Abstract
Vinegar is a sour-taste liquid product produced mainly by three sequential steps: saccharification, alcohol fermentation, and acetification. Vinegar has a significant position in Chinese dishes and daily life due to its flavor characteristics and health benefits. It is directly used as a food ingredient or as a diluted solution with water. Nowadays, there are many types of vinegar, and every type has its unique and desirable flavors, differentiated based on their fermentation method and raw material used. This review summarized the fermentation techniques for producing different kinds of vinegar, especially for the four famous Chinese vinegar. Furthermore, the differences between traditional and modern techniques were also described. On the other hand, the previous studies conducted on the analysis of vinegar aroma profiles have been summarized. In addition, previous studies on the analysis of vinegar aroma compounds were elaborated, including the techniques for aroma extraction, identification, and quantification.
Similar content being viewed by others
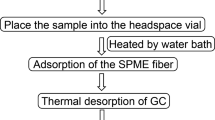
Explore related subjects
Discover the latest articles, news and stories from top researchers in related subjects.Avoid common mistakes on your manuscript.
Introduction
Vinegar is an acidic solution commercially processed through three consecutive phases, including saccharification of carbohydrates, fermentation of alcohol, and acetic acid fermentation. Saccharification is done in the first step to getting the simple sugars from starch, which are subsequently digested by fungi or yeasts to produce ethanol (i.e., second step) (Garcia-Parrilla et al. 2017; Mas et al. 2014). In the third step, ethanol turns into hydrated acetaldehyde, which undergoes dehydrogenation by the enzyme of acetaldehyde dehydrogenase and produces acetic acid, with the aid of oxygen and acetic acid bacteria (Garcia-Parrilla et al. 2017; Canning 1985).
More than 20 types of vinegar are now produced in China, mainly cereal vinegar (Chen et al. 2009). “Among them, the four popular and famous in China are the Shanxi aged vinegar (SAV), Zhenjiang aromatic vinegar (ZAV), Sichuan bran vinegar (SBV), and Fujian Monascus vinegar (FMV)” (Chen et al. 2009). Besides, several kinds of Chinese vinegar are also popular and commonly used in China, such as Longmen vinegar (LV) and Zhengrong rice vinegar (ZRV) (Zhao et al. 2018; Al-Dalali et al. 2019a, 2019b, 2020a).
The aroma profile is one of the significant indices of vinegar, which is reflected by aroma-active volatiles that contribute to the quality of vinegar (Ríos-Reina et al. 2019). Vinegar aromas are affected by several factors, including the region’s local environment, raw materials, the type of microorganisms (starter) (Zhao and Li 2005; Zhang et al. 2019; Sant’Ana 2016), fermentation methods (i.e., modern or traditional) (Morales et al. 2001; Callejón et al. 2009; Cejudo-Bastante et al. 2018; Al-Dalali et al. 2019a), and aging period and aging containers (Ubeda et al. 2016; Ríos-Reina et al. 2019). In addition, some additional materials such as herbal in Sichuan vinegar (Chen et al. 2009), sugar in Longmen aromatic sweet rice vinegar (LASRV), and spices in Longmen aromatic rice vinegar (LARV) can also affect the aroma profiles of vinegar. Besides, some special processing steps such as adding parched rice and decoction process in Zhenjiang aromatic vinegar (Al-Dalali et al. 2019a), solarization process (Palacios et al. 2002) in LASRV and LARV, and smoking process in Longmen smoked vinegar (LSV) (Al-Dalali et al. 2019b) can also contribute to the aroma profiles of vinegar. All the abovementioned special conditions have contributed to the uniqueness of the aroma profiles of respective vinegar.
A vinegar’s aroma profile could include several hundreds of volatiles belonging to various chemical classes, including esters, aldehydes, alcohols, phenols, acids, pyrazines, ketones, and other chemicals (Schreier 1979). However, only some of them contribute to the aroma profile of vinegar because they are aroma-active compounds (Corsini et al. 2019).
The fermentation processes for manufacturing many types of vinegar were reviewed in this article, focusing on the four well-known Chinese vinegar. The distinctions between traditional and modern methods were also discussed. Previous research on vinegar analysis has been summarized in terms of their flavor profiles. Previous investigations on the study of vinegar aroma components, including methodologies for aroma extraction, identification, and quantification, were further elaborated.
History of Vinegar
Vinegar is a sour-taste liquid product, which is directly used as a food ingredient or used as a diluted solution with water (Ho et al. 2017; Lim et al. 2019). The acetic acid content of vinegar ranges from 4 to 7%.
The term vinegar came from the French word “vin” and “aigre,” which means “sour wine” (Lim et al. 2019). The vinegar production can be dated back to 10,000 years ago (Ho et al. 2017; Conner and Allgeier 1976), which was considered a by-product of wine spoilage (Chen et al. 2009; Ho et al. 2017). According to the history of civilization, it was reported that vinegar was developed by the Egyptians, Persians, and Mesopotamians (Mazza and Murooka 2009). Besides, from the historical records, it was written that the vinegar was produced from dates in the Babylonian civilization before 5000 BC, and it was used for food preservation, especially in the pickling (Mazza and Murooka 2009), while, in the Greece civilization, vinegar was used in the medical treatment of sores and to clean ulcerations by a physician Hippocrates (460–377 BC). Also, diluted vinegar was used by the Romanian soldiers in the war as a refreshing and safer drink instead of drinking water alone because it contains antimicrobial substances (Johnston and Gaas 2006; Mazza and Murooka 2009).
The vinegar production in China can be dated back to 3000 years ago. From the previous literature, the first report about the production of vinegar was recorded in a book in the title of “Zhouli” written in 1058 BC about the Zhou Dynasty, and its professional workshop for vinegar processing was organized in the Chunqiu Dynasty (770–476 BC) (Chen et al. 2009; Shen 2007; Hu 2005; Zhao 2004). In ancient times, vinegar production was expensive and consumed only by wealthy noblemen. Until the 25–220 AD during the Donghan Dynasty, vinegar became available for ordinary people (Shen 2007). Afterward, in 420–581 AD, a book titled “Qiming Yao Shu” described 23 different techniques for vinegar fermentation (Chen et al. 2009; Hu 2005).
In Japan, vinegar was used as a powerful beverage for activating the strength and power of Samurai soldiers in the eigth to twelfth centuries (Liu et al. 2014). It was used as a hand-washer during the same period, which was suggested by Sung Tse (1186–1249) during autopsies (Lim et al. 2019; Chan et al. 1994). Besides, in 1348, it was suggested to use vinegar as an antiseptic agent during the spread of plague infection in Italy, which was used to protect the mouth, face, and hand against the infection (Mazza and Murooka 2009). In the seventeenth to eighteenth centuries, it was proposed that drinking vinegar in breakfast could benefit human longevity, whereas John Adams, “the second president of the USA,” who used apple cider vinegar as a daily habit in the breakfast, lived over 90 years (Liu et al. 2014). Although vinegar was initially produced as a home-made product, its industrial production appeared first in Orléans, France, in 1349, which took several months for the vinegar fermentation (Lim et al. 2019). This traditional method is still used today. The upper layer of the produced vinegar is removed, and the lower layer will be mixed with fresh wine or alcoholic solution in a barrel container for subsequent vinegar fermentation (Mazza and Murooka 2009).
The demand for vinegar is increasing rapidly along with the population increase. So, a new method was invented in light of its faster fermentation in Germany in 1823, called the generator process, of which the fermentation time was reduced to 3–7 days. This method involves a large container consisting of two chambers separated by a screen. The acetification process occurs in the upper chamber, while the vinegar product receives in the lower chamber after passing through the screen (Ho et al. 2017).
Recently, in 1955, Hromatka in Germany developed another new and fast method to produce vinegar to meet the increasing demand by consumers. This method is called the submerged (also called modern or industrial) technique. In which, the acetification takes inside a large container fitted with a high-speed motor that is used to apply the air and agitate the mixture, leading to accelerating the fermentation, yielding acetic acid during a short time (Lim et al. 2019; Al-Dalali et al. 2019a; Ho et al. 2017). Currently, vinegar factories have common acetators with capacities ranging from 10,000 to 40,000 L (Ho et al. 2017).
The taste of Chinese vinegar is sweeter than the taste of other vinegar produced in western countries. Several spices are added to Sichuan vinegar during the brewing process to enhance its flavor (Xu et al. 2009). In general, most Chinese vinegars are fermented by traditional methods. However, a new technique called submerged liquid brewing for both cereal and fruit vinegar is also used to meet the growing demand for vinegar (Xu et al. 2009).
Types of Chinese Vinegars
Many types of vinegar are produced globally, differentiated based on their fermentation method and raw material used. Among them, several types are well known for their unique aroma profile, such as “balsamic vinegar, rice vinegar, cider vinegar, malt vinegar, and sherry vinegar” (Lazim et al. 2019). More than 20 types of cereal vinegar are available in the Chinese market (Chen et al. 2009). These vinegars are brewed from cereal products such as rice, barley, corn, wheat, and sorghum. Among them, four brands are famous in China, including (1) SAV made from sorghum as raw material and Daqu (as a starter); (2) ZAV made from sticky rice as raw material and Daqu and produced by multilayer solid-state fermentation (SSF); (3) SBV made from wheat bran, Daqu, and several types of spices (herbs); and (4) FMV made from sticky rice as raw material and red yeast rice as a saccharifying agent and produced by liquid state fermentation (LSF) (Liu et al. 2004; Chen et al. 2009; Huang and Cai 1998).
Besides the most famous four Chinese vinegars, there are other well-known types of vinegar in China and commonly used in Chinese daily life, such as:
-
1-
Shanghai rice vinegar: amylase is used as a fermentation agent instead of Qu and is produced by modern fermentation.
-
2-
Zhejiang rose vinegar is made from steamed rice, allowing the Monascus to grow spontaneously during alcohol fermentation. At the same time, acetification is conducted with the aid of acetic acid bacteria through SSF/SmF (Lazim et al. 2019).
-
3-
For Longmen vinegar, several types are made from normal rice or sticky rice (the details will be described in the production of Chinese vinegar part) (Al-Dalali et al. 2020c).
-
4-
Zhengrong vinegar is made from ordinary rice (Chen et al. 2009; Al-Dalali et al. 2019b; Al-Dalali et al. 2020a, b, c).
Production of Chinese Vinegar
Chinese vinegar can be fermented by SSF or submerged fermentation (SmF), where traditional or modern techniques can carry out the latter. In all the cases, the vinegar produced by the SSF or the traditional SmF is preferred over other products made by the modern SmF in terms of their contents of main components and their unique flavor characteristics. On the other hand, during the SSF brewing, the generation of substances is formed continuously and simultaneously compared to their semi-continuous generation in the modern SmF (Xia et al. 1985; Liu 1982). However, the fundamental of brewing is almost the same as shown in Fig. 1 (Chen et al. 2009).
