Abstract
Selenite cataracts are effective and convenient animal models for simulation of human senile nuclear cataracts. These models are widely used to study the effects of various stresses on eye lenses and to screen anticataract drugs. However, there have been no comprehensive toxicological evaluations of these animal models. To investigate the effects of sodium selenite on some important organs in selenite cataract model animals, this study analyzed (1) histopathology by hematoxylin and eosin (H&E) staining; (2) methionine sulfoxide reductase (Msr) A and B1 protein expression; (3) glutathione peroxidase (GPx), thioredoxin reductase (TrxR) and superoxide dismutase (SOD) activity; and (4) malondialdehyde (MDA) levels in the liver, kidney, and brain in a selenite cataract rat model. The results showed that sodium selenite induced severe oxidative damage, especially in the hippocampus and corpus striatum of the brain, in Sprague–Dawley (SD) rats. This damage was evidenced by mild gliocyte proliferation, significant disorder of neuronal arrangement with acidophilic changes in the hippocampus, and significant occurrence of focal microglia or lymphocytic infiltration in the corpus striatum after selenite injection for cataract simulation. The damage was closely related to significant decreases in antioxidant enzyme expression and activity and significant increases in lipid peroxidation (MDA) levels. Furthermore, nonsignificant swelling and scattered spotty necrosis were observed in the liver. These results imply that physiological changes in model animals should be considered when carrying out anticataract drug screening and that pathological changes in other nontarget organs should be prevented.
Similar content being viewed by others
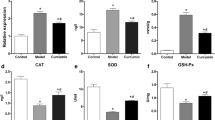
Avoid common mistakes on your manuscript.
Introduction
Animal models are important tools for studying the onset and development of human disease and are helpful for drug screening [1,2,3]. During drug screening, the effective dose that reaches any affected tissue or organ can be determined through analysis of the pharmacokinetics of a candidate drug in test animals. Drug biological function is inevitably influenced by the physiological condition of a model animal, especially in the case of orally administered drugs.
Cataracts are a leading cause of blindness, accounting for approximately 51% of all cases (approximately 20 million people became blind because of cataracts in 2010) [4]. Many animal models of cataracts have been developed for drug screening [5]. Among the several animal models of drug-induced cataracts, selenite cataracts are extremely effective and convenient for simulation of senile nuclear cataracts. These cataract models have played leading roles in research on the effects of various stresses on eye lenses and the mechanisms of cataract formation and in studies screening potential anticataract drugs [6, 7]. However, the physiological conditions of test animals should be considered during anticataract drug screening.
Selenium can cause negative effects such as nervous system disturbances, and selenite has been reported to cause irregular neuronal growth in fish embryos [8]. Overdosing of normotensive Wistar Kyoto rats with Se in the form of sodium selenite (9 mg/kg) significantly increases Se content in the liver and kidney. Consequently, GPx activity slightly increases in the liver but does not change in the kidney; meanwhile, TrxR activity increases significantly in the liver but decreases significantly in the kidney [9].
However, the effects of sodium selenite on other important organs, such as the liver, kidney, and brain, have not been well reported in the literature. Liver status directly affects drug metabolism, and liver enzymes chemically change drug components into metabolites. Furthermore, the kidneys play important roles in drug, hormone, and xenobiotic excretion [10], and the brain plays an important role in metabolic regulation. Thus, the current study investigated the effects of sodium selenite on the liver, kidney, and brain in a selenite cataract animal model.
High levels of selenium induce the generation of reactive oxygen species (ROS), which easily leads to oxidative damage. Oxidative damage in the eye lens is a major cause of cataract formation [11]. Sodium selenite was used in this study to establish a selenite cataract rat model. Our previous study investigated the effects of sodium selenite on the mRNA expression levels of glutathione peroxidase (GPx) 1, methionine sulfoxide reductase (Msr) A, and MsrB1 in the lenses of rats with selenite cataracts [12]. Therefore, oxidative damage and several antioxidant enzymes were assayed in this study. Specifically, MsrA and MsrB1 protein expression levels; GPx, thioredoxin reductase (TrxR), and superoxide dismutase (SOD) activity; and malondialdehyde (MDA) levels were analyzed. Furthermore, histopathological examination was carried out to diagnose pathological changes in the livers, kidneys, and brains of selenite cataract model rats.
