Abstract
The complex magnetic materials La0.70Ca0.1Sr0.2Fe0.1Mn0.9O3 (LCSFMO) and La0.70Ca0.1Sr0.2MnO3 (LCSMO), applicable for the magnetic cooling, have been explored. The X-ray diffraction patterns show that these samples are in single phase with rhombohedral structure. A remarkable decrease in the Curie temperature from 346 to 223 K was observed for 10% of Fe doping at Mn-site in the LCSMO sample. The substitution of Mn by Fe results in a combination of doping disorder, a site-percolation and Mn–Fe super-exchange interactions, which suppresses the ferromagnetism. The maximum magnetic entropy change of 1.53 J kg−1 K−1 with field variation of 3 T was obtained for the LCSFMO sample. The relative cooling power (RCP) ∼120 J kg−1 was found for an applied magnetic field of 3 T. The comparable magnetic entropy change and RCP values suggested that the studied samples have potential as a refrigerant for cooling applications.
Similar content being viewed by others
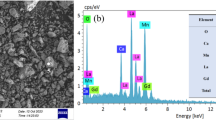
Avoid common mistakes on your manuscript.
1 Introduction
In recent times, the magnetic refrigeration at room temperature has been received increasing attention against the well-established gas refrigeration technology.[1–3] The magnetic materials that can display a large magnetocaloric effect (MCE) were reported as candidate materials for magnetic refrigeration.[4–7] Rare-earth manganites of the A 1−x B x MnO3 type (where A = La, Pr, Nd, etc. and B = Ca, Sr, Ba, etc.) have been explored in the last decade, owing to their good magnetocaloric response at various temperatures.[8,9] The MCE is the property of some materials (like paramagnetic salts or magnetic stuffs) to get heated in the presence of magnetic field and cool down when the field is removed from the materials.[4] The compounds which show the sharp temperature-dependent magnetic-phase transition can have large MCE.[10] The magnetic cooling has several benefits against the gas refrigeration, such as low vibration, high efficiency, durability, and is environmental friendly. Therefore, in last decade more effort was done to develop the refrigerants with large MCE based on magnetic substances.
The physical properties of perovskite-type manganites can be changed by doping at A-site or B-site (Mn-site) with various elements having different ionic radii.[11,12] In manganites, doping with different elements alters the Mn3+ –O–Mn4+ network, which changes the strength of double exchange (DE) as well as superexchange interactions.[13] As compared to A-site doping, Mn-site doping will not only modify the Mn3+–O–Mn4+ network but also brings about many complicated new magnetic exchange interactions between the Mn ions and doped transition metal ions.[14–16] The lattice effect, the concentration of Mn4+ ions, oxygen vacancies and the interaction of ferromagnetic DE or anti-ferromagnetic superexchange between the dopant and the Mn ions should be taken into account for Mn-site doping.[17,18] Due to this reason the Mn-site doping was performed in this work. It was found that dopant size and valence-induced local strain dominate the magneto-transport properties in manganites and change the long-range FM ordering; consequently change in the magnetic properties of the pervoskite manganites. In order to get the enhancement in the magnetocaloric response of the La0.70Ca0.1Sr0.2MnO3 (LCSMO) manganite, Fe was doped at Mn-site. The second goal of this research is to develop a potential refrigerant for magnetic cooling.
2 Experimental
The polycrystalline LCSMO and La0.70Ca0.1Sr0.2Fe0.1Mn0.9O3 (LCSFMO) samples were synthesized by using the solid-state reaction technique.[19] The metal oxide: La2O3, SrO, CaO, Fe2O3 and Mn2O3 (99 .99% pure, Sigma-Aldrich) powders were mixed in stoichiometric amount using ethanol and were ball milled for 6 h. The mixed slurry was dried for 10 h at 80∘C and then first heated at 800∘C in air for calcination for 10 h. The dried powder was grinded and then pressed into a pellet shape. The pellets were first annealed at 1100∘C for 30 h followed by repeated grinding. Finally, the sintering of pellets was done at 1250∘C for 24 h. The as-obtained pellets were used for characterization.
The polycrystalline behaviour and phase purity of the samples were studied by using a X-ray diffractometer (Bruker-D8-Advance) operating with CuK α1 (λ=1.5406 Å, 40 kV, 40 mA) radiation at room temperature. Scanning electron microscope (SEM; SEM-JSM5610) was used to study the surface morphology of the samples. Average grain size was determined by using the Image-J software from SEM micrographs. The magnetic measurements were recorded by using a vibrating sample magnetometer (PPMS-6000 VSM, Quantum design) with a temperature range of 4–400 K.