Production of Vinegar by SSF Procedure
Most modern Chinese vinegars are produced by solid-state fermentation. It is also called “parallel fermentation,” in which slow saccharification of starch occurs at low temperatures to produce alcohol (Xu et al. 2009). Saccharification is the first step of vinegar production. It depends on the action of an enzyme (i.e., “glucoamylase”) to produce glucose from starch, which serves as a substrate consumed by yeast or fungi to produce alcohol further. The last step involves the oxidation of alcohol by acetic acid bacteria with oxygen and wheat or rice hull to produce acetic acid (Xu et al. 2009). Subsequently, the collected vinegar is matured, filtered, and pasteurized for bottling. Produced vinegar by the SSF technique has its unique flavor characteristics and can be classified into several types as follows, (1) Daqu vinegar, (2) Xiaoqu vinegar, and (3) Fuqu vinegar based on the saccharifying agent used.
Qu (koji in Japanese) is a saccharifying agent used in SSF. It is prepared by mixing grain (i.e., rice, wheat, beans, sorghum, and barley) with water, on which the molds grow spontaneously by controlling the temperature and air contents for a long time. The main microorganisms in Qu starters include molds (i.e., Rhizopus, Aspergillus, and Monascus) and bacteria (lactic acid bacteria), and yeast (Saccharomyces). It also includes many produced enzymes by microorganisms (i.e., “glucoamylase, α-amylase, lipase, and acid protease”) (Steinkraus 2004; Chen et al. 2009).
There are many forms of Qu in China, including Maiqu, Daqu, Xiaoqu, Fuqu, and Hongqu. The main differences among them are the raw materials, shape, and the dominant microbe. For example, the Maiqu prepared from the wheat in the brick shape contains Aspergillus spp. as a dominant microbe. In contrast, the Xiaoqu prepared in an egg shape is made from rice, and the Rhizopus spp. as its main microorganism and Daqu is prepared in a brick shape from the wheat, peas, and barley as raw materials with the dominant Mucor spp. (microbe) (Chen et al. 2009; Chen 1999; Huang and Cai 1998).
Production of Vinegar by LSF Technique
The liquid or semi-liquid state technique is commonly used in Europe and China. This method produces two famous Chinese vinegars, including Fujian red rice and Zhejiang rice vinegars. It is not complicated like SSF. The technique has serval types, including surface fermentation, sparged fermentation, and submerged fermentation (Xu et al. 2009).
Nevertheless, some modifications have been made in this fermentation process, which a new method is adapted by applying both SSF and LSF during the production, where the LSF is used to produce alcohol and then followed by modern SSF to produce acetic acid. The acetification process in the modern SSF occurs in a cement pool fitted with the aeration instead of jars. As a result of combining both techniques, vinegar is produced on a large scale. On the contrary, alcohol and acetic acid are produced inside jars in the traditional technique, which takes a long time and large spaces (Xu et al. 2009; Chen et al. 2009).
Submerged Fermentation
Vinegar can be produced by one of the three primary methods: “static, SSF, or submerged fermentation.” The submerged fermentation (also called modern fermentation) represents a rapid technique that strictly regulates oxidative conversion processing parameters, such as oxygen, temperature, acidity, and alcohol content. It requires a strong energy input and is performed to produce modern vinegar. In comparison, the static and SSF are generally used with a long brewing time to produce traditional vinegar, resulting in low energy consumption (Mazza and Murooka 2009). The first study that used submerged fermentation in vinegar production was conducted in 1923, but its first industrial application was in the 1940s. Then, in the 1950s, the method was improved by the invention of cavitator, which facilitates air intake into the mixture. Finally, the last improvement was achieved by the invention of automated Frings acetators, which have shown good ability and efficiencies in vinegar production. This technique can be conducted quickly for approximately 20 to 24 h at 30 °C for the production of wine vinegar (see Fig. 2), while in the Chinese vinegar it takes place for a long time, determined about 20 days, and is usually handled in an oblong cement pool (Budak et al. 2014; Natera et al. 2003; Garcia-Parilla et al. 1997; Xu et al. 2009; Callejón et al. 2009).
In the 1960s, an improvement was achieved in vinegar production, like the concentration of vinegar by “Girdler process equipment chemetron corporation.” In this method, vinegar is concentrated, frozen, and used in many new applications, such as modern pickling methods (Mazza and Murooka 2009).
Finally, acetic acid fermentation can be conducted by either traditional fermentation or modern (industrial) fermentation. As we know, acetic acid is a precursor to producing many flavor compounds (Wang et al. 2015) and contributes to the desired quality of vinegar (Morales et al. 2001). The modern technique is popularly known as the submerged method. It is a quick fermentation method due to the availability of oxygen that encourages the starter’s growth (acetic acid bacteria; AAB). The action of agitation provides oxygen. Also, this method can produce a large amount of vinegar to meet the increasing demand, or be conducted in a small size, for example, in the oblong cement pool (Natera et al. 2003; Garcia-Parilla et al. 1997; Xu et al. 2009; Budak et al. 2014; Callejón et al. 2009; Al-Dalali et al. 2019a).
In comparison, the traditional method is called slow fermentation or surface fermentation. AAB grows on the surface of vinegar pei inside a wood barrel or pottery jars to get oxygen for its growth. This method takes a long time and needs ample space to keep the jars or the wood barrels (Callejón et al. 2009; Al-Dalali et al. 2019a; Chen et al. 2009).
Various Fermentation Procedures Used to Produce Chinese Vinegars
The main steps in Chinese vinegar production are similar, including saccharification, alcohol fermentation, acetification, adding salt, aging, filtration, pasteurization, and packaging. But there are special processes in every type of vinegar that could be responsible for its distinctive aromas, which can be summarized as follows:
In ZAV, its fermentation involves using two Qu types: Daqu and Xiaoqu, as starting agents for alcoholic fermentation (Wang et al. 2015; Al-Dalali et al. 2019a). It also applies a multilayer fermentation during the production of acetic acid (Shen 2007; Chen et al. 2009), uses parched rice as a coloring agent (Shen 2007), and in particular, adopts decoction in its last processing (Chen et al. 2009). The decoction is carried out by combining the vinegar obtained after its leaching stage with 2% sugar, and then this mixture is heated to 102 °C for over 60 min (Liu et al. 2016). Finally, during the aging of ZAV, both vinegar pei and vinegar are applied to the aging process (Chen et al. 2009).
SBV is also known as an herbal vinegar because it uses herbal Daqu as a starter prepared by more than 60 traditional Chinese herbs. So, it is also considered the only medicinal vinegar in China (Chen et al. 2009; Shang 2003). Chen et al. (2009) reported the involved herbs, including licorice, eucommia bark, and villous amomum fruit. This benefits the growth of Rhizopus spp., (main fungal in Daqu) during the brewing process, making a significant contribution to the yield of the unique aroma profile of SV (Shang 2003).
In SAV, its fermentation has some special characteristics that involve thermal heating of vinegar pei, which it exposes to a higher temperature (80–90 °C) by a mild fire on the third day, and then on the sixth day, its temperature is gradually decreased to 50 °C (Huang and Cai 1998; Chen et al. 2009). This distinct process could improve the color, fragrance, luster, taste, and smoky odor of vinegar products. During the vinegar aging of SAV, only the fresh vinegar keeps inside the open vase in an open room for more than 1 year. The volume of vinegar is decreased to about one-third of its original volume due to intense vaporization through the exposure of the vase to the sun during the summer, while in winter, as a result of ice removal from the vase, the amount of non-volatile acids, soluble solids, and volatile flavor compounds increases (Chen et al. 2009).
In FMV, its fermentation involves using Gutian Hong Qu as starting agents for saccharification and alcoholic fermentation due to its content of many hydrolytic enzymes, such as glucoamylase, lipase, α-amylase, β-amylase, and protease, produced during FMV fermentation by Monascus spp. Also, its flavor could be improved by adding white sugar and sesame during the fermentation processes (Huang and Cai 1998; Chen et al. 2009). After the first step of alcoholic fermentation, red rice wine with a high concentration of ethanol and the amino acid was kept inside the first vase with 1-year-old vinegar at a ratio of 1:1 v/v and mixed well for 1 year. In the second year, one part of the last patch is mixed with one part of 2-year-old vinegar, and the same for the third and fourth years (Huang and Cai 1998; Chen et al. 2009).
In Longmen vinegar, there is four common Longmen vinegars, including the Longmen aromatic sweet rice vinegar (LASRV), Longmen aromatic rice vinegar (LARV), Longmen rice vinegar (LRV), and Longmen Smoked Vinegar (LSV). The main differences among the four vinegars mentioned above are as follows: (1) the LASRV, LSV, and LRV use normal rice as the raw material, while the LARV uses the sticky rice; (2) the LASRV and LARV are solarized for 3 months before the sterilization, while the LRV does not adopt the solarization; and (3) sugar is added before the solarization process of LASRV, while spices are added before the pasteurization process of LARV; however, neither the sugar nor spices are added to the LRV; (4) the LSV is processed by an additional smoking procedure (Al-Dalali et al. 2019b, 2020c). The solarization (also called solera system) is a process by which the vinegar is poured into a big vat (or container) and stored for an extended period in the glasshouse to facilitate the biochemical reactions involved in the vinegar processing, as well as to decrease the water content by the evaporation (Palacios et al. 2002). In addition, the LSV is handled by an additional smoking process, which includes the following steps: a half amount of Vinegar Pei (the intermediate vinegar product) is moved to a closed jar before its filtration, heated gradually to keep the vinegar at 70–80 °C, and stirred once a day for 7 days. This thermally heated (or smoked) vinegar product will then be mixed with the remaining half of the vinegar without smoking, followed by filtration and pasteurization (Li and Tan 2009; Al-Dalali et al. 2019b). This special processing gives the LSV a unique smoky aroma profile containing many volatile chemicals, including aldehydes, phenol, pyrazines, and ketones.
Uses of Vinegar
As mentioned before, vinegar contains acetic acid as the main component, besides other bioactive and nutritional compounds such as vitamins and phenolic acids. So, it is used to prevent human diseases and improve human health or used as condiments in salad dressing, sauce, mustard, mayonnaise, and ketchup. Besides, it is commonly used as a taste and aroma enhancer (Lim et al. 2019). Also, it is used during the pickling of vegetables and fruits as a preservative agent against microbial spoilage. Due to its high acidity, vinegar can be used as a disinfectant and cleaning agent (Lim et al. 2019).
Characterization of Aroma-Active Compounds in Vinegar Using Gas Chromatography–Olfactometry (GC-O)
GC-O is often used to identify aroma-active compounds. GC facilitates the separation of volatile compounds in the tested samples, and the human nose is used as a detector to smell and determine the intensity and odor of the separated odorants (Úbeda et al. 2019). Several techniques have been applied in GC-O to characterize aroma-active compounds by identifying and quantifying the odorants depending on the length of elution time of aroma (the initial time to sniff the odor until it disappears). These methods included:
-
(1) Dilution to the Threshold
These methods are applied to quantify the potency of odor compounds depending on “the odor concentration and its perception threshold in the air” (Úbeda et al. 2019). Among these methods are “combined hedonic aroma response measurement (CHARM), and aroma extract dilution analysis (AEDA).” The difference between both mentioned methods is the registered data during the sniffing. In the first method, the data required during the registration is the aroma length (Plutowska and Wardencki 2008; Úbeda et al. 2019), while the data needed to register in the AEDA method is the ability of individual odor to be sniffed at the maximum dilution (Úbeda et al. 2019).