Materials and Methods
Materials
MsrA (LF-PA0031) and MsrB1 (LF-PA0088) primary antibodies were obtained from Thermo Fisher (Waltham, MA, USA). A β-actin primary antibody was obtained from Servicebio (Wuhan, Hubei, China). GPx activity, TrxR activity, SOD activity, and MDA concentration measurement kits were purchased from Nanjing Jiancheng (Nanjing, Jiangsu, China). All other chemicals were of the highest commercial grade available.
Animals and Treatment
Male and female Sprague–Dawley (SD) rats (250–300 g) were purchased from Hubei Research Centre for Laboratory Animals (Wuhan, Hubei, China), housed in an air-conditioned animal room with a 12-h day/night schedule at a temperature of 23 ± 1 °C, fed rat chow diet, and given access to tap water ad libitum. The chow diet was purchased from Hubei Research Centre for Laboratory Animals and contained 0.112 μg selenium/g diet and 65 IU vitamin E/kg diet. All animal experiments complied with the Animal Research: Reporting of In Vivo Experiments (ARRIVE) guidelines and were carried out in accordance with the National Institutes of Health Guide for the Care and Use of Laboratory Animals (NIH Publication No. 8023, revised 1978).
One female and one robust male rat were housed in each cage. Approximately 21 days later, the rat pups were born. The day of birth was designated as postnatal day 0. The rat pups were housed with their mother. As the rats grew, they were divided into two groups: the selenite cataract model (SeCa) group and the control (C) group. In the SeCa group, the rat pups were subcutaneously injected in the nape of the neck with sodium selenite on postnatal days 8, 10, 11, and 12 (21 nmol/g body weight [BW]). In the C group, the rat pups were injected in parallel with equivalent volumes of normal saline.
On postnatal day 21 (the fifth day after eyelid opening), the rats were sacrificed. Their livers, kidneys, and brains were quickly excised, rapidly rinsed in ice-cold phosphate-buffered saline (pH 7.4), and dried with absorbent paper. The organs were then used for subsequent analyses or were stored frozen at − 80 °C until analysis. The rats were treated according to the Association for Research in Vision and Ophthalmology Statement for the Use of Animals in Ophthalmic and Vision Research.
Western Blot Analysis
The protein expression levels of MsrA and MsrB1 in the liver, kidney, and brain were assessed for the two groups. There were six rats in each group, and the three tissues from each rat were processed to form three independent protein samples. The proteins were separated by sodium dodecyl sulfate–polyacrylamide gel electrophoresis (SDS–PAGE) and transferred onto polyvinylidene difluoride (PVDF) membranes (300 mA and 25 min for MsrA and MsrB1, 300 mA and 30 min for β-actin). After transfer, the PVDF membranes were incubated for 30 min at room temperature in 5% (w/v) nonfat milk in Tris-buffered saline containing Tween-20 (TBST; 20 mM Tris-HCl and 500 mM NaCl, pH 7.5, supplemented with 0.05% [v/v] Tween-20) to block nonspecific binding. The membranes were subsequently probed overnight at 4 °C with 5% nonfat milk in TBST containing primary antibodies for MsrA, MsrB1, and β-actin at dilutions of 1:2000, 1:500, and 1:2500, respectively. After three washes (5 min each time) with TBST, the membranes were incubated for 30 min at room temperature with horseradish peroxidase-conjugated secondary antibodies diluted 1:3000 in TBST. The membranes were again washed three times (5 min each time) with TBST. To identify specific proteins, an enhanced chemiluminescence (ECL) system was used (Servicebio). β-Actin was used as the protein loading control for MsrA and MsrB1. Quantitative analysis of the blot signals was performed using a CLiNX-6300 imaging system (Clinx Science Instruments Co., Ltd., Shanghai, China) and AlphaEase FC software (open source).
