resistant Enterococcus faecium to tigecycline, daptomycin, and linezolid and molecular epidemiology of the isolates: results from the tigecycline in vitro surveillance in Taiwan (TIST) study, 2006 to 2010. Antimicrob Agents Chemother 56:3402–3405. https://doi.org/10.1128/AAC.00533-12
Funding
This work was financially supported by the National Natural Science Foundation of China (81673199), the Fundamental Research Funds for the Central Universities of China (xjh012019054) and the Natural Science Foundation of Shaanxi province, China (2018JM7054).
Author information
Authors and Affiliations
Corresponding author
Ethics declarations
Conflict of Interest
The authors declare that they have no competing interests to this paper.
Additional information
Publisher’s Note
Springer Nature remains neutral with regard to jurisdictional claims in published maps and institutional affiliations.
Electronic supplementary material
ESM 1
(PDF 1541 kb)
Rights and permissions
About this article
Cite this article
Wang, J., Da, R., Tuo, X. et al. Probiotic and Safety Properties Screening of Enterococcus faecalis from Healthy Chinese Infants. Probiotics & Antimicro. Prot. 12, 1115–1125 (2020). https://doi.org/10.1007/s12602-019-09625-7
Published:
Issue Date:
DOI: https://doi.org/10.1007/s12602-019-09625-7