-
(2) Time-Intensity
In this method, the panelists are asked to register the presence or absence of the odor and its intensity based on the defined scale (Úbeda et al. 2019).
-
(3) Frequency Detection
This is also called nasal impact frequency (NIF). In this method, the panelists are asked to sniff the same sample under the same conditions and record the odor’s presence or absence, intensity, and description. If the odor is sniffed more frequently by all the panelists at the same retention time, its intensity value equals 100%. Besides, it is defined as a combination of frequency and intensity and called modified frequency (MF). The calculation way of this method is explained in this review in the quantitation part. Finally, the advantages of this method are it is simple, it needs less time and shows good reproducibility, and no more training is required for the panelists (Úbeda et al. 2019).
Survey on Flavors and Aroma-Active Compounds Identified in Chinese Vinegar
Vinegar is a fermented product mainly produced by three steps, in which volatile compounds are mainly formed (Callejón et al. 2009). Besides, the aging process also makes a significant contribution to the final aroma profile of vinegar, since most of the volatile compounds are generated during this step, and some of them decrease or increase their concentrations depending on the aging time, condition, post-treatment, and aging container (Callejón et al. 2010). The main volatile chemicals of vinegar include carboxylic acid, alcohols, acetic acid esters, and ethyl esters. Different kinds of vinegar have different volatile profiles in contents of ketones, aldehydes, terpenes, acetals, lactones, pyrazines, or phenols (Úbeda et al. 2019). The most commonly identified compounds in vinegar are acetoin, acetic acid, and ethyl acetate (Callejón et al. 2008; Guerrero et al. 2007). Acetic acid contributes a sour, acidic, and pungent odor to the aroma profile of vinegar. Besides, many other chemicals, such as esters, aldehydes, ketones, and other organic volatiles, also contribute to the aroma profile of vinegar (Ozturk et al. 2015; Ho et al. 2017). These compounds are generated through vinegar production (i.e., fermentation and aging) and are affected by the used raw materials, the starter, and the fermentation technique (Pizarro et al. 2008; Lim et al. 2019).
Several studies have been done to characterize flavor and aroma-active compounds in different types of Chinese vinegar as follows:
Zhou et al. (2017) identified volatile flavor and aroma-active compounds in the ZAV using GC × GC-TOFMS and GC-O. The authors identified about 360 volatile compounds in the vinegar samples; the ketones were detected in many identified compounds, followed by esters, aldehydes, furans, and alcohols. Besides, they found that furfural, methanthiol, phenethyl acetate, 2- and 3-methylbutanoic acid, 2-methyl propanal, octanal, 2- and 3-methyl butanal, trimethylpyrazine, 1-octen-3-one, and acetic acid were the most contributors to aroma profiles of ZAV. On the other hand, 35 compounds were identified as aroma-active compounds. Lu et al. (2011) identified the volatile compounds of the ZAV using a supercritical fluid extraction combined with GC–MS, by which 44 compounds were identified. Al-Dalali et al. (2019a) compared the aroma profile between the modern and traditional ZAV using HS–SPME–GC–MS and GC-O. They identify 53 volatile compounds in both samples, including 29 aroma-active compounds. Regarding the concentration, most of the individual chemical concentrations and the concentration of their relative chemical groups were higher in the modern samples than in their traditional counterpart. Besides, butyl benzoate and isopentyl alcohol were detected only in the traditional samples, while propiophenone was detected only in the modern samples. Finally, the total concentrations of chemical groups were increased along with the aging time in the modern samples. In the same period, their concentrations in the traditional samples were decreased with increasing the aging time, except for total concentrations of esters and acids groups.
Al-Dalali et al. (2019b) evaluated the aroma profiles of commercial ZAV, LSV, and ZRV using HS-SPME combined with GC–MS and GC-O. A total of 75 volatiles and 30 aroma-active compounds were characterized, in which 22 compounds were detected in the Zhengrong rice vinegar, 23 in the ZAV, and 26 in the LSV, while 18 were detected in all three types of vinegar. On the other hand, two aromas were identified for the first time in vinegar as aroma-active compounds: phenol acetone and 3,5-diethyl-2-methylpyrazine.
Liang et al. (2016) characterized the aroma compounds in the SAV before and after its aging by using SAFE-GC–MS and GC-O. As a result, 87 aroma-active compounds were characterized by GC-O, and 7 compounds were found at high concentrations after aging, which were furfuryl alcohol, 3-hydroxy-2-butanone, 2,3-butanedione, furfural, acetic acid, 3-methylbutanoic acid, and tetramethylpyrazine. Xiong et al. (2015) evaluated the aroma profiles of 13 samples of SAV, 22 samples of ZAV, and 15 commercial Chinese vinegar. They reported that fifteen volatile flavor compounds were identified as markers to discriminate between ZAV and SAV. They were butyl acetate, butanoic acid, 2-methylbutyric acid, 2-butanol, 3-methyl-1-butanol, 3-ethoxy-1-propanol, 2,5-dimethylhexanol, 2-acetyl furan, and 2,2-dimethyl-1,3-dioxolane-4-carboxaldehyde as markers in ZAV samples, while phenyl propyl acetate, propionic acid, 2-methoxyphenol, 4-allyl-2-methoxyphenol, 1-phenyl-2-propanone, and 2,3-dimethylpyrazine were identified as markers for Shanxi aged vinegar. Zhu et al. (2016) reported that the volatile extraction at 50 °C for 20 min with the aid of DVB/PDMS was the optimal condition from SAV samples. Twenty-three compounds were identified; 19 were characterized as aroma-active compounds after calculating their odor activity values (OAVs). Besides, they found that the furfural, propanoic acid, 3-methylbutanoic acid, acetic acid, butanoic acid, acetoin, and trimethyloxazole were elaborate most powerful odorants. Aili et al. (2011) characterized the aroma-active compounds in SAV by dynamic headspace extraction. They found that 45 compounds were detected. Among them, 13 were identified for the first time in vinegar.
Xiao et al. (2011) characterized the aroma profiles of several types of Chinese vinegar using HS–SPME–GC–MS at the following extraction condition: 45 °C and 30 min. The authors found several chemical groups, including aldehydes, esters, ketones, acids, alcohols, pyrazines, phenols, and other miscellaneous chemicals. Besides, they found that the major volatiles in Chinese vinegar were furfural, phenylethyl alcohol, acetic acid, 3-hydroxy-2-butanone, ethyl acetate, isopentyl acetate, 3-methyl-1-butanol, and benzaldehyde. Al-Dalali et al. (2020a, b, c) investigated the effect of fermentation procedures on aroma changes of Zhengrong vinegar by using HS-SPME. Those fermentation procedures include “before adding salt, after adding salt, after filtration, and final product.” The authors found that the individual chemical concentration was decreased along with the fermentation process. Besides, two aroma-active compounds were identified for the first time in vinegar, and they were ethyl hexanoate, and 1,2-dimethoxybenzoate. Zhao et al. (2020) analyzed the flavor and metabolite components in Chinese rose vinegar using the HS-SPME and silylation, respectively, in GC–MS. They reported that 48 volatile flavor compounds and 76 metabolites were identified. Besides, after calculating OAVs, the results showed that aldehydes contributed greatly to the aroma of this type of vinegar. Among them are decanal, dodecanal, nonanal, heptanal, and 3-methylbutanal. Al-Dalali et al. (2020b) compared the traditional and modern SBV products in molecular sensory science. They found that 42 aroma-active compounds were detected; 26 of them have OAVs larger than 1. Besides, they concluded that acids and esters showed a high contribution to the aroma profiles of this type of vinegar. Al-Dalali et al. (2022) evaluated the changes in volatile flavor in the same batch of Zhengrong vinegar during 2 years of aging. During the several stages of aging, they discovered a total of 67 volatiles and 30 aroma-active compounds. Many aroma-active compounds, such as trimethylpyrazine, methional, 2-acetyl-3-ethylpyrazine, and acetophenone, were produced during the aging process, whereas many pyrazines were developed after 24 months.
Survey on Flavors and Aroma-Active Compounds Identified in Western Vinegar
This part was added to this review to provide a general conclusion for the researchers and readers interested in the aroma profiles of vinegar. Several studies concerning western vinegar are summarized in this part as follows:
Del Signore (2001) compared the aroma profiles of 56 types of traditional balsamic vinegar. The authors found that the samples contained more ester compounds and propionic acid. Moreover, the traditional samples showed higher heptanal, diacetyl, octanal, and hexanal contents than the other samples. Chinnici et al. (2009) characterized volatile compounds of traditional and common balsamic vinegar and sherry vinegar by solid-phase extraction (SPE) coupled with GC–MS. They identified and quantified 90 volatile compounds, including furans, esters, and short-chain fatty acids. In another study of the traditional balsamic vinegar investigated by Guerrero et al. (2008), the authors reported an optimal condition to extract the vinegar volatiles by SPE as follows: 7 g of diluted sample (1:4), 5 mL water, and 10 mL of dichloromethane solvent. Corsini et al. (2019) characterized aroma-active compounds in several types of balsamic vinegar by GC-O with a modified frequency (MF) tool. Forty-five aromas were identified. Among them, 14 compounds have MF ≥ 60%.
Aceña et al. (2011) characterized the aroma-active compounds in the Sherry vinegar by HS-SPME-GC-O. The authors found that 37 aromas contributed to its characteristic aroma profile. Among them, 13 compounds were found for the first time in the Sherry vinegar. Besides, they reported that ethyl isovalerate, ethyl isobutyrate, isoamyl acetate, and isovaleric acid had contributed more than other aromas based on their high OAVs. Callejón et al. (2008) also studied the aroma compounds of the Sherry vinegar. They identified 58 compounds by GC–MS. Among those volatiles, 7 were reported for the first time in the Sherry vinegar. In addition, ethyl isobutyrate, diacetyl, ethyl acetate, stolon, isoamyl acetate, and isovaleric acid were the main contributors to the Sherry aroma profile to their high OAVs.