Measurement of GPx Activity
GPx activity was measured according to the method of Hafeman et al. [13] with a commercially available kit (Jiancheng, Nanjing, China). The sample homogenates were centrifuged at 3500 rpm and 4 °C for 10 min, and then, the supernatants were collected for biochemical assays, each of which had two sequential steps: an enzymatic reaction and a chromogenic reaction. The absorbance was measured at 412 nm by an Epoch Microplate Spectrophotometer (BioTek Instruments, Inc., Winooski, VT, USA). One unit of GPx activity was defined as the amount required to decrease the concentration of glutathione by 1 μmol/L per minute per milligram of protein after the nonenzymatic reaction was subtracted, and the results are expressed in units per milligram of protein. The protein content was determined using the Bradford method with bovine serum albumin as the standard.
Measurement of TrxR Activity
TrxR activity was measured according to the DTNB method [14] with a commercially available kit (Jiancheng). The sample homogenates were centrifuged at 8000g at 4 °C for 10 min, and then, the supernatants were collected for biochemical assays. The absorbance was measured at 412 nm by an Epoch Microplate Spectrophotometer (BioTek Instruments, Inc.). One unit of TrxR activity was defined as the amount required to reduce 1 nmol of DTNB per minute per milligram of protein at 37 °C. The protein content was determined using the Bradford method with bovine serum albumin as the standard.
Measurement of SOD Activity
SOD activity was measured according to the hydroxylamine method [15] with a commercially available kit (Jiancheng). The sample homogenates were centrifuged at 3500 rpm and 4 °C for 10 min, and then, the supernatants were collected for biochemical assays. The absorbance was measured at 550 nm by an Epoch Microplate Spectrophotometer (BioTek Instruments, Inc.). One unit of SOD activity was defined as the amount of SOD required for 50% inhibition of the xanthine and xanthine oxidase system reaction in 1 mL of reaction solution per milligram of protein. The protein content was determined using the Bradford method with bovine serum albumin as the standard.
Lipid Peroxidation Detection
Lipid peroxidation was determined indirectly by measuring MDA formation using an MDA measurement kit (Jiancheng) according to the manufacturer’s instructions [16] and is expressed as the MDA level in nanomoles per milligram of protein. The sample homogenates were centrifuged at 3500 rpm and 4 °C for 10 min, and then, the supernatants were collected for biochemical assays. The absorbance was measured at 532 nm by an Epoch Microplate Spectrophotometer (BioTek Instruments, Inc.). The protein content was determined using the Bradford method with bovine serum albumin as the standard.
Histopathology Examination
In brief, tissues were removed and fixed in 10% neutral formalin fixative for 48 h. Then, the tissue samples were washed, dehydrated, cleared, dipped in wax, embedded, sliced, coated, dried, dewaxed, washed, and stained with hematoxylin and eosin (H&E). Before histopathological examination, whole brains were divided into three parts: the hippocampus, corpus striatum, and cerebellum.
Statistical Analysis
All data are expressed as the mean ± standard deviation. All the data were analyzed by Origin computer software (OriginLab, Inc., Northampton, MA, USA). The statistical significance of differences between groups was evaluated by analysis of variance (ANOVA) followed by Student’s t test for pairwise comparisons, and a P value less than 0.05 was considered to indicate statistical significance.
Results
Histopathological Examination of Selenite Cataract Model Rats
H&E staining was used for histopathological examination of liver, kidney, hippocampus, corpus striatum, and cerebellum tissues, and representative images of the results are shown in Fig. 1. In the C group, no abnormalities were observed in the liver, kidney, hippocampus, corpus striatum, or cerebellum. The cells were clearly outlined with compact structures, and the nucleoli were clearly visible. In the SeCa group, no abnormalities were observed in the kidney or cerebellum, and the cells in these tissues were clearly outlined with compact structures. Although the nucleoli were clearly visible, there were slight pathological changes in the liver and obvious pathological changes in the hippocampus and corpus striatum of the brain. In the liver, slight swelling and scattered spotty necrosis were observed, while the hippocampus exhibited mild gliocyte proliferation and significant disordered neuronal arrangement with acidophilic changes. The corpus striatum exhibited significant occurrence of focal microglia or lymphocytic infiltration. Images from other rats in the same group that show the same histopathological changes are shown in Fig. S1 (Supplement).
