Abstract
In this study, an integrative approach to produce biohydrogen (H2) and polyhydroxyalkanoates (PHA) from the wastes of biological origin was investigated. A defined set of mixed cultures was used for hydrolysis and the hydrolysates were used to produce H2. The effluent from H2 production stage was used for PHA production. Under batch culture, a maximum of 62 l H2/kg of pure potato peels (Total solid, TS 2 %, w/v) and 54 l H2/kg of mixed biowastes (MBW1) was recorded. Using effluent from the H2 production stage of biowaste mixture (MBW1), Bacillus cereus EGU43 could produce 195 mg PHA/l and 15.6 % (w/w). Further, supplementation of GM-2 medium (0.1×) and glucose (0.5 %) in H2 production stage effluents, resulted in significant improvements of up to 11 and 41.7 % of PHA contents, respectively. An improvement of 3.9- and 17-fold in PHA yields as compared to with and without integrative H2 production from the MBW1 has been recorded. This integrative approach seems to be a suitable process to improve the yields of H2 and PHA by mixing biowastes.
Similar content being viewed by others
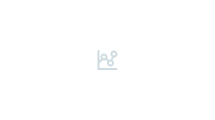
Explore related subjects
Discover the latest articles, news and stories from top researchers in related subjects.Avoid common mistakes on your manuscript.
Introduction
Replacement of non-renewable fossil fuel with biofuels and non-degradable plastics with bioplastics (polyhydroxyalkanoates—PHA) has gained major attention due to serious global environmental impact, recently [1–8]. In addition, our primary dependence on fossil fuel is un-sustainable, due to rapidly depleting reserves. Therefore, vigorous research initiatives were conducted worldwide to replace them by developing alternative renewable energy resources and PHA [1, 5, 7–17]. Hydrogen (H2) has been considered as a future fuel for over 3 decades due to its eco-friendly nature that can be instrumental in the global economy of the twenty first century. Biological H2 and PHA production seems to be a promising approach for developing sustainable processes [1, 2, 10, 18–25]. These bioproducts are produced by the diverse type of microbial strains, including Bacillus and Enterobacter under dark-fermentative and aerobic conditions [14, 26]. Physiological screening of H2 and PHA producers requires significant efforts [24], and comparative genomics contributed a new approach to identify newer producers [27, 28]. Generally, pure sugars have been widely employed for the production of H2 and PHA [1, 5]. Thus, utilization of biowastes originating from agricultural, food processing and other industrial sources could be a potential low cost feed. However, due to their complex nature, biowastes need to be pre-hydrolyzed before using them for producing H2 and PHA [3, 6]. Various pre-treatment methods for the hydrolysis have been proposed [19]. Biological pre-treatment of biowastes seems to be a promising approach [3, 6, 11, 25, 29, 30]. In general, individual microorganism has limited ability to utilize the diverse kind of substrates present in the biowaste, which may lead to low or poor process efficiency. On the other hand, the exploitation of the defined mixed microbial culture could provide significant advantages over monocultures and undefined enriched cultures [1, 6].
Previous studies had suggested that integrating of H2 and PHA production processes hold promise for the future [3, 7, 31–33]. However, the complex nature of mixed biowastes is likely to result in the significant variation during the overall process of H2 and PHA [2, 6, 29]. In this study, an integrative approach was employed to improve H2 and PHA production from pre-hydrolyzed biowastes by defined hydrolytic mixed culture (MHC2).