Morales et al. (2001) summarized that the aroma profile of Sherry vinegar produced by the traditional method is different from that produced by the submerged method and concluded that the acetification technique played a significant role in forming its unique aroma profile. Mejías et al. (2002) found that the CAR/PDMS fiber was the best one to extract the volatile flavor compounds from commercial Sherry vinegar. Also, they reported that when the vinegar samples contained a high concentration of acetic acid, the extraction efficiency was significantly decreased. Bruna-Maynou et al. (2020) improved the aroma profiles of Sherry vinegar by maceration of orange peels inside the vinegar. They found that the best-applied conditions of ultrasounds were orange peels 200 g/L, pulses 20 s off and 40 s on, and 550 w/L sonication power, in which 82 volatile flavor compounds were identified. It can be concluded that the maceration of orange peels with ultrasound treatment for vinegar improved its aroma profile and its accessibility to assessors. Chanivet et al. (2020) evaluated several types of oak wood (i.e., Spanish oak, American oak, French oak, and chestnut barrels) through the whole year aging of Sherry vinegar to find the best one in terms of its contribution to the aroma profile of this type of vinegar. They reported that the chestnut barrels and Spanish oak had seemed the best alternatives for the American oak during the aging process of Sherry vinegar.
Charles et al. (2000) characterized aroma compounds in wine vinegar samples by GC-O. They reported that acetic acid, 2- and 3-methyl-1-butanol, 3-methylbutyric acid, 2-methylbutyric acid, 2-phenyl-1-ethanol, butyric acid, 2,3-butanedione, 3-(methylthio)-1-propanol, and 3-hydroxy-2-pentanone were characterized as the important aroma-active compounds in the tested samples. Callejón et al. (2009) characterized the volatile compounds between the traditional and modern fermentation of red wine vinegar. The authors found that concentrations of volatile compounds were higher in traditional fermentation than in modern fermentation, except acid compounds predominate in modern samples. Besides, among the 57 identified compounds, 3 of them were reported for the first time in wine vinegar, including limonene, ethyl benzoate, and ethyl furoate. Callejón et al. (2010) evaluated changes in volatiles of wine vinegar during the aging in different barrels’ woods. They found acetophenone, ethyl furoate, benzaldehyde, and ethyl benzoate in high concentrations in cherry barrels. On the other hand, the eugenol and oak lactones were found in the chestnut and oak barrels in the aged vinegar. Ríos-Reina et al. (2019) found that acetate compounds were the most abundant in Spanish wine vinegar. Besides, 22 compounds out of 160 detected compounds in these types of vinegar were identified for the first time in vinegar.
Cejudo-Bastante et al. (2018) analyzed traditional and modern fermentation techniques of orange vinegar. The latter technique resulted in higher concentrations of volatile compounds and better custom acceptability based on the sensory analysis. Ubeda et al. (2012 and 2016) characterized aroma-active compounds in strawberry vinegar using LLE with GC-O using modified frequency (MF). The results showed that about 79 aromas were detected in several samples. Among them, 12 were reported as more contributors to the aroma profiles of vinegar samples, including methional, 2-phenylethanol, sotolon, acetic acid, vanillin, 3-nonen-2-one, butyric acid, phenyl acetic acid, pentalactone, isovaleric acid, p-vinylguaiacol, and furaneol.
Natera et al. (2003) evaluated the aroma profile of 83 types (i.e., wine vinegar, balsamic vinegar, cider vinegar, honey vinegar, and apple vinegar). The chemometric studies grouped these vinegars based on their raw materials and the fermentation processes in which apple and balsamic vinegars were clustered together. In contrast, cider, honey, and alcohol vinegars were also clustered in one area. Besides, the abundant compounds in the tested samples were 3-hydroxy-2-butanone, 3-methylbutanol, 2,3-butanediol, 2-phenylethanol, and isopentanoic acid. Ozturk et al. (2015) studied the aroma profiles of Turkish vinegar made by traditional and industrial methods. The authors found that the major compounds in the traditional samples were ethyl acetate and α-terpineol, compared to the isoamyl acetate and octanoic acid in the industrial samples. In addition, ethyl acetate and isoamyl acetate were identified in the vinegar produced from grapes as raw material, and octanoic acid was found in vinegar produced from the pomegranate. Table 1 summarizes the published papers about the volatiles of vinegar in both English and Chinese languages.
Chemometric Analysis of Aroma-Active Compounds in Chinese Vinegar
Principal component analysis (PCA), variable importance in projection (VIP), and Venn diagram were analyzed using MetaboAnalyst 5.0 and Unscrambler X 10.4 software. Aroma-active compounds were summarized in all the kinds of Chinese vinegar and applied for chemometric analysis. A total of 126 aroma-active compounds were characterized by GC-O in several types of Chinese vinegar, including SAV, SBV, ZAV, LSV, LASRV, LARV, LRV, and ZRV (Table 2). Among them, 38 aromas were reported only in SAV, and they were ethyl propanoate, p-cresol, (Z)-2-nonenal, propanoic acid, 2-acetylfuran, ethyl caprate, 4-propylguaiacol, pyrazine, 2-acetyl-3,5-dimethylpyrazine, dihydro-2-methyl-3(2H)-furanone, β-damascenone, methyl tetradecanoate, estragole, ethyl pyrazine, 2,3,5-trimethyl-6-ethylpyrazine, anethole, benzophenone, γ-pentalactone, hexadecanoic acid ethyl ester, dimethyl disulfide, benzoic acid, γ-decalactone, 3,5-dihydroxybenzaldehyde, 2-acetylpyrazine, pantolactone, butanedioic acid, monomethyl ester, piperonal, heptanal, 2-ethyl-6-methyl pyrazine, 2-butanone, 3-(2-furanyl)-2-propenal, 2,5-dimethyl-3-isopropylpyrazine, (3E)-4-(2-furyl)-3-buten-2-one, 2-phenyl-3-(2-furyl)- propenal, γ-hexalactone, methyl pyrazine, phenol, and isoamyl formate (Table 2 and Fig. 3A). ZAV was characterized by 13 aromas compared to other kinds of Chinese vinegar; they were 2-methylbutanal, octanal, 4-vinylguaiacol, butyl benzoate, 2,6-dimethyl-pyrazine, safranal, dimethyl sulfide, methanethiol, 1-octen-3-one, 2-methylpropanal, 2,5-dimethyl-2,4-dihydroxy-3(2H)-furanone, carbon disulfide, and 1-octen-3-ol (Table 2 and Fig. 3A). On the other hand, 12 aromas were only reported in SBV were butyrolactone, benzothiazole, (E)-3-(furan-2-yl)-2-methylprop-2-enal, 2-phenyl-3-(2-furyl)-propenal, heptanoic acid, 1H-pyrrole-2-carboxaldehyde, 2,4,5-trimethyl-1,3-dioxolane, 4-methylpentanoic acid, 1-(4-hydroxy-3-methoxyphenyl)-ethanone, 2-phenylacetic acid, and phenylmethanol (Table 2), while only one aroma-active compound was reported in ZRV, and two aromas were only reported in LV (Table 2). On the other side, the VIP method was applied to screen the marker components among the several Chinese vinegars, as shown in Fig. 3B. Ten components had VIPs larger than 1.0. Among them, 7 components were found with high-frequency values in LV, including isopentyl alcohol, butanoic acid, trimethyloxazole, 2-furanmethanol, 2-ethyl-4,5-dimethyloxazole, ethyl 3-(methylthio)propionate, and benzoyl methyl ketone. In the SAV, isopentyl alcohol, butanoic acid, trimethyloxazole, 2-furanmethanol, and benzoyl methyl ketone showed VIPs larger than 1.0, as shown in Fig. 3B, while SBV was characterized by two components that had VIPs larger than 1.0, namely, 2-furanmethanol and 2-methylbutanoic acid, and ZAV included butanoic acid and 2-methylbutanoic acid (Fig. 3B). On the other hand, ZRV contained three components with VIPs larger than 1.0, 2-methylbutanoic acid, 2-ethyl-1-hexanol, and 1,2-dimethoxybenzene.
A Venn diagram of aroma-active compounds (VIP > 1.0) identified in several kinds of Chinese vinegar; and B important aroma-active compounds (VIP > 1.0) identified by PLS-DA in several kinds of Chinese vinegar, the numbers in accordance with the numbers in Table 2
PCA was performed for the aroma-active compounds in Table 2, using the normalized reported compounds as the variable and the vinegar brands as the samples, and the results are shown in Fig. 4. The sum of the PC-1 and PC-2 components explained nearly 71.0% of the total variance. Also, the vinegar brands of samples were scattered in the score plot and divided into three regions. The SAV was distinguished well from other vinegar brands. These could be mainly attributed to the different specific processes during the fermentation and aging of this kind of vinegar. At the same time, the SBV was distinguished well from other vinegar brands (Fig. 4). This could be attributed to the added herbs for this kind of vinegar during fermentation. On the other hand, the vinegar brands of LV and ZRV were placed close together, indicating that these vinegar brands’ flavor compositions and fermentation processes are similar.
Proposed Mechanisms for the Formation of Aroma-Active Compounds
Aroma compounds could be formed during the fermentation and aging process through different reactions, including caramelization, Maillard reaction, thermal degradation, oxidation of lipids, and the degradation of major and minor components, such as proteins, sugars, vitamins, pigments, and ribonucleotides (Diez-Simon et al. 2019).
Maillard Reaction
The first study of the Maillard reaction was conducted by Louis-Camille Maillard (1912), who identified the reaction between reducing sugar and peptides. Generally, the Maillard reaction is defined as the occurred reaction between reducing sugar and amino acids. It is recognized as the most important way to form flavor and color compounds in processed foods (Shahidi et al. 2014; Diez-Simon et al. 2019). Hundreds of possible reactions can occur through the reaction between the amino acid and reducing sugar, yielding hundreds of flavor and color compounds in processed foods (Diez-Simon et al. 2019). Nowadays, food scientists consider this complex reaction to identify and characterize the proposed mechanisms and identify new compounds that contribute to the food flavors (Tamanna and Mahmood. 2015; Jaeger et al. 2010; Diez-Simon et al. 2019). Pathway of Maillard reaction can be divided into three phases, which include the following: (1) the early stage occurred between amino acid and reducing sugar by the condensation of sugar-amine to generate n-glucosylamine in case of the sugar is hexose or n-fructosylamine in the case of the sugar ketose, and then amadori rearrangement to produce 1-amino-2-deoxy-2-ketose; (2) the second stage is the intermediate phase, in which several reactions are included such as deamination to form deoxyosones that undergo sugar fragmentation (sugar breakdown) to generate reductones and dehydroreductones that formed furanones, pyranones, pyrroles, and thiophenes, retro-aldolization to form several flavor compounds (hydroxyacetone, cyclotene, dihydroxyacetone, hydroxyacetyl, glyoxal, pyruvaldehyde, glycoaldehyde, and glyceraldehyde), and strecker reaction to produce aldehydes and aminoketones, which further undergo heterocyclization to generate several flavor compounds including pyradines, thiazoles, pyrroles, pyrazines, imidazoles, and oxazoles; (3) the third stage, which includes the following reaction aldehyde-amine condensation, aldol condensation, and generation of nitrogen-containing compounds (Diez-Simon et al. 2019; Hodge 1953; van Boekel 2006).