Histopathological evaluation of the liver, kidney, hippocampus, corpus striatum, and cerebellum tissues of SD rats after selenite cataract formation by H&E staining. SeCa images at postnatal day 21 (the fifth day after eyelid opening) of rats that were injected with sodium selenite on postnatal days 8, 10, 11, and 12 (21 nmol/g BW). C images at postnatal day 21 (the fifth day after eyelid opening) of rats that were injected with equivalent volumes of normal saline on postnatal days 8, 10, 11, and 12. In the SeCa group, slight swelling and scattered spotty necrosis (marked with arrows) were observed in the liver. The representative image of spotty necrosis has been enlarged at the top right corner (marked with a white square frame). Mild gliocyte proliferation and significant disorder of neuronal arrangement with acidophilic changes (marked with square frames) were observed in the hippocampus, and significant occurrence of focal microglia or lymphocytic infiltration (marked with asterisks) was observed in the corpus striatum. CA3: cornu ammonis 3, DG: dentate gyrus. Scale bar: 100 μm. Magnification: 100×
Effect of Selenite Cataract Formation on GPx Activity
GPx, a major selenium-containing antioxidant enzyme, belongs to the family of selenoproteins. GPx reduces lipid hydroperoxides to their corresponding alcohols and free H2O2 to water and plays an important role in protecting the cells from oxidative damage. To analyze differences in antioxidant enzymes in the rat livers, kidneys, and brains, GPx activity was measured. As shown in Fig. 2, GPx activity was increased by 50% (p < 0.01) in the liver, decreased by 16% (p < 0.01) in the brain, and unchanged in the kidney in the SeCa group compared with the C group.
Changes in GPx activity in the livers, kidneys, and brains of SD rats after selenite cataract formation. The data are expressed as the mean ± standard deviation (n = 6 for each group). SeCa liver, kidney, and brain tissues at postnatal day 21 (the fifth day after eyelid opening) from rats injected with sodium selenite on postnatal days 8, 10, 11, and 12 (21 nmol/g BW). C liver, kidney, and brain tissues at postnatal day 21 (the fifth day after eyelid opening) from rats injected with equivalent volumes of normal saline on postnatal days 8, 10, 11, and 12. **p < 0.01, compared to the C group
Effect of Selenite Cataract Formation on TrxR Activity
TrxR is a cellular antioxidant containing selenocysteine in its C-terminal redox center that contributes to total antioxidant and detoxification capacity [17]. As shown in Fig. 3, compared to that in group C, the TrxR activity in group SeCa was increased by 17% (p < 0.01) and 27% (p < 0.001) in the liver and kidney, respectively. However, brain TrxR activity did not differ between the groups.
Changes in TrxR activity in the livers, kidneys, and brains of SD rats after selenite cataract formation. The data are expressed as the mean ± standard deviation (n = 6 for each group). SeCa liver, kidney, and brain tissues at postnatal day 21 (the fifth day after eyelid opening) from rats injected with sodium selenite on postnatal days 8, 10, 11, and 12 (21 nmol/g BW). C liver, kidney, and brain tissues at postnatal day 21 (the fifth day after eyelid opening) from rats injected with equivalent volumes of normal saline on postnatal days 8, 10, 11, and 12. **p < 0.01 and ***p < 0.001, compared to the C group
Effect of Selenite Cataract Formation on SOD Activity
SOD, the first known enzyme in the detoxification process, catalyzes the dismutation of superoxide radicals to H2O2 and molecular oxygen [18]; excessive amounts of these products can start a lethal chain reaction in which the metabolites oxidize and disable structures required for cellular integrity and survival [19]. As shown in Fig. 4, compared to that in the C group, the activity of SOD in the SeCa group was increased by 15% (p < 0.01) in the liver but decreased by 38% (p < 0.001) and 24% (p < 0.001) in the kidney and brain, respectively.