Materials and Methods
Organisms and Growth Conditions
Mixture of defined bacterial strains, previously designated as MHC2, was used for hydrolysis of biowaste. MHC2 consisted of Bacillus sphaericus EGU542; Bacillus thuringiensis EGU378; Bacillus sp. strains EGU85, EGU367 and EGU447; and Proteus mirabilis EGU30, Defined mixed culture designated as MMC4 was used for H2 production. MMC4 was composed of Enterobacter aerogenes EGU16; Proteus mirabilis EGU21; Bacillus cereus EGU43; Bacillus thuringiensis EGU45; Bacillus pumilus HPC464; and Bacillus sp. HPC459. For PHA production, B. cereus EGU43 was used. These strains have been used in previous studies [3, 15, 29]. Bacterial strains were grown in HiMedia nutrient broth (13 g/l) at 37 °C at 200 rpm for 16–20 h. Actively growing cells were centrifuged at 5600×g for 20 min and their protein content was estimated using Lowry’s method [29]. The mixed cultures of defined bacteria were prepared for inocula using equal proportions to achieve a final protein concentration of 10 µg/ml as described previously [29].
Preparation of Mixed Biowaste and Hydrolysis
Eleven vegetable biowastes were collected from municipal market of Delhi (India). Each biowaste was washed and cut into small pieces (0.25 cm). These pieces were used within 1 h of their collection. Eleven mixed biowaste combinations (MBW1–MBW11) were based on the Plackett Burman design as described earlier [18]. Each combination consisted of 6 different vegetable wastes. For batch-culture digestion, a final concentration of 2 % total solids (TS) was used. Unsterilized slurry (250 ml) was prepared with distilled water in 300 ml bottles. The slurry was inoculated with MHC2 and the mixture was incubated at 37 °C for a period of 2 days as described earlier [29].
Hydrogen Production
For batch-culture digestions, 250 ml of hydrolytic slurries on individual waste basis as well as mixed biowastes were inoculated with MMC4 and the pH of the slurry was adjusted to 7.0 as previously described [29]. Bottles were flushed with argon and incubated at 37 °C. The pH of the solution was checked by opening the bottle and adjusted to 7.0 with 2 N NaOH or 2 N HCl on a daily basis. The evolved gases were collected by water displacement method [18]. Since, certain bacteria, which may accompany the biowaste as contaminants, their contribution towards H2 production was evaluated to be 5–15 ml from 250 ml mixed biowaste (2 %, TS). Further, influence of TS on the H2 production was evaluated using MBW1 containing about 1, 2, 3, 5 and 7 % of TS as a feed.
PHA Production
Samples (200 ml) of biowastes (individual or mixed at 2 %, TS: (1) hydrolysate produced by MHC2, and (2) effluent generated after H2 production were centrifuged and used as a feed for PHA production [29]. The pH of biowaste was adjusted to 7.2 and inoculated with B. cereus EGU43 at the rate of 10 μg cell protein/ml for producing PHA [29]. Further, supplementation of effluents from H2 production stage was done with: (1) medium GM-2(0.1×), and (2) glucose (0.5 %), to evaluate their influence on PHA yields.
Analytical Methods
Gas Analysis
The evolved biogas was analyzed by Gas Chromatographer (Nucon GC5765, India) fitted with a thermal conductivity detector. The carrier gas (Argon) was flown at a rate of 30 ml/min. Gas collection and analyses were done daily and H2 component calculated as described previously [26].
Volatile Fatty Acids Analysis
1.5 ml of liquid samples of effluents after H2 production from individual or mixed biowaste hydrolysates were centrifuged for 20 min at 10,000 rpm, 4 °C and filtered through a filter paper (0.45 μm). Volatile fatty acids (VFA) were analyzed by gas liquid chromatography (Nucon GC5765) using flame ionization detector [29].
PHA Analysis
200 ml aliquots of bacterial culture were used for the analysis of dry cell mass (DCM) and PHA production as described previously [3]. PHA was also analyzed with GC–FID fitted with 10 % Reoplex 400 column. Gravimetric estimation of the produced PHA yield was performed by extraction of the polymer using chloroform and methanol as described previously [34].
TS Analysis
The TS of the vegetables biowaste was estimated by heating a sample at 110 °C for 24 h Table S1 [29].