Lipid Oxidation
A lipid is a group of important chemicals in food and food products. It plays an essential role in the price of products, texture, flavor, and the accessibility of food products by consumers (Diez-Simon et al. 2019). However, lipids can provide the foods with positive or negative qualities depending on the type of food in which it is responsible for rancid flavor in the milk (negative effect). They are considered the major flavor component (positive effect) (Diez-Simon et al. 2019). Fatty acids are subject to autoxidation to form hydroperoxides during food processing, such as heating, cooking, or frying, which further undergo decomposition and produce peroxyl and hydroxyl radicals (in general, oxidation of fatty acids occurs in three consecutive stages: initiation, propagation, and termination). Peroxyl and hydroxyl radicals will rapidly undergo cleavage in many routes to produce volatile and non-volatile compounds such as alcohols, ketones, aldehydes, acids, dimers, polymers, and epoxides (Frankel 1980; Diez-Simon et al. 2019).
Other Sources
Besides forming aroma compounds from Maillard reaction and lipid oxidation, some compounds, such as ascorbic acid, thiamine, and carotenoids, can play roles in developing aroma compounds through their chemical degradation during food processing. For instance, sulfur-containing compounds can be formed due to the degradation of thiamine, which contributes meaty aroma of cooked foods (Khan et al. 2015; Diez-Simon et al. 2019).
Aroma Analysis of Vinegar
The aroma is one of the important quality indicators of organoleptic properties. The aroma is a kind of volatile compound that contributes to odor products and can be sniffed by the nose through the olfactory receptors. While the other constitutes flavors that do not contribute to the odor, they can also mask or enhance other aroma compounds (Úbeda et al. 2019). Several factors influence the characterization of the aroma profile of vinegar, including the best selection for the extraction method, identification method, and quantitation method. In this part, extraction, identification, and quantitation of volatiles are discussed in detail as follows:
Several extraction methods have been used to extract and concentrate volatile compounds from the sample matrixes, including solvent-free extraction techniques and solvent extraction techniques. The first one includes several methods, such as solid-phase microextraction (SPME), dynamic headspace (DHS), static headspace (SHS), stir bar sorptive extraction (SBSE), and headspace sorptive extraction (HSSE). In contrast, the latter includes liquid–liquid extraction, solvent-assisted flavor evaporation (SAFE), and simultaneous distillation extraction (SDE) (Úbeda et al. 2019). Herein, the one standard method used by every group will be discussed in detail (i.e., solvent-free extraction techniques and solvent extraction techniques) as follows:
-
Solid-Phase Microextraction (SPME)
SPME is one of the common free-solvent methods used to extract volatiles from foods and beverages, in which it simply contains a “needle of a syringe-like device” to fix the coated fiber with a “thin layer of extraction phase” (Wardencki et al. 2004; Úbeda et al. 2019). In this method, the volatile compounds are extracted from the headspace (HS-SPME) of vinegar for a limited time at a specific temperature. The volatile compounds are absorbed into the fiber and then directly desorbed into the GC-O instrument in the injection port of GC at 250 °C. There are several types of extraction phases containing different polymers as follows, carboxen/polydimethylsiloxane (CAS/PDMS), polydimethylsiloxane (PDMS), divinylbenzene/polydimethylsiloxane (DVB/PDMS), and carboxen/divinylbenzene/polydimethylsiloxane (CAR/DVB/PDMS). Many previous researchers have used the SPME method to extract volatile and aroma-active compounds from vinegar (Al-Dalali et al. 2019a and 2019b; Al-Dalali et al. 2020a, b, c; Xiao et al. 2011; Zhou et al. 2017; Xiong et al. 2015; Yu et al. 2012; Aceña et al. 2011; Marín et al. 2002; Guerrero et al. 2008; Zhu et al. 2016; Mejías et al. 2002).
-
Solvent-Assisted Flavor Evaporation (SAFE)
SAFE is the best and most versatile method that can isolate the flavor compounds from different matrices without any side effects from other components in the matrix (i.e., amino, organic, and phenolic acids) (Engel and Schieberle 1999; Liang et al. 2016). This apparatus was developed by Engel and Schieberle (1999). It is a new and versatile technique used to isolate and extract the volatile and aroma-active compounds from several food matrixes. In the case of the liquid sample, it can be treated directly by SAFE and then extracted by the solvent. For a solid sample, the volatiles can be extracted from the samples by a solvent at first and then followed by SAFE.
SAFE apparatus contains a head and two legs. There are several propeller-shaped barriers inside the head, which are used to remove non-volatile substances from volatiles. Legs connect with two flasks, one of them is used to receive the sample (sample flask), and this flask keeps inside a water bath (40 °C), and the other flask is used to receive the distillate (distillate flask), and this flask keeps inside the liquid nitrogen. Besides, it contains two traps; one of them is a dropping funnel to the raw sample, and the other is a cooling trap to fill up with liquid nitrogen and connected with the vacuum pipe (in a range of 10−4 to 10−5 mbar by using a vacuum pump). This method’s advantage lies in its higher volatile yield and provides an extracted sample as close as its flavor to the natural product.
Identification of Volatile Flavor Compounds
Thousands of food volatiles have been identified since the invention of GC–MS coupling around 50 years ago. However, a thorough review of the literature reveals that many of these “identifications” are based only on assumptions based on fragmentation patterns acquired by mass spectrometric data, with no syntheses or NMR experiments done in many cases. Furthermore, headspace isolation methods such as SPME have made the volatile separation procedure easier. However, as many writers have done, combining SPME isolation with mass spectrometric identifications based only on commercial databases may be a source of mistakes or called tentative identification. Only volatiles that interact with receptor proteins in the human olfactory bulb are responsible for a specific aroma. Suppose the research objective is to determine the aroma or changes in the aroma. In that case, the identification studies should focus on the odor-active volatiles using GC-O as described above (Molyneux & Schieberle 2007).
For accurate identification, separating volatile compounds is performed on capillary columns, particularly by two different columns in different polarities, such as HP-5 and DB-Wax. First, separated chemicals in the chromatogram (total ion chromatogram, TIC) are tentatively identified after matching their mass spectra (MS) with those found in the National Institute of Standard and Technology library (NIST). Meanwhile, those compounds’ retention index (RI) is calculated based on a mixture of n-alkane (C6-C28) and then compared with those standards conducted by GC–MS or GC-FID under the same condition for the samples. The RI is calculated by Eq. 1 (Liu et al. 2018):
where RI is the retention index; T is the retention time of peak (compound) in the sample; Tn−1 is the retention time of n-alkane that has RT lower than that for the peak; Tn+1 is the retention time of n-alkane that has RT higher than that for the peak; and n is the number of carbon in n-alkane.
The third method for the identification of flavor compounds relies on standard compounds. After tentative identification by MS and RI, the authentic standards of those temporarily identified compounds will be used to confirm the identification (positively identification). The standard chemicals are injected into the GC–MS under the same conditions as the samples. Their RI values and MS spectra are compared with those of the temporarily identified peaks in the sample. The fourth identification method depends on matching the aroma description of the standard compounds with those of the separated compounds by GC-O. This method generally is used to characterize aroma-active compounds (Al-Dalali et al. 2019a and 2019b; Al-Dalali et al. 2020a; Aili et al. 2011).
Molyneux and Schieberle (2007) reported that to minimize making a mistake, use the following technique to precisely identify volatiles or aroma compounds:
-
(1) First, mass spectra (MS-electron ionization; MS-chemical ionization) and retention indices must be calculated on at least two separate GC columns with different polarity stationary phases.
-
(2) The results must be compared to reference compound spectra and retention indices.
-
(3) The retention indices of both enantiomers of chiral substances must be assessed using the corresponding enantiomers.
-
(4) Odor characteristics or odor thresholds established by GC-O may be useful tools for identifying compounds.
Quantitation of Volatile Flavor Compounds
The concentration of identified volatile compounds in the food products depends on comparing the GC signal (IOFI 2011). It depends on the signal of known standard concentration in the external standard method or standard addition method or the known concentration of isotope compound in the stable isotope dilution assay method (SIDA).
There are several methods used in the quantitation and semi-quantitation of volatile compounds that will explain in detail below:
-
1. External Standard Method
In this method, the obtained results represent the real concentration of quantified compounds. This quantitation method can be done in solvent or free-solvent extraction. The first one is in solvent extraction of the sample (i.e., SAFE or liquid–liquid extraction), and the latter is extracted volatile from headspace such as SPME. Generally, several descending concentrations are prepared for the targeted compounds with or without IS. After injecting the standard solution either in GC–MS or GC-FID (3 times/one concentration), a linear calibration curve is constructed based on the ratio of the concentration of compounds and their peak area (PA) in the case of the standard solution without IS or based on the ratio of the concentration of compounds to the concentration of IS (X-axis) and the ratio of peak area of standard compounds to the peak area of IS (Y-axis) in case of standard solution with IS (IOFI 2011).
Finally, analyte concentrations can be calculated from the calibration line or the calibration equation extracted from the calibration curve. Otherwise, it can also be calculated from the single-point calibration by using Eq. 2:
where “Ca is the concentration of analytes in the sample; Cs is the concentration of standard compound; Aa is the peak area of analytes in the sample; and As is the peak area of the standard compound.”
Besides, there is another calculation for the concentration of analytes in this method depending on the calculation of response factor (RF) as follows (Eq. 3):
where As is the peak area of one single standard concentration and Cs is the known standard concentration.
Then, the RF is calculated for all prepared standard concentrations (5–6 points), and after that, the mean of RFs is calculated. Finally, to calculate the concentration of analytes in the sample, Eq. 4 can be used:
Ca is the concentration of analytes in the sample; Aa is the peak area of analytes in the sample, and RFmean is the mean of the response factor (Sparkman et al. 2011).
-
2. Internal Standard Method
It is a semi-quantitation and straightforward method. In this method, a pure IS compound that should not have existed naturally in the sample and be eluted in a different retention time is added in a known amount to the sample before the extraction. Then, the concentration of analytes can be calculated by Eq. 5:
where “Ca is the concentration of analytes in the sample; Aa is the peak area of analytes in the sample; Ais is the peak area of IS in the sample; Cis is the known concentration of IS in the sample.”
-
3. Stable Isotope Dilution Assay Method (SIDA)
This method is considered one of the internal standardization methods, more accurate than the external method. However, it is expensive, and also, some isotope-labeled compounds are not commercially available. These compounds must be similar in their structure and physicochemical properties (polarity and boiling point) to those present in the samples (IOFI 1997), in which these compounds can be added to a sample in a known concentration plus IS before extraction of volatile compounds or in nominal concentrations in a ratio of the standard: internal standard as follows, 1:5; 1:3; 1:1; 3:1, and 5:1, and the concentration of the analytes can be calculated by the following equations:
At first, the relative response factor is calculated by Eq. 6:
where As is the peak area of the standard, Ais is the peak area of IS, Cis is the amount of IS added to the standard solution, and Cs is the amount of standard in the standard solution.
Second, the concentration of analytes in the sample can be calculated by Eq. 7:
where Ca is the concentration of analytes in the sample, Aa is the peak area of analytes in the sample, Ais is the peak area of IS added to the sample, and Cis is the amount of IS added to the sample (IOFI 2011; León et al. 2020).