Changes in SOD activity in the livers, kidneys, and brains of SD rats after selenite cataract formation. The data are expressed as the mean ± standard deviation (n = 6 for each group). SeCa liver, kidney, and brain tissues at postnatal day 21 (the fifth day after eyelid opening) from rats injected with sodium selenite on postnatal days 8, 10, 11, and 12 (21 nmol/g BW). C liver, kidney, and brain tissues at postnatal day 21 (the fifth day after eyelid opening) from rats injected with equivalent volumes of normal saline on postnatal days 8, 10, 11, and 12. **p < 0.01 and ***p < 0.001, compared to the C group.
Effect of Selenite Cataract Formation on MsrA and MsrB1 Protein Expression Levels
Elevated levels of methionine sulfoxide (Met-O) have been detected in human cataractous lenses. Normally, Met-O can be reduced by the family of thioredoxin-dependent oxidoreductases, which includes MsrA and MsrB; these enzymes, respectively, reduce the S- and R-enantiomers of Met-O back to the reduced form Met [20]. As shown in Fig. 5, compared to that in the C group, the protein expression level of MsrA in the SeCa group was 17% higher (p < 0.05) in the liver, 30% lower (p < 0.05) in the brain, and the same in the kidney. However, the protein expression level of MsrB1 was decreased by 34% (p < 0.01) in the liver, increased by 42% (p < 0.05) in the kidney, and unchanged in the brain in the SeCa group compared with the C group.
Changes in MsrA (a) and MsrB1 (b) protein expression levels in the livers, kidneys, and brains of SD rats after selenite cataract formation. The data are expressed as the mean ± standard deviation (n = 6 for each group). SeCa liver, kidney, and brain tissues at postnatal day 21 (the fifth day after eyelid opening) from rats injected with sodium selenite on postnatal days 8, 10, 11, and 12 (21 nmol/g BW). C liver, kidney, and brain tissues at postnatal day 21 (the fifth day after eyelid opening) from rats injected with equivalent volumes of normal saline on postnatal days 8, 10, 11, and 12. *p < 0.05 and **p < 0.01, compared to the C group
Effect of Selenite Cataract Formation on MDA Levels
ROS-induced lipid peroxidation is one of the basic mechanisms of lens opacity in selenite cataract models [21]. MDA, a ROS-induced lipid peroxidation product, is considered to be an indicator of oxidative stress. Compared to those in the C group, the MDA levels in the SeCa group were 21% lower (p < 0.01) and 20% lower (p < 0.01) in the liver and kidney, respectively, but 10% higher (p < 0.01) in the brain (Fig. 6).
Changes in MDA levels in the livers, kidneys, and brains of SD rats after selenite cataract formation. The data are expressed as the mean ± standard deviation (n = 6 for each group). SeCa liver, kidney, and brain tissues at postnatal day 21 (the fifth day after eyelid opening) from rats injected with sodium selenite on postnatal days 8, 10, 11, and 12 (21 nmol/g BW). C liver, kidney, and brain tissues at postnatal day 21 (the fifth day after eyelid opening) from rats injected with equivalent volumes of normal saline on postnatal days 8, 10, 11, and 12. **p < 0.01, compared to the C group
Discussion
Selenite cataracts, which are animal models used to simulate human senile cataracts, are formed by injecting selenite into neonatal rats before their eyelids have opened. The application of these cataract models has been critical for studying the effects of various stresses on the eye lens, investigating the mechanisms of cataract formation, and screening potential anticataract drugs [7]. Sodium selenite injection induces severe oxidative damage in rat eye lenses, resulting in cataract formation.
In the present study, we investigated whether sodium selenite leads to oxidative damage in other important organs, such as the liver, kidney, and brain, and thus causes pathological changes in these organs.