Results
H2 Production from Biowaste
With Mixed Bacterial Cultures: MHC2 and MMC4
With mixed hydrolytic bacterial culture (MHC2), the observed H2 evolution varied from 20 to 160 ml from 250 ml feed of different biowastes, over 4 days of incubation. H2 constituted 20–51 % of the total biogas produced and the yields were equivalent to 4–32 l H2/kg of TS feed (Table 1). Among these different biowastes, potato peels resulted in maximum H2 yields of 32 l/kg TS fed, whereas turnip resulted in lowest yields of 4 l/kg of TS fed. With a mixture of H2-producing bacteria (MMC4) H2 evolution was observed to be in the range of 25–185 ml from the different biowastes, equivalent to H2 yield of 5–37 l/kg TS fed (Table 1). Here, MMC4 was observed to perform better than hydrolytic cultures (MHC2).
With combination of mixed bacterial cultures: MHC2 and MMC4
A beneficial effect on enhancing H2 yields was observed by treating pea-shell slurry with hydrolytic and H2 producing mixed cultures [29]. All the wastes, when subjected to MHC2 for 2 days and subsequently to MMC4 were found to evolve 30–310 ml of H2/250 ml of feed. It constituted 40–60 % of the total biogas evolved (Table 1). Among these different biowastes, potato peels proved to be the most effective, with a maximum H2 yield of 62 l/kg TS fed. The combinations of MHC2 and MMC4 resulted in up to twofold improvement in H2 yields, in comparison to either of them on an individual basis.
H2 Production from mixed biowastes
Different combinations of biowastes based on Placket-Burmann design proved helpful in improving the H2 production [18]. Here, H2 production from 11 different biowaste combinations designated as MBW1 to MBW11 are presented in Table 2. On the basis of the individual potential of wastes to produce H2, we can expect to generate 90–165 ml of H2 from 250 ml of 2 % TS slurry. It can be observed that H2 production by MMC4 from mixed biowastes were higher than their expected values. These H2 yields varied from 80 to 270 ml. It indicates that in certain combinations mixed wastes provide better feed to bacteria. The H2 yields were observed in the ranges of 16–54 l/kg TS fed. Combination MBW1 proved to be the most effective (54 l/kg TS fed), with 1.6-fold improvement over expected value and H2 content of 54 %.
H2 production results with MMC4 from pre-hydrolysed MBW1 (TS in the range of 1–7 %) are presented in Fig. 1. H2 evolution varied in the ranges of 110–450 ml. Here, H2 constituted 43.8–60.4 % of total biogas produced. It was observed that volumetric H2 production has increased with increase in TS fed from 1 to 7 %. The high H2 yield of 54 l/kg TS was obtained with 2 % TS of MBW1. The H2 yields were negatively influenced by higher TS % feed.
PHA Production
Biowastes
PHA production by B. cereus EGU43 from biowaste hydrolyzed with MHC-2 is presented in Table 3. Growth on the different biowastes were observed in the ranges of 570–880 mg DCM/l. Here, PHA production was in the ranges of 8–17 mg/l with contents in the range of 1.1–2.1 %. Although, PHA production was not observed by B. cereus EGU43 in case of individual biowaste of capsicum, carrot and eggplant hydrolysate. However, this study demonstrated that PHA can be produced from the different biowastes. Potato and tomato wastes turned out to better feeds for PHA production.
Mixed Biowastes
PHA production from the mixed biowaste MBW1–MBW11 hydrolysed with MHC2 by B. cereus EGU 43 is presented in the Table 4. The growth of B. cereus EGU43 was significantly improved than the individual biowastes. The DCM was observed in the ranges of 825–1030 mg/l. Here, PHA production was in the ranges of 14–45 mg/l of DCM with its contents in the ranges of 1–7–4.4 %. Interestingly, the PHA contents improved up to fourfold from the mixed biowaste as compared to the individual wastes.