-
4. Calculation of Corrected Concentration
It is a simple method and depends on the preparation of the standard compounds in one concentration. For example, all the standard compounds and the IS are mixed at 20 mg/kg in 10 mL of suitable solution (7% of acetic acid for vinegar sample) for SPME extraction (Al-Dalali et al. 2020a). Or by mixing 1 µL of all the standard compounds and IS in 1 mL of n-hexane solvent in the case of direct injection (Liu et al. 2018), this solution is called the standard solution. Besides, the exact volume of IS is added to the sample to get the sample solution. Then, the two solutions are analyzed by GC–MS under the same conditions. After extraction of the peak areas of compounds in both solutions, the corrected concentration is calculated by applying three equations as follows:
“Where \({f}^{^{\prime}}\) the correction factor, C1 is the concentration of the authentic chemical in the standard solution, A1 is the peak area of the authentic chemical in the standard solution, Cs is the concentration of the IS in the standard solution, As is the peak area of the IS in the standard solution, Ći is the concentration of the compound in the sample solution, Ai is the peak area of the compound in the sample solution, Ás is the peak area of the IS in the sample solution, Ćs is the concentration of the compound in the sample solution, and Ci is the corrected concentration of the compound” (Al-Dalali et al. 2020a, b, c).
Furthermore, other methods are also used to express analyte concentration or their percentages, such as the standard addition and internal normalization methods.
Conclusions
Vinegar is an acidic solution commercially processed through three phases: saccharification of carbohydrates, fermentation of alcohol, and acetic acid fermentation. Alcoholic fermentation of Chinese vinegar could be conducted by either Qu in SSF or by yeast in LSF. At the same time, acetification is conducted by acetic acid bacteria (i.e., vinegar Pei) of cereal food. As a result of the increasing demand for agricultural products, there is a surplus in these products. Their waste causes environmental pollution, and those wastes can be used as raw materials and converted to other fermented products like vinegar. Nowadays, vinegar fermentation can be done by the traditional method or by the modern method. The main component in vinegar is acetic acid, other organic acids, and other volatile flavor chemical groups such as esters, ketones, aldehydes, and pyrazines. These chemicals are considered the main contributors to Chinese vinegar’s aroma profiles. However, every type of Chinese vinegar has its unique aroma and taste, which are affected by its individual distinct process steps during the fermentation, raw materials, type of starter, and fermentation technique used.
Change history
28 November 2022
A Correction to this paper has been published: https://doi.org/10.1007/s12161-022-02428-7
References
Aceña L, Vera L, Guasch J, Busto O, Mestres M (2011) Chemical characterization of commercial Sherry vinegar aroma by headspace solid-phase microextraction and gas chromatography-olfactometry. J Agric Food Chem 59:4062–4070
Aili W, Huanlu S, Changzhong R, Zaigui L (2011) Key aroma compounds in Shanxi aged tartary buckwheat vinegar and changes during its thermal processing. Flavour Fragr J 27(1):47–53
Al-Dalali S, Zheng F, Li H, Huang M, Chen F (2019a) Characterization of volatile compounds in three commercial Chinese vinegars by SPME-GC-MS and GC-O. LWT-Food Sci Technol 112:108264. https://doi.org/10.1016/j.lwt.2019.108264
Al-Dalali S, Zheng F, Sun B, Chen F (2019b) Comparison of aroma profiles of traditional and modern Zhenjiang aromatic vinegars and their changes during the vinegar aging by SPME-GC-MS and GC-O. Food Anal Methods 12(2):544–557
Al-Dalali S, Zheng F, Sun B, Chen F (2020a) Characterization and comparison of aroma profiles and aroma-active compounds between traditional and modern Sichuan vinegars by Molecular sensory science. J Agric Food Chem 68(18):5154–5167. https://doi.org/10.1021/acs.jafc.0c00470
Al-Dalali S, Zheng F, Sun B, Chen F, Wang P, Wang W (2020b) Determination of the aroma changes of Zhengrong vinegar during different processing steps by SPME-GC-MS and GC-O. J Food Meas Charact 14:535–547. https://doi.org/10.1007/s11694-019-00298-y
Al-Dalali S, Zheng F, Sun B, Zhou C, Li M, Chen F (2020c) Effects of different brewing processes on the volatile flavor profiles of Chinese vinegar determined by HS-SPME-AEDA with GC-MS and GC-O. LWT-Food Sci Technol 133:109969. https://doi.org/10.1016/j.lwt.2020.109969
Al-Dalali S, Zheng F, Sun B, Rahman T, Chen F (2022) Tracking volatile flavor changes during two years of aging of Chinese vinegar by HS-SPME-GC-MS and GC-O. J Food Compos Anal 106:104295. https://doi.org/10.1016/j.jfca.2021.104295
Boonsupa W, Chumchuere S, Chaovarat M (2017) Evolution of volatile compounds generated during one-stage and two-stage fermentation and aging processes of banana vinegars using HS-SPME-GC-MS. Biotechnol: Indian J 13(5):147.
Bruna-Maynou FJ, Castro R, Rodríguez-Dodero MC, Barroso CG, Durán-Guerrero E (2020) Flavored Sherry vinegar with citric notes: characterization and effect of ultrasound in the maceration of orange peels. Food Res Int. https://doi.org/10.1016/j.foodres.2020.109165.
Budak NH, Aykin E, Seydim AC, Greene AK, Guzel-Seydim ZB (2014) Functional properties of vinegar. J of Food Science 79(5):757–764
Callejón RM, Morales ML, Ferreira ACS, Troncoso AM (2008) Defining the typical aroma of Sherry vinegar: sensory and chemical approach. J Agric Food Chem 56:8086–8095
Callejón RM, Tesfaye W, Torija MJ, Mas A, Troncoso AM, Morales ML (2009) Volatile compounds in red wine vinegars obtained by submerged and surface acetification in different woods. Food Chem 113:1252–1259
Callejón RM, Torija MJ, Mas A, Morales ML, Troncoso AM (2010) Changes of volatile compounds in wine vinegars during their elaboration in barrels made from different woods. Food Chem 120(2):561–571
Canning A (1985) Vinegar Brewing. J Food Sci, 20–21.
Cejudo-Bastante C, Durán-Guerrero E, García-Barroso C, Castro-Mejías R (2018) Comparative study of submerged and surface culture acetification process for orange vinegar. J Sci Food Agric 98:1052–1060
Chan E, Ahmed JM, Wang M, Chan JC (1994) History of medicine and nephrology in Asia. Am J Nephrol 14:295–301
Chanivet M, Durán-Guerrero E, Barroso CG, Castro R (2020) Suitability of alternative wood types other than American oak wood for the ageing of Sherry vinegar. Food Chem 126386. https://doi.org/10.1016/j.foodchem.2020.126386.
Charles M, Martin B, Ginies C, Etievant P, Coste G, Guichard E (2000) Potent aroma compounds of two red wine vinegars. J Agric Food Chem 48:70–77
Chen ZF (1999) Vinegar. In: Wang FY (ed) Modern food fermentation technology. China Light Industry Press, Beijing, pp 506–566
Chen T, Gui Q, Shi JJ, Zhang XY, Chen FS (2013) Analysis of variation of main components during aging process of Shanxi aged vinegar. Acetic Acid Bact 2:31–38
Chen F, Li L, Qu J, Chen C (2009) Cereal vinegars made by solid-state fermentation in China. In: Solieri L, Giudici P (ed) Vinegars of the world. Springer-Verlag, Italia, Chapter 15, pp. 243–259
Chinnici F, Guerrero ED, Sonni F, Natali N, Marín RN, Riponi C (2009) Gas chromatography-mass spectrometry (GC-MS) characterization of volatile compounds in quality vinegars with protected European geographical indication. J Agric Food Chem 57:4784–4792
Chung N, Jo Y, Joe MH, Jeong MH, Jeong YJ, Kwon JH (2017) Rice vinegars of different origins: discriminative characteristics based on solid-phase microextraction and gas chromatography with mass spectrometry, an electronic nose, electronic tongue and sensory evaluation. J Ins Brew 123(1):159–166
Chunju L, Haiou W, Dajing L, Chunquan L (2015a) Effects of vinegar pickling and drying on the volatile flavor compounds of garlic. J Nuc Agric Sci 29(9):1743–1748 (In Chinese language)
Chunju L, Haiou W, Dajing L, Chunquan L (2015b) Analyse of volatile flavor compounds in different faba beans treated with vinegar-pickling and drying. Food Ferment Ind 41(2):135–140 (In Chinese language)
Conner HA, Allgeier RJ (1976) Vinegar: its history and development. Adv Appl Microbiolo 81–133.
Corsini L, Castro R, Barroso CG, Durán-Guerrero E (2019) Characterization by gas chromatography-olfactometry of the most odour-active compounds in Italian balsamic vinegars with geographical indication. Food Chem 272:702–708
Dan H, Youqing L, Yue N, Junmo O, Hua Y, Mengting S (2016) Study of main aroma components of Sichuan bran vinegar based on ROAV value. Food Indu 37(9):288–292 (In Chinese language)
Del Signore A (2001) Chemometric analysis and volatile compounds of traditional balsamic vinegars from Modena. J Food Eng 50(2):77–90
Diez-Simon C, Mumm R, Hall RD (2019) Mass spectrometry-based metabolomics of volatiles as a new tool for understanding aroma and flavour chemistry in processed food products. Metabolomics 15(3). https://doi.org/10.1007/s11306-019-1493-6.
Dong D, Zheng W, Jiao L, Lang Y, Zhao X (2016) Chinese vinegar classification via volatiles using long-optical-path infrared spectroscopy and chemometrics. Food Chem 194:95–100
Engel W, Bahr W, Schieberle P (1999) Solvent assisted flavour evaporation ± a new and versatile technique for the careful and direct isolation of aroma compounds from complex food matrices. Eur Food Res Technol 209:237–241
Frankel EN (1980) Lipid oxidation. Prog Lipid Res 19(1–2):1–22
Fuxiu Y, Kaowen Z (2019) Analysis of volatile flavor components in water tower vinegar by headspace solid phase microextraction and GC-MS. Food Sci. https://doi.org/10.7506/spkx1002-6630-20190727-361. (In Chinese language).
Garcia-Parilla MC, Gonzalez GA, Heredia FJ, Troncoso AM (1997) Differentiation of wine vinegars based on phenolic composition. J Agric Food Chem 45:3487–3492
Garcia-Parrilla MC, Torija MJ, Mas A, Cerezo AB, Troncoso AM (2017) Vinegars and other fermented condiments. In Frias J, Martinez-Villaluenga C, Peñas E (eds). Fermented Foods in Health and Disease Prevention. chapter 25. Elsevier Inc.