Our findings suggested that GPx, TrxR, and SOD activity and MsrA protein expression were increased significantly in the livers of selenite cataract model rats, while MsrB1 protein expression and MDA levels (indicating lipid peroxidation) were both decreased significantly. Histopathological detection by H&E staining showed the presence of slight swelling and nonsignificant scattered spotty necrosis, which may have been associated with metabolism of sodium selenite in the liver followed by slight toxicity.
In the kidney, TrxR activity and MsrB1 protein expression were increased significantly in the SeCa group compared with the C group, while SOD activity and MDA levels were decreased significantly; however, GPx activity and MsrA protein expression did not differ between the groups. Furthermore, there were no visible pathological changes, as evidenced by H&E staining.
In the brain, GPx and SOD activity and MsrA protein expression levels were decreased significantly in the SeCa group, TrxR activity and MsrB1 protein expression did not differ between the groups, and MDA levels were increased significantly in the SeCa group. In addition, histopathological analysis by H&E staining showed mild gliocyte proliferation and significant disorder of neuronal arrangement with acidophilic changes in the hippocampus and significant occurrence of focal microglia or lymphocytic infiltration in the corpus striatum. Since it was very difficult to precisely dissect the whole brain into three parts (the hippocampus, corpus striatum, and cerebellum) for separate biochemical assays, whole brains were used for measurement of GPx, TrxR, and SOD activity and lipid peroxidation and for Western blot analysis of MsrA and MsrB1. Obvious pathological changes and significant increases in MDA levels in the brain indicated that subcutaneous injection of sodium selenite (21 nmol/g BW) into the napes of rat pups induced severe oxidative damage in the brain. GPx activity decreased significantly after selenite cataract formation; in contrast, TrxR activity did not change in the brain but increased significantly in the liver and kidney. These results imply that the expression of some important selenoproteins is of low priority in the brain; thus, pathological changes appeared quickly because of low antioxidant capacity.
We also found that the selenite cataract rat pups showed some behavioral abnormalities: they often shook their heads and sometimes rushed straight forward, which seemed to indicate some stimulation of the nervous system. The hippocampus is an important functional area in the brain; therefore, the pathological changes in the hippocampus in our histopathological examination may have been related to the behavioral abnormalities of the selenite cataract rats. However, we cannot ignore the fact that the rat pups in the SeCa group were actually blind because of the cataracts induced by sodium selenite injection. Therefore, it will be difficult but important to distinguish whether the behavioral abnormalities are induced by stimulation of the nervous system or are a result of blindness.
In general, providing selenium to selenium-deficient subjects increases GPx activity. When mice are fed a basal diet supplemented with 0.1 mg/kg Se as sodium selenite, GPx1 mRNA expression in the liver increases by more than 2.2-fold [22]. In line with this finding, a study by Ahmadvand H. et al. [23] showed that sodium selenite possesses antioxidant activity and has beneficial effects, attenuating reductions in serum, renal, and liver antioxidant enzyme (GPx and CAT) levels in rats with alloxan-induced diabetes. Moreover, Thirunavukkarasu C. et al. [24] found that Se levels and GPx activity are both increased in nodular tissue, surrounding liver cells and other organs in Se-supplemented rats. All these findings demonstrate the chemopreventive and chemotherapeutic effects of selenite. On the other hand, selenium overexposure decreases GPx activity; although the mechanism has not been well clarified, it probably involves oxidative effects of selenite ions resulting from increased formation of free radicals [25]. In our study, injection of rat pups with selenite to induce cataract formation significantly increased GPx activity in the liver, significantly decreased GPx activity in the brain, and did not affect GPx activity in the kidney, indicating that selenite exerts its effects in these organs through different mechanisms.
There have been no reports on the effects of sodium selenite dietary supplementation or injection on TrxR activity in selenite cataract model animals; thus, we performed this work to address the lack of knowledge. In our study, TrxR activity was increased significantly in the liver and kidney but showed no change in the brain upon selenite treatment. Interestingly, although both TrxR and GPx are selenium-containing antioxidant enzymes, they showed different changes in the kidney and brain after selenite injection for cataract formation. It will be necessary to study the mechanism of this phenomenon in future work.