Effluents of H2 production
PHA production from the effluents of H2 production stages (under non-shaking conditions) of different MBW1–MBW11 by B. cereus EGU43 was quite variable (Table 4). The PHA yields were 28–195 mg/l of DCM with improved biomass in the ranges of 955–1250 mg/l. Here, PHA content varied from 2.8 to 15.6 % of the DCM. The maximum PHA production resulted in the MBW1, which constituted combinations of capsicum, carrot, cauliflower, onion, potato and radish. This high PHA production directly related to the metabolites intermediate produced during the fermentation at H2 production stage. The major metabolites intermediates were acetate, propionate and butyrate during the H2 fermentation stage of both individual and mixed biowaste (Fig. 2). These metabolites acetate, propionate and butyrate were in the ranges of—(1) 540–1480, 120–205 and 365–840 mg/l, and (2) 510–1220, 70–180 and 290–715 mg/l, respectively. Here, high contents PHA in MBW1 can be explained with higher concentrations of these metabolites intermediates. The direct utilization of the biowaste hydrolysate is not suitable for the high PHA yields and suitable complementation of these wastes can lead slightly higher PHA production than the individual biowastes. Therefore, integrative approach of H2 and PHA production in two stage system proved to be more beneficial.
In our previous study, we had observed that supplementation of the medium (GM-2) and glucose is a suitable approach to improve the PHB contents in the B. cereus from hydrolysate [29], or effluents of H2 production from PSS [3]. Here, the supplementation of medium GM-2 (0.1×) and glucose (0.5 %) were evaluated with the MBW1 on effluent from H2 production stage. Supplementation of hydrolysate with GM-2 (0.1×) resulted in the improvement of PHA contents from 15.6 to 19.5 % with slightly higher growth than controls (Fig. 3). Further, supplementation of medium with glucose (0.5 %) resulted in significant improvement in the PHA production. Here, PHA contents increased from 15.6 to 41.7 % of DCM. Overall, PHA yield improved from 45 to 765 mg/l after integration with H2 production stage effluents.
Discussion
Phylogenetically diverse groups of the organisms are well reported for the H2 and PHA production from pure sugars and biowaste as feed [3, 23, 24, 26, 29, 30, 35]. Recently, a few reports have shown the alternative strategy of integrating H2 with PHA production [3, 7, 31–33]. Previously, we had developed an integrative approach of H2 and PHB production from the defined cultures of Bacillus spp.—B. cereus and B. thuringinesis using glucose and biowaste PSS as feed [3, 20, 35]. Nutritional balance of C:N ratio and metabolites intermediates need to be manipulated for the enhancement of PHB production at the second stage. PS slurry has resulted in only production of PHB. Further, to explore the feasibility of different co-polymers production, mixed biowaste combinations were found suitable as alternative initial feed. Here, we have investigated the H2 and PHA production potential of the individual and defined mixed biowastes. Among individual biowaste, potato peels resulted in the maximum H2 yields of 62 l/kg of TS feed by combinations of hydrolytic (MHC2) and H2 producers (MMC4.) This value is significantly higher than the individual mixed cultures with H2 yields of 29 and 37 l/kg of TS feed, respectively. Fermentation of hydrolysed (MHC2) biowaste by B. cereus EGU43 resulted in quite varied in PHA yields. These variations might be primarily due to the balanced C:N components in their hydrolysate. With potato peels, maximum PHA yield of 17 