Guerrero ED, Marín RN, Mejías RC, Barroso CG (2007) Stir bar sorptive extraction of volatile compounds in vinegar: validation study and comparison with solid phase microextraction. J Chromatogra A 1167(1):18–26
Guerrero ED, Chinnici F, Natali N, Marín RN, Riponi C (2008) Solid-phase extraction method for determination of volatile compounds in traditional balsamic vinegar. J Sep Sci 31:3030–3036
GuiQiu L, Quan L, Chun-Xia L, Jing-Jing W, Fei L, Geng-Sheng X, Xiao-Ling Z (2012) Analysis of volatile components in mulberry fruit vinegar by headspace solid-phase micro-extraction coupled with GC-MS. Sci Sericulture 38(6):1137–1141 (In Chinese language)
Ho CW, Lazim AM, Fazry S, Zaki UKHH, Lim SJ (2017) Varieties, production, composition and health benefits of vinegars: a review. Food Chem 221:1621–1630
Hodge JE (1953) Dehydrated foods, chemistry of browning reactions in model systems. J Agric Food Chem 1(15):928–943
Hongmei H, Shengwan Z, Caixia G, Meiping L (2016) Analysis of volatile components in Hawthorn vinegar by headspace solid-phase microextraction-gas chromatography-mass spectrometry (HS-SPME-GC-MS). Food Sci 37(2):138–141 (In Chinese language)
Hongwen L, Xuping W, Xiaolan Y (2015) Influence of different fumigation processes on aroma compounds of Shanxi aged vinegar. Food Sci 36(20):90–94 (In Chinese language)
Hu JP (2005) Literary history of production technology of vinegar. Chinese Condiment 9:10–12 (In Chinese language)
Huaimin Z, Guofeng D, Jia C, Rufu W (2017) Analysis of volatile components in metamorphic vinegar based on SPME-GC-MS and HS-GC-MS. Chinese Condiment 42(1):31–36 (In Chinese language)
Huang ZH, Cai MZ (1998) Vinegar. In Technology for fermented Condiments, Shanghai Institute of Brewing Science., Eds.; China Light Industry Press, Beijing, pp. 289–423.
IOFI (2011) Guidelines for the quantitative gas chromatography of volatile flavouring substances, from the Working Group on Methods of Analysis of the International Organization of the Flavor Industry (IOFI). Flavour Fragr J 26:297–299
IOFI (1997) Analytical procedure for a general quantitative method using coupled capillary gas chromatography/mass spectroscopy with selected ion monitoring (SIM), Z. Lebensm.-Unters.-Forsch 204, 395.
Jaeger H, Janositz A, Knorr D (2010) The Maillard reaction and its control during food processing The potential of emerging technologies. Pathologie Biologie 58(3):207–213
Jihong Z, Yongmao Z, Chaozhen Z, Sanjiang K, Haiyan Z (2013) GC-MS analysis of volatile compounds in fermented Apple vinegar. Liquor-Making Sci Technol 5:94–96 (In Chinese language)
Jin W, Yu C, Wang Z-Y, Yue Y-D, Feng S (2011) Comparison of two sample pretreatment methods for volatile composition analysis of Bamboo vinegar. Food Sci 32(18):198–201 (In Chinese language)
Johnston CS, Gaas CA (2006) Vinegar: medicinal uses and antiglycemic effect. MedGenMed: Medscape general medicine 8(2): 61.
Jones DD, Greenshields RN (1969) Volatile constituents of vinegar. I. A survey of some commercially available malt vinegars. J Inst Brew 75(5): 457–463.
Khan MI, Jo C, Tariq MR (2015) Meat flavor precursors and factors influencing flavor precursors-A systematic review. Meat Sci 110:278–284
Lazim AM, Lim SJ, Ho CW, Fazry S (2019) Types of vinegars. In Bekatorou A, Raton B (eds). Advances in vinegar production, CRC Press, Taylor and Francis, Chapter 2, 1st Edition.
León CSD, Ortíz DKR, González DFJ (2020) Sensory approach and chiral analysis for determination of odour active compounds from feijoa (Acca sellowiana). Food Chem 126383. https://doi.org/10.1016/j.foodchem.2020.126383.
Leonés A, Durán-Guerrero E, Carbú M, Cantoral JM, Barroso CG, Castro R (2018) Development of vinegar obtained from lemon juice: optimization and chemical characterization of the process. LWT-Food Sci Technol. https://doi.org/10.1016/j.lwt.2018.10.096.
Li Z, Tan H (2009) Traditional Chinese foods: production and research progress. Nova Science Publishers Inc., New York, USA
Li Y, Ruichang G, Xuexia G (2011) Using different sampling methods to determine volatile components of beverage. Chinese Condiment 12(36):92–94 (In Chinese language)
Li S, Li P, Liu X, Luo L, Lin W (2016) Bacterial dynamics and metabolite changes in solid-state acetic acid fermentation of Shanxi aged vinegar. Appl Microbiol Biotechnol 100(10):4395–4411
Liang J, Xie J, Hou L, Zhao M, Zhao J, Cheng J, Wang S, Sun BG (2016) Aroma constituents in shanxi aged vinegar before and after Aging. J Agric Food Chem 64(40):7597–7605
Lianhua X, Lu Y, Quanzeng W, Fuping L, Hongbin W (2013) Comparison of the flavor compounds of aged vinegar from the ageing jar of the Qing dynasty. China Brew 32(9):32–37 (In Chinese language)
Lim SJ, Ho CW, Lazim AM, Fazry S (2019) History and current issues of vinegar. In Bekatorou A (eds). Advances in vinegar production. Chapter 1, pp. 1–17, Boca Raton, CRC Press, Taylor and Francis, 1st Edition.
Limin Q, Gengsheng Z, Jijun W, Yujuan X, Daobang T, Weijie Y (2012) Effect on volatile aromatic components in litchi vinegar of three different fermentation ways. China Brew 31(4):106–110 (In Chinese language)
Liu DR, Zhu Y, Beeftink R, Ooijkaas L, Rinzema A, Chen J, Tramper J (2004) Chinese vinegar and its solid-state fermentation process. Food Rev Int 20:407–424
Liu S, Zhang D, Chen J (2014) History of solid state fermented foods and beverages. In: Chen J, Zhu Y (eds) Solid state fermentation for foods and beverages. CRC Press, Boca Raton, FI, pp 59–118
Liu J, Gan J, Yu Y, Zhu S, Yin L, Cheng Y (2016) Effect of laboratory-scale decoction on the antioxidative activity of Zhenjiang aromatic vinegar: the contribution of melanoidins. J of Functional Foods 21:75–86
Liu Y, He C, Song H (2018) Comparison of SPME versus SAFE processes for the analysis of flavor compounds in Watermelon juice. Food Anal Methods 11(6):1677–1689
Liu J (1982) Fujian Yunchun old vinegar. China Brew 1: 30-31. (In Chinese language).
Lu ZM, Xu W, Yu NH, Zhou T, Li GQ, Shi JS, Xu ZH (2011) Recovery of aroma compounds from Zhenjiang aromatic vinegar by supercritical fluid extraction. Int J Food Sci Technol 46:1508–1514
Maillard LC (1912) Action des acides amines sur les sucres: Formation des melanoidines par voie methodique. Comptes Rendus Hebdomadaires Des Seances De L’academie Des Sciences 154:66–68
Marín RN, Mejías RC, Moreno MVG (2002) Headspace solid-phase microextraction analysis of aroma compounds in vinegar “Validation study.” J Chromatogra A 967:261–267
Mas A, Torija MJ, García-Parrilla MC, Troncoso AM (2014) Acetic acid bacteria and the production and quality of wine vinegar. Sci World J 1–6.
Mazza S, Murooka Y (2009) Vinegars through the ages. Vinegars of the World 17–39.
Mejías RC, Marín RN, Moreno MVG (2002) Optimisation of headspace solid-phase microextraction for analysis of aromatic compounds in vinegar. J Chromatogr A 953:7–15
Molyneux RJ, Schieberle P (2007) Compound identification: a journal of agricultural and food chemistry perspective. J Agric Food Chem 55(12):4625–4629. https://doi.org/10.1021/jf070242j
Morales ML, González AG, Casas JA, Troncoso AM (2001) Multivariate analysis of commercial and laboratory produced Sherry wine vinegars: influence of acetification and aging. Eur Food Res Technol 212:676–682
Natera R, Castro R, Garciäa-moreno MDV, Ndez MJH, Garciäa-Barroso C (2003) Chemometric studies of vinegars from different raw materials and processes of production. J Agric Food Chem 51:3345–3351
Ozturk I, Caliskan O, Tornuk F, Ozcan N, Yalcin H, Baslar M, Sagdic O (2015) Antioxidant, antimicrobial, mineral, volatile, physicochemical and microbiological characteristics of traditional home-made Turkish vinegars. LWT-Food Sci Technol 603:141–151
Palacios V, Valcárcel M, Caro I, Pérez L (2002) Chemical and biochemical transformations during the industrial process of Sherry vinegar aging. J Agric Food Chem 50:4221–4225
Pizarro C, Esteban-Díez I, Sáenz-González C, González-Sáiz JM (2008) Vinegar classification based on feature extraction and selection from headspace solid-phase microextraction/gas chromatography volatile analyses: a feasibility study. Anal Chim Acta 608(1):38–47
Plutowska B, Wardencki W (2008) Application of gas chromatography-olfactometry (GC-O) in analysis and quality assessment of alcoholic beverages-A review. Food Chem 107(1):449–463
Qiang Z, Xiu-lan X, Fu-min Y, Liang C (2015) Evaluation of the relative odor activity value in red raspberry fruit vinegar by principal component analysis. Modern Food Sci Technol 31(11):332–338 (In Chinese language)
Qing L, Senmiao T, Jianyi M (2014) Volatile compounds from bamboo vinegar with HS-SPME and GC-MS. J Zhejiang A & F Uni 31(2):308–314 (In Chinese language)
Qing-hui H, Jian-min Y, Yong-qiang S, Yu W (2012) Changes of volatile composition during fumigating process of Liangzhou fumigated vinegar. Sci Technol Food Ind 33(22):146–151 (In Chinese language)
Ríos-Reina R, Segura-Borrego MP, García-González DL, Morales ML, Callejón RMA (2019) A comparative study of the volatile profile of wine vinegars with protected designation of origin by headspace stir bar sorptive extraction. Food Res Int 123:298–310
Sant'Ana ADS (2016) Microbial ecology of cereal and cereal-based foods, John Wiley & Sons, Ltd.
Schreier P (1979) Flavor-composition-of-wines-a-review. Crit Rev Food Sci Nutr 12:59–111
Shahidi F, Samaranayaka AGP, Pegg RP (2014) Maillard reaction and browning. Encyclopedia of meat sciences, (Vol. 1). Amsterdam: Elsevier. https://doi.org/10.1016/B978-0-12-384731-7.00130 -6.
Shang Y (2003) The traditional processing technology of Langzhong Baoning vinegar. Chin Brew 6:32–33 (In Chinese language)
Shen ZY (2007) Zhenjiang aromatic vinegar. Hong Kong Medical Press, Hong Kong
Sparkman OD, Penton ZE, Kitson FG (2011) Gas chromatography and mass spectrometry: a practical guide. Academic press in an imprint of Elsevier, Second edition.