MsrA has been linked to the aging process and has been shown to extend lifespan in animals. MsrA knockout mice have been shown to have 40% shorter lifespan than wild-type mice [26], while overexpression of MsrA in Drosophila melanogaster has been shown to increase lifespan by up to 70% [27]. Previous quantitation of MsrB1 mRNA expression has indicated that it ranks in the following order: liver, kidney, heart, and brain [28]. These findings are also consistent with the MsrB1 protein expression in these organs in the mice in the current study, as analyzed by Western blot assays. Our results showed that compared to that in the control rats, MsrA protein expression in the selenite cataract rats was increased significantly in the liver, decreased significantly in the brain, and not different in the kidney. However, MsrB1 protein expression was decreased significantly in the liver, increased significantly in the kidney, and not different in the brain in the cataract rats compared with the control rats. These results show the different influential mechanisms of selenite on different organs and the different effects of selenite on Msr protein expression.
Kumari et al. [29] found that mean SOD enzyme activity is significantly reduced in hemolysate during sodium selenite-mediated cataractogenesis. A similar tendency of change appeared in the rat kidneys and brains in our study. Ojeda et al. [30] found that the activity of the hepatic antioxidant enzymes GPx and SOD significantly increases in rats after Se dietary supplementation. The same tendency of change appeared in the rat livers in our study. In line with these findings, Shilo et al. [31] found that sodium selenite supplementation dose-dependently upregulates MnSOD expression significantly in rat livers.
Since selenite is a strong oxidant, selenite-induced oxidative stress may result in proteolytic precipitation, aggregation of soluble proteins into insoluble proteins, and lipid peroxidation (as reflected by increases in MDA) [6, 32]. Indeed, MDA levels have been shown to be significantly increased in selenite-injected model rats [32, 33]. In our study, MDA levels were decreased significantly in the liver and kidney but increased significantly in the brain in model rats. These results show that oxidative stress induced by selenite injection resulted in different physiological changes in the selenite cataract model animals and that the brain was more susceptible to oxidative damage than other organs.
In a study by Musik I. et al. [34], sodium selenite was found to insignificantly increase the activity of the antioxidant enzymes GPx and SOD in rat brains, and insignificant increases in glutathione (GSH) levels were also observed. However, paradoxically, decreases in total antioxidant status (TAS) could hardly be associated with increases in any other studied antioxidants, since TAS was insignificantly decreased in the brain. In our study, the activity of the antioxidant enzymes GPx and SOD and the protein expression levels of MsrA were decreased significantly in selenite cataract rat brains, but the activity of TrxR and the protein expression levels of MsrB1 did not differ between the control and cataract rats. Accordingly, the lipid peroxidation levels (MDA levels) were increased significantly in the model rats. This implies a distinct relationship with oxidative damage in the hippocampus and corpus striatum of selenite cataract rat brains.
In summary, in the selenite cataract rat model, GPx activity was increased significantly in the liver, decreased significantly in the brain, and unchanged in the kidney. The same tendency was observed for MsrA protein expression. TrxR activity in model rats was increased significantly in the liver and kidney but unchanged in the brain. SOD activity was increased significantly in the liver but decreased significantly in the kidney and brain. MsrB1 protein expression was decreased significantly in the liver and increased significantly in the kidney but remained unchanged in the brain. MDA levels were decreased significantly in the liver and kidney but increased significantly in the brain.
Conclusion
In our previous study, we discovered that large amounts of selenite can enter the eyes before eyelid opening, severely injuring the lens and resulting in cataract formation, because of the immaturity of the blood-retinal barrier (BRB). Moreover, the smaller amounts of selenite that enter the eyes after eyelid opening because of the mature BRB are insufficient to induce cataract formation [12]. Based on our previous study on eye lenses, we conducted a comprehensive toxicological evaluation with a selenite cataract rat model.