3 Results and discussion
The structural study of LCSFMO sample in terms of X-ray diffraction (XRD) pattern is shown in figure 1. The polycrystalline behaviour of the LCSFMO sample was observed from the XRD pattern with the multiple reflections. The rhombohedral structure (R−3c space group) with the lattice constants of a=b=5.4981±0.005 Å and c=13.2433±0.005 Å was obtained for this sample using POWDER-X software. Also, the highest intensity was observed for the (104) plane as preferred orientation with rhombohedral structure. In order to compare, the XRD pattern of LCSMO is also plotted.
Figure 2 shows the polycrystalline structure in typical SEM micrographs of the LCSFMO and LCSMO samples. It was observed that the both samples have mainly strongly connected grains. The LCSFMO sample has smaller grain size. The average grain size was found to ∼9 and 6 μm for LCSMO and LCSFMO samples, respectively.
Figure 3a shows the magnetization of samples as a function of temperature. The magnetization measurement was performed in the temperature range of 100–375 K during the warming up cycle. The Curie temperature (T C) was evaluated by determination of minimum of the derivative d M/d T of the M–T curve (not shown here). The obtained values of T C are 232 and 350 K for LCSFMO and LCSMO samples, respectively. The lowering of T C is because of the change in DE interaction in the LCSFMO sample created by Fe doping. On the other hand, ferromagnetism in Fe-doped sample is expected to enhance because of the Fe3+–O–Mn3+ super-exchange interaction.[20] However, we observed negative contribution due to the weakening of DE interaction caused by Fe doping. From thermodynamics point of view, the presence of magnetic hysteresis in the working materials (magnetic refrigerants) has adverse effect on cooling efficiency. In that context, it is impressive to have the sample like LCSFMO because of no magnetic hysteresis (inset of figure 3b), which is benign for the cooling efficiency.
The magnetocaloric effect was evaluated by calculating the magnetic entropy change (ΔS M) upon an application of magnetic field using the thermodynamic Maxwell’s equation, given as follows:[21]
The numerical approximation of equation (1) for magnetization measured at distinct field and temperature intervals is given as
where M i and M i+1 are the magnitude of magnetization at the temperature T i and T i+1, respectively, under a magnetic field H i . In our case, the minimum field was set to 0. Inset of figure 4 shows the evolution of magnetization with magnetic field (up to 3 T) at different temperatures on both sides of the T C for LCSFMO sample. For accurate determination of maximum in magnetic entropy change \(\left (\left | {\Delta } S_{\mathrm {M}}^{\max } \right |\right )\), the different temperature interval was selected for different region (5 K near T C and 10–20 K in other temperature regions). The increase in magnetization was observed with the decrease in temperature from 260 to 80 K, where more spins get aligned in the direction of field with decreasing temperature. The type of the magnetic phase transition in the LCSFMO sample has been investigated on the basis of the Banerjee criterion.[22] This criterion tells that at temperature higher than T C, M 2 vs. H/M curves (Arrott plot) have positive and negative slopes for second- and first-order phase transition, respectively. A quintessential set of Arrott plots for the LCSFMO sample is depicted in figure 4. Clearly, the as-obtained Arrott plots show positive slope for all temperatures, i.e., the second order of phase transition.
The magnetic entropy change (ΔS M) was determined numerically by using equation (2), for LCSFMO sample at different applied magnetic fields. Figure 5 shows that the LCSFMO sample has well enough \(\left |{\Delta } S{_{\mathrm {M}}^{\max } } \right |\) as compared with other manganites.[10,23] Also, inset of figure 5 reveals that, the \(\left | {\Delta S_{\mathrm {M}}^{\max } } \right |\) was found to increase deliberately with the magnetic field. The numerical values of \(\left | {\Delta S_{\mathrm {M}}^{\max } } \right |\) at field change (ΔH) of 1 and 3 T were found ∼0 .71 and 1 .53 J kg−1 K−1, respectively. On the basis of this result, we conclude that the enhancement in \(\left | {\Delta S_{\mathrm {M}}^{\max } } \right |\) is expected at higher applied magnetic fields.
The parameter, relative cooling power (RCP) plays a significant role in selection of good quality of refrigerants. It can be expressed as follows:[21]
where ∂ T FWHM is the full-width at half-maximum and \(\left | {\Delta S_{\mathrm {M}}^{\max } } \right |\) is the respective magnetic entropy change. The better magnetocaloric materials usually have large RCP values and consequently, have high cooling efficiency. Inset of figure 5 provides the information about RCP of the LCSFMO sample, also gives the behaviour of RCP against the applied magnetic field. The RCP value of 120 J kg−1 was observed for the field change (ΔH) of 3 T. One can notice that the higher magnetic field gives the larger RCP values for the same sample.