mg/l. Generally, available biowastes get mixed during disposal. To overcome this problem in addition to low yields of H2 and PHA, we have evaluated the potential of defined mixed biowastes for improvement in H2 and PHA production. In comparison with biowastes, defined composition of mixed biowastes are more efficient for the H2 and PHA production. Here, maximum H2 production was observed up to 1.8-fold higher the expected values with their individual combinations in each mixed biowastes (MBW1–MBW11). The H2 production by MMC4 from MBW1 is quite efficient at higher TS feed up to 7 %. On the other hand, PHA production was also improved up to 2.6-fold with mixed biowaste (MBW1). Interestingly, all combinations (MBW1–MBW11) lead to PHA production, whereas individual biowaste capsicum, carrot and eggplant did not result in PHA production by B. cereus EGU43. Further, PHA yields were increased up to 4.9-fold using the H2 production effluents MBWs. Many reports suggested that the high ratio of C:N is required to achieve high PHA production from either pure sugars or biowaste material [20, 29, 34]. After the supplementation of medium GM-2 (0.1×) and glucose (0.5 %), overall PHA yields up to 17-folds in integrative H2 and PHA process were observed than the single stage of PHA production. This PHA content of 41.7 % of DCM is significantly higher than the previous report on oil mill and synthetic medium wastewater with PHA contents of 8.9 and 25 % in integrative H2 and PHA production processes, respectively [31, 32]. These results suggest that more feasibility of utilization of mixed biowastes in suitable combinations for the production of integrative H2 and co-polymers of PHA. This PHA content by B. cereus EGU43 is quite similar with PHA contents of 43.3 % of DCM by B. cereus from Taihu blue algae as feed in the integrative process [33]. Bacillus has abilities to utilize the different waste materials for H2 and PHA production [3, 18, 22–24, 29, 35]. Bacillus also has the unique ability to produce PHA under non-limiting physioloigcal conditions [24]. Although, very few reports are available on H2 production with unsterilized biowastes as feed [3, 29, 36]. Here, the high compatibility for efficient hydrolysis and H2 production by these strains under unsterilized conditions from biowaste suggests that a cost effective integrative approach of H2 and PHA production is feasible.
References
Patel SKS, Kumar P, Kalia VC (2012) Enhancing biological hydrogen production through complementary microbial metabolisms. Int J Hydrogen Energy 37:10590–10603. doi:10.1016/j.ijhydene.2012.04.045
Patel SKS, Kumar P, Singh S, Lee JK, Kalia VC (2015) Integrative approach for hydrogen and polyhydroxybutyrate production. In: Kalia VC (ed) Microbial factories waste treatment. Springer, New Delhi, pp 73–85. doi:10.1007/978-81-322-2598-0_5
Patel SKS, Kumar P, Singh S, Lee JK, Kalia VC (2015) Integrative approach to produce hydrogen and polyhydroxybutyrate from biowaste using defined baterial cultures. Bioresour Technol 176:136–141. doi:10.1016/j.biortech.2014.11.029
Patel SKS, Selvaraj C, Mardina P, Jeong JH, Kalia VC, Kang YC, Lee JK (2016) Enhancement of methanol production from synthetic gas mixture by Methylosinus sporium through covalent immobilization. Appl Energy 171:383–391. doi:10.1016/j.apenergy.2016.03.022
Kumar P, Patel SKS, Lee JK, Kalia VC (2013) Extending the limits of Bacillus for novel biotechnological applications. Biotechnol Adv 31:1543–1561. doi:10.1016/j.biotechadv.2013.08.007
Kumar P, Ray S, Kalia VC (2016) Production of co-polymers of polyhydroxyalkanoates by regulating the hydrolysis of biowastes. Bioresour Technol 200:413–419. doi:10.1016/j.biortech.2015.10.045