Steinkraus KH (2004) Handbook of indigenous fermented foods, Marcel Dekker, New York. Subsidiary Food 2nd edn, 21: 1–4.
Tamanna N, Mahmood N (2015) Food processing and Maillard reaction products: effect on human health and nutrition. Int J Food Sci Technol. https://doi.org/10.1155/2015/52676 2.
Ubeda C, Callejón RM, Troncoso AM, Moreno-Rojas JM, Peña F, Morales ML (2012) Characterization of odour active compounds in strawberry vinegars. Flavour Fragrance J 27:313–321
Ubeda C, Callejón RM, Troncoso AM, Moreno-Rojas JM, Peña F, Morales ML (2016) A comparative study on aromatic profiles of strawberry vinegars obtained using different conditions in the production process. Food Chem 192:1050–1059
Úbeda C, Ríos-Reina R, Segura-Borrego MP, Callejón RM, Morales ML (2019) Vinegar aroma profile and analysis. In Bekatorou A, Raton B (eds). Advances in vinegar production. CRC Press, Taylor and Francis, Chapter 17, 1st Edition.
van Boekel MAJS (2006) Formation of flavour compounds in the Maillard reaction. Biotechnol Advances 24(2):230–233
Wang A, Zhang J, Li Z (2012) Correlation of volatile and nonvolatile components with the total antioxidant capacity of tartary buckwheat vinegar: influence of the thermal processing. Food Res Int 49(1):65–71
Wang ZM, Lu ZM, Yu YJ, Li GQ, Shi JS, Xu ZH (2015) Batch-to-batch uniformity of bacterial community succession and flavor formation in the fermentation of Zhenjiang aromatic vinegar. Food Microbiol 50:64–69
Wang ZM, Lu ZM, Shi JS, Xu ZH (2016) Exploring flavour-producing core microbiota in multispecies solid-state fermentation of traditional Chinese vinegar. Sci Reports 6(1). https://doi.org/10.1038/srep26818.
Wardencki W, Michulec M, Curylo J (2004) A review of theoretical and practical aspects of solid-phase microextraction in food analysis. Int J Food Sci Technol 39:703–717
Weiqing L, Liting WHJ, Li L (2011) Determination of volatile aroma compounds in litchi vinegar by HS-SPME-GC/MS. China Brew 12:160–162 (In Chinese language)
Wenxiu W, Lin L, Xin J, Xinpeng D, Jianfeng S, Jie W, Yankun P, Yutaka K (2019) Quality change law of vinegar during automatic mechanical fermentation. Trans Chinese Soci Agric Eng 35(18):273–281 (In Chinese language)
Xia S, Pang Y, Liu S (1985) The dynamic analysis of the components of vinegar during its fermentation course. Chinese Condiment 6:5–6 (In Chinese language)
Xia T, Zhang B, Duan W, Zhang J, Wang M (2019) Nutrients and bioactive components from vinegar: a fermented and functional food. J Funct Foods 103681. https://doi.org/10.1016/j.jff.2019.103681.
Xiao ZB, Dai SP, Niu YW, Yu HY, Zhu JC, Tian HX, Gu YB (2011) Discrimination of Chinese vinegars based on headspace solid-phase microextraction-gas chromatography mass spectrometry of volatile compounds and multivariate analysis. J Food Sci 76:C1125–C1135
Xiaoqing X, Shuxian L, Erihemu, (2017) Identification of different brands of vinegar by electronic nose. Sci Technol Food Indu 38(09):277–284 (In Chinese language)
Xie X, Zheng Y, Liu X, Cheng C, Zhang X, Xia T, Wang M (2017) Antioxidant activity of Chinese Shanxi aged vinegar and its correlation with polyphenols and flavonoids during the brewing process. J Food Sci 82(10):2479–2486
Xin L, Xiao-yan Z, Shao-hua W, Sheng-rong G, Tao L, Guang-quan X (2014) Fermentation technology of red globe Grape vinegar and analyses of volatile aroma components. Hubei Agric Sci 53(14):3370–3372 (In Chinese language)
Xiong C, Zheng Y, Xing Y, Chen S, Zeng Y (2015) Discrimination of two kinds of geographical origin protected Chinese vinegars using the characteristics of aroma compounds and multivariate statistical analysis. Food Anal Methods 9(3):768–776
Xiuli L, Ying Y, Shengmin L, Chenlei Z (2016) Effect of fermentation methods on sensory and nutritional quality of Persimmon vinegar. Food Sci 37(19):154–159 (In Chinese language)
XiWen Z, Sha L, XiaoTu C (2019) Analysis and study on volatile components of 6 kinds of vinegar. Food Saf Quality Detect Technol 10(13):4429–4437 (In Chinese language)
Xu W, Xu Q, Chen J, Lu Z, Xia R, Li G, Ma Y (2011) Ligustrazine formation in Zhenjiang aromatic vinegar: changes during fermentation and storing process. J Sci Food Agric 91(9):1612–1617
Xu Y, Wang D, Fan WL, Mu XQ, Chen J (2009) Traditional Chinese biotechnology. In: Tsao G, Ouyang P, Chen J (ed) Biotechnology in China II. Advances in biochemical engineering / biotechnology, vol 122. Springer, Berlin, Heidelberg, pp. 189–233
Yijie Z, Yun G, Yonggang Q, Shiyao L, Ye B, Yang C, Bing G (2016) Analysis of volatile components in Watermelon vinegar by different fermentation process. China Brew 35(1):156–160 (In Chinese language)
Youlan S, Yongguang H, Dongya T, Xingqin W (2019) Analysis of flavor characteristics of vinegar brewed by honeysuckle tail liquor based on odor activity value and sensory attributes. Food Ferment Indust 45(15):233–241 (In Chinese language)
Yu YJ, Lu ZM, Yu NH, Xu W, Li GQ, Shi JS, Xu ZH (2012) HS-SPME/GC-MS and chemometrics for volatile composition of Chinese traditional aromatic vinegar in the Zhenjiang region. J Inst Brew 118:133–141
Yuan L, Junkui L, Zongbao S, Junling Y, Bo C (2019) Comparison of volatile compounds in Zhenjiang aromatic vinegar produced by traditional handicrafts and modern industrial technology with different ageing time. Food Industry 40(7):34–38 (In Chinese language)
Yue X, Zhi-fei H, Hong-jun L, Gang Q, Ting W (2011) Determination of volatile compounds in Sichuan bran vinegars using head space-solid phase micro-extraction coupled with gas chromatography-mass spectrometry. Food sci. (In Chinese language).
Zhang X, Wang P, Xu D, Wang W, Zhao Y (2019) Aroma patterns of Beijing rice vinegar and their potential biomarker for traditional Chinese cereal vinegars. Food Res Int. https://doi.org/10.1016/j.foodres.2019.02.008.
Zhao DA (2004) Function, origin, technology and quality of vinegar. Jiangsu Condiment and Subsidiary Food 21:1–4 (In Chinese language)
Zhao LQ, Li L (2005) The history, present status, development trend of the production technology of Chinese vinegar. Chinese Condiment 1:3–6 (In Chinese language)
Zhao C, Xia T, Du P, Duan W, Zhang B, Zhang J, Zhu S, Zheng Y, Wang M, Yu Y (2018) Chemical composition and antioxidant characteristic of traditional and industrial Zhenjiang aromatic vinegars during the aging process. Molecules. https://doi.org/10.3390/molecules23112949.
Zhao G, Kuang G, Li J, Hadiatullah H, Chen Z, Wang X, Wang Y (2020) Characterization of aldehydes and hydroxy acids as the main contribution to the traditional Chinese rose vinegar by flavor and taste analyses. Food Res Int 108879. https://doi.org/10.1016/j.foodres.2019.108879.
Zhencheng L, Li GH, Zhang B-S, Fen L (2011) Separation and identification of volatile compounds in Leechee vinegar. Food Ferment Indust 37(8):170–174 (In Chinese language)
Zheng-dan Z, Hua-chang W, Jing D, Shang-chun Z, Yang L, Yu-bin L, Jia-lu G, Jian-jun G (2015) Identification and study of fermentation metabolites of aroma-producing yeast strains from Baoning vinegar. Sci Technol Food Indust 24:206–211 (In Chinese language)
Zhou Z, Liua S, Konga X, Jia Z, Hana X, Wu J, Mao J (2017) Elucidation of the aroma compositions of Zhenjiang aromatic vinegar using comprehensive two dimensional gas chromatography coupled to time-of-flight mass spectrometry and gas chromatography-olfactometry. J Chromatogr A 1487:218–226
Zhu H, Zhu J, Wang LL, Li ZG (2016) Development of a SPMEGC-MS method for the determination of volatile compounds in Shanxi aged vinegar and its analytical characterization by aroma wheel. J Food Sci Technol 53:171–183
Zhu Y, Zhang F, Zhang C, Yang L, Fan G, Xu Y, Sun B, Li X (2018) Dynamic microbial succession of Shanxi aged vinegar and its correlation with flavor metabolites during different stages of acetic acid fermentation. Sci Reports 8:8612. https://doi.org/10.1038/s41598-018-26787-6
Funding
This work was financially supported by the National Key R&D Program of China (2016YFD0400500) under the Ministry of Science and Technology of the People’s Republic of China, and Fundamental Research Funds for the Central Universities of China (Grant No. JZ2021HGQB0277).
Author information
Authors and Affiliations
Corresponding authors
Ethics declarations
Conflict of Interest
Sam Al-Dalali declares that he has no conflict of interest. Fuping Zheng declares that he has no conflict of interest. Baocai Xu declares that he has no conflict of interest. Mahmoud Abughoush declares that he has no conflict of interest. Lianghao Li declares that he has no conflict of interest. Baoguo Sun declares that he has no conflict of interest.
Ethics Approval
This article does not contain any studies with human participants or animals performed by any of the authors.
Consent to Participate
Not applicable.
Additional information
Publisher's Note
Springer Nature remains neutral with regard to jurisdictional claims in published maps and institutional affiliations.
The original online version of this article was revised: In this article the affiliation 'Department of Clinical Nutrition and Dietetics, Faculty of Applied Medical Sciences, The Hashemite University, Zarqa 13133, Jordan' for Mahmoud Abughoush was missing.
Rights and permissions
Springer Nature or its licensor (e.g. a society or other partner) holds exclusive rights to this article under a publishing agreement with the author(s) or other rightsholder(s); author self-archiving of the accepted manuscript version of this article is solely governed by the terms of such publishing agreement and applicable law.
About this article
Cite this article
Al-Dalali, S., Zheng, F., Xu, B. et al. Processing Technologies and Flavor Analysis of Chinese Cereal Vinegar: a Comprehensive Review. Food Anal. Methods 16, 1–28 (2023). https://doi.org/10.1007/s12161-022-02328-w
Received:
Accepted:
Published:
Issue Date:
DOI: https://doi.org/10.1007/s12161-022-02328-w