On the other hand, it is well understood that the magnetic refrigerants have good efficiency around the Curie temperature. In this regard, the magnetic refrigerants composed of materials having similar chemical and physical properties can facilitate wide range of working temperature as well as enhanced cooling efficiency. As the LCSFMO sample has the broad transition temperature and comparable RCP value, it can be used as potential magnetic refrigerants with a wide range of operating temperature.
4 Conclusions
We have made a precise analysis on magnetocaloric properties of complex LCSFMO compound. The polycrystalline LCSFMO manganite crystallized in the rhombohedral structure with preferred orientation of (104) plane. This compound exhibits considerably no magnetic hysteresis at 200 K, which is favourable for the magnetic cooling efficiency. A second order of transition from ferromagnetic to paramagnetic phase was observed. The improvement in the value of \(\left | {\Delta S_{\mathrm {M}}^{\max } } \right |\) was found at higher magnetic field for the LCSFMO sample. The RCP value of 120 J kg−1 was observed for the ΔH of 3 T and increases with the applied magnetic field.
References
Kumar P and Mahendiran R 2012, Appl. Phys. Lett. 101 042411
Gschneidner Jr K A, Pecharsky V K, and Tsokol A O 2005, Rep. Prog. Phys. 68 1479
Anwar M S, Kumar S, Ahmed F, Arshi N, and Koo B H 2012a, Mater. Res. Bull. 47 2977
Othmani S, Blel R, Bejar M, Sajieddine M, Dhahri E, and Hlil E K 2009, Solid State Commun. 149 969
Gschneidner Jr K A and Pecharsky V K 1999, J. Appl. Phys. 85 5365
Pecharsky V K and Gschneidner J. K A 1997, Appl. Phys. Lett. 70 3299
Sarkar P, Mandal P, and Choudhury P 2008, Appl. Phys. Lett. 92 182506
Koubaa W C, Koubaa M, and Cheikhrouhou A 2011, J. Alloys Compd. 509 4363
Anwar M S, Kumar S, Ahmed F, Arshi N, Kim G W, Lee C G, and Koo B H 2011, J. Magn. 16 457
Anwar M S, Kumar S, Ahmed F, Heo S N, Kim G W, and Koo B H 2013, J. Electroceram. 30 46
Yang J, Song W H, Ma Y Q, Zhang R L, Zhao B C, Sheng Z G, Zheng G H, Dai J M, and Sun Y P 2004, Phys. Rev. B 70 092504
Anwar M S, Kumar S, Ahmed F, Arshi N, and Koo B H 2012b, J. Nanosci. Nanotechnol. 12 5523
Hwang H Y, Cheong S W, Radaelli P G, Marenzio M, and Batlogg B 1995, Phys. Rev. Lett. 75 914
Mnefgui S, Zaidi N, Dhahri A, Hlil E K, and Dhahr J 2014, J. Solid State Chem. 215 193
Phan T L, Zhang P, Thanh T D, and Yu S C 2014, J. Appl. Phys. 115 17A912
Reshmi C P, Pillai S S, Suresh K G, and Verma M R 2013, Solid State Sci. 19 130
Troyanchuk I O, Bushinsky M V, Szymczak H, Baran M, and Barner K 2007, J. Magn. Magn. Mater. 312 470
De K, Majumdar S, and Giri S 2007, J. Phys. 40 5810
Anwar M S, Kumar S, Ahmed F, Hoon S C, Heo S N, and Koo B H 2012c, J. Ceram. Process. Res. 13 S100
Kanamori J 1959, J. Phys. Chem. Solids 10 87
Thiyagarajan R, Muthu S E, Mahendiran R, and Arumugam S 2014, J. Appl. Phys. 115 043905
Banerjee S K 1964, Phys. Lett. 12 16
Ehsani M H, Kameli P, Ghazi M E, Razavi F S, and Taheri M 2013, J. Appl. Phys. 114 223907
Acknowledgements
This research was supported by the Basic Science Research Program through the National Research Foundation of Korea (NRF) funded by the Ministry of Education, Science and Technology (2012-R1A1B3000784), and National Research Foundation of Korea (NRF) grant funded by the Korea government (MEST) (No. 2012-0009452).
Author information
Authors and Affiliations
Corresponding author
Rights and permissions
About this article
Cite this article
ANWAR, M.S., AHMED, F. & KOO, B.H. Magnetocaloric response of La0.70Ca0.1Sr0.2Fe0.1Mn0.9O3 pervoskite for magnetic refrigeration. Bull Mater Sci 38, 101–104 (2015). https://doi.org/10.1007/s12034-014-0816-5
Received:
Revised:
Published:
Issue Date:
DOI: https://doi.org/10.1007/s12034-014-0816-5