Arumugam A, Sandhya M, Ponnusami V (2014) Biohydrogen and polyhydroxyalkanoates co-production by Enterobacter aerogenes and Rhodobacter sphaeroides from Calophyllum inophyllum oil cake. Bioresour Technol 164:170–176. doi:10.1016/j.biortech.2014.04.104
Venkata Mohan S, Nikhil GN, Chiranjeevi P, Reddy CN, Rohit MV, Naresh AK, Sankar O (2016) Waste biorefinery models towards sustainable circular bioeconomy: critical review and future perspectives. Bioresour Technol. doi:10.1016/j.biortech.2016.03.130
Reddy CSK, Ghai R, Rashmi, Kalia VC (2003) Polyhydroxyalkanoates: an overview. Bioresour Technol 87:137–146. doi:10.1016/S0960-8524(02)00212-2
Kalia VC, Purohit HJ (2008) Microbial diversity and genomics in aid of bioenergy. J Ind Microbiol Biotechnol 35:403–419. doi:10.1007/s10295-007-0300-y
Levin DB, Chahine R (2010) Challenges for renewable hydrogen production from biomass. Int J Hydrogen Energy 35:4962–4969. doi:10.1016/j.ijhydene.2009.08.067
Singh M, Patel SKS, Kalia VC (2009) Bacillus subtilis as potential producer for polyhydroxyalkanoates. Microb Cell Fact 8:38. doi:10.1186/1475-2859-8-38
Singh M, Kumar P, Ray S, Kalia VC (2015) Challenges and opportunities for the customizing polyhydroxyalkanoates. Indian J Microbiol 55:235–249. doi:10.1007/s12088-015-0528-6
Patel SKS, Kumar P, Mehariya S, Purohit HJ, Lee JK, Kalia VC (2014) Enhancement in hydrogen production by co-cultures of Bacillus and Enterobacter. Int J Hydrogen Energy 39:14663–14668. doi:10.1016/j.ijhydene.2014.07.084
Kumar P, Mehariya S, Ray S, Mishra A, Kalia VC (2015) Biodiesel industry waste: a potential source of bioenergy and biopolymers. Indian J Microbiol 55:1–7. doi:10.1007/s12088-014-0509-1
Kumar P, Mehariya S, Ray S, Mishra A, Kalia VC (2015) Biotechnology in aid of biodiesel industry effluent (glycerol): biofuels and bioplastics. In: Kalia VC (ed) Microbial factories. Springer, New Delhi, pp 105–119. doi:10.1007/978-81-322-2598-0
Kalia VC, Prakash J, Koul S (2016) Biorefinery for glycerol rich biodiesel industry waste. Indian J Microbiol 56:113–125. doi:10.1007/s12088-016-0583-7
Patel SKS, Purohit HJ, Kalia VC (2010) Dark fermentative hydrogen production by defined mixed microbial cultures immobilized on ligno-cellulosic waste materials. Int J Hydrogen Energy 35:10674–10681. doi:10.1016/j.ijhydene.2010.03.025
Kim D-H, Kim M-S (2013) Development of a novel three-stage fermentation system converting food waste to hydrogen and methane. Bioresour Technol 127:267–274. doi:10.1016/j.biortech.2012.09.088
Singh M, Kumar P, Patel SKS, Kalia VC (2013) Production of polyhydroxyalkanoate co-polymer by Bacillus thuringiensis. Indian J Microbiol 53:77–83. doi:10.1007/s12088-012-0294-7
Kumar P, Pant DC, Mehariya S, Sharma R, Kansal A, Kalia VC (2014) Ecobiotechnological strategy to enhance efficiency of bioconversion of wastes into hydrogen and methane. Indian J Microbiol 54:262–267. doi:10.1007/s12088-014-0467-7
Kumar P, Singh M, Mehariya S, Patel SKS, Lee JK, Kalia VC (2014) Ecobiotechnological approach for exploiting the abilities of Bacillus to produce co-polymer of polyhydroxyalkanoate. Indian J Microbiol 54:1–7. doi:10.1007/s12088-014-0457-9
Kumar P, Sharma R, Ray S, Mehariya S, Patel SKS, Lee JK, Kalia VC (2015) Dark fermentative bioconversion of glycerol to hydrogen by Bacillus thuringiensis. Bioresour Technol 182:383–388. doi:10.1016/j.biortech.2015.01.138
Kumar P, Ray S, Patel SKS, Lee JK, Kalia VC (2015) Bioconversion of crude glycerol to polyhydroxyalkanoate by Bacillus thuringiensis under non-limiting nitrogen conditions. Int J Biol Macromol 78:9–16. doi:10.1016/j.ijbiomac.2015.03.046
Nissila ME, Lay C-H, Puhakka JA (2014) Dark fermentative hydrogen production from lignocellulosic hydrolysates—a review. Biomass Bioenergy 67:145–159. doi:10.1016/j.biombioe.2014.04.035
Porwal S, Kumar T, Lal S, Rani A, Kumar S, Cheema S, Purohit HJ, Sharma R, Patel SKS, Kalia VC (2008) Hydrogen and polyhydroxybutyrate producing abilities of microbes from diverse habitats by dark fermentative process. Bioresour Technol 99:5444–5451. doi:10.1016/j.biortech.2007.11.011
Kalia VC, Chauhan A, Bhattacharyya G, Rashmi (2003) Genomic databases yield novel bioplastic producers. Nat Biotechnol 21:845–846. doi:10.1038/nbt0803-845
Kalia VC, Lal S, Ghai R, Mandal M, Chauhan A (2003) Mining genomic databases to identify novel hydrogen producers. Trends Biotechnol 21:152–156. doi:10.1016/S0167-7799(03)00028-3
Patel SKS, Singh M, Kumar P, Purohit HJ, Kalia VC (2012) Exploitation of defined bacterial cultures for production of hydrogen and polyhydroxybutyrate from pea-shells. Biomass Bioenergy 36:218–225. doi:10.1016/j.biombioe.2011.10.027
Patel SKS, Kalia VC (2013) Integrative biological hydrogen production: an overview. Indian J Microbiol 53:3–10. doi:10.1007/s12088-012-0287-6
Ntaikou I, Kourmentza C, Koutrouli EC, Stamatelatou K, Zampraka A, Kornaros M, Lyberatos G (2009) Exploitation of olive oil mill wastewater for combined hydrogen and biopolymer production. Bioresour Technol 100:3724–3730. doi:10.1016/j.biortech.2008.12.001
Venkata Mohan S, Reddy MV, Subhash GV, Sarma PN (2010) Fermentative effluents from hydrogen producing bioreactor as substrate for poly(β-OH) butyrate production with simultaneous treatment: an integrated approach. Bioresour Technol 101:9382–9386. doi:10.1016/j.biortech.2010.06.109
Yan Q, Zhao M, Miao H, Ruan W, Song R (2010) Coupling of the hydrogen and polyhydroxyalkanoates (PHA) production through anaerobic digestion from Taihu blue algae. Bioresour Technol 101:4508–4512. doi:10.1016/j.biortech.2010.01.07
Kumar T, Singh M, Purohit HJ, Kalia VC (2009) Potential of Bacillus sp. to produce polyhydroxybutyrate from biowaste. J Appl Microbiol 106:2017–2023. doi:10.1111/j.1365-2672.2009.04160.x
Patel SKS, Singh M, Kalia VC (2011) Hydrogen and polyhydroxybutyrate producing abilities of Bacillus spp. from glucose in two stage system. Indian J Microbiol 51:418–423. doi:10.1007/s12088-011-0236-9
Castello E, Santos CG, Iglesias T, Paolino G, Wenzel J, Borzacconi L, Etchebehere C (2009) Feasibility of biohydrogen production from cheese whey using a UASB reactor: links between microbial community and reactor performance. Int J Hydrogen Energy 34:5674–5682. doi:10.1016/j.ijhydene.2009.05.060
Acknowledgments
The authors wish to thank the Director of CSIR-Institute of Genomics and Integrative Biology, Delhi, India, CSIR-WUM (ESC0108), Government of India for providing the necessary funds, facilities and moral support. This research was also supported by the 2015 KU Brain Pool of Konkuk University.
Author information
Authors and Affiliations
Corresponding author
Ethics declarations
Conflict of interest
The authors declare no conflict of interest.
Electronic supplementary material
Below is the link to the electronic supplementary material.
Rights and permissions
About this article
Cite this article
Patel, S.K.S., Lee, JK. & Kalia, V.C. Integrative Approach for Producing Hydrogen and Polyhydroxyalkanoate from Mixed Wastes of Biological Origin. Indian J Microbiol 56, 293–300 (2016). https://doi.org/10.1007/s12088-016-0595-3
Received:
Accepted:
Published:
Issue Date:
DOI: https://doi.org/10.1007/s12088-016-0595-3