This study showed that sodium selenite induced severe oxidative damage, especially in the hippocampus and corpus striatum of the brain, in SD rats. Specifically, mild gliocyte proliferation, significant disorder of neuronal arrangement with acidophilic changes in the hippocampus, and significant occurrence of focal microglia or lymphocytic infiltration in the corpus striatum were observed after selenite injection for cataract simulation. This damage was closely related to significant decreases in the expression and activity of antioxidant enzymes and significant increases in lipid peroxidation (MDA levels). Furthermore, nonsignificant swelling and scattered spotty necrosis in the liver were observed. These results imply that physiological changes in model animals should be considered when carrying out anticataract drug screening and that pathological changes in other nontarget organs should be prevented.
References
Tolbert WD, Subedi GP, Gohain N, Lewis GK, Patel KR, Barb AW et al (2019) From rhesus macaque to human: structural evolutionary pathways for immunoglobulin G subclasses. mAbs 11:709–724. https://doi.org/10.1080/19420862.2019.1589852
Swearengen JR (2018) Choosing the right animal model for infectious disease research. Animal Model Exp Med 1:100–108. https://doi.org/10.1002/ame2.12020
Xiaoguang W, Jianjun C, Qinying C, Hui Z, Lukun Y, Yazhen S (2018) Establishment of a Valuable Mimic of Alzheimer's Disease in Rat Animal Model by Intracerebroventricular Injection of Composited Amyloid Beta Protein. J Vis Exp:56157. https://doi.org/10.3791/56157
World Health Organization (2018) Priority eye diseases. In: Blindness and vision impairment prevention. https://www.who.int/blindness/causes/priority/en/index1.html. Accessed 18 Apr 2018
Khan SA, Choudhary R, Singh A, Bodakhe SH (2016) Hypertension potentiates cataractogenesis in rat eye through modulation of oxidative stress and electrolyte homeostasis. J Curr Ophthalmol 28:123–130. https://doi.org/10.1016/j.joco.2016.05.001
Shearer TR, Ma H, Fukiage C, Azuma M (1997) Selenite nuclear cataract: review of the model. Mol Vis 3:8
Kyselova Z (2010) Different experimental approaches in modelling cataractogenesis: an overview of selenite-induced nuclear cataract in rats. Interdiscip Toxicol 3:3–14
Ma Y, Wu M, Li D, Li XQ, Li P, Zhao J, Luo MN, Guo CL, Gao XB, Lu CL, Ma X (2012) Embryonic developmental toxicity of selenite in zebrafish (Danio rerio) and prevention with folic acid. Food Chem Toxicol 50:2854–2863. https://doi.org/10.1016/j.fct.2012.04.037
Melčová M, Száková J, Mlejnek P, Zídek V, Fučíková A, Praus L, Zídková J, Mestek O, Kaňa A, Mikulík K, Tlustoš P (2018) The effect of zinc and/or vitamin E supplementation on biochemical parameters of selenium-overdosed rats. Pol J Vet Sci 21:731–740
Lohr JW, Willsky GR, Acara MA (1998) Renal Drug Metabolism. Pharmacol Rev 50:107–141
Babizhayev MA (2016) Generation of reactive oxygen species in the anterior eye segment. Synergistic codrugs of N-acetylcarnosine lubricant eye drops and mitochondria-targeted antioxidant act as a powerful therapeutic platform for the treatment of cataracts and primary open-angle glaucoma. BBA Clin 6:49–68. https://doi.org/10.1016/j.bbacli.2016.04.004
Chen H, Tian W, Huang K (2013) Effect of blood-retinal barrier development on formation of selenite nuclear cataract in rat. Toxicol Lett 216:181–188. https://doi.org/10.1016/j.toxlet.2012.11.016
Hafeman DG, Sunde RA, Hoekstra WG (1974) Effect of dietary selenium on erythrocyte and liver glutathione peroxidase in the rat. J Nutr 104:580–587. https://doi.org/10.1093/jn/104.5.580
Brandstaedter C, Fritz-Wolf K, Weder S, Fischer M, Hecker B, Rahlfs S, Becker K (2018) Kinetic characterization of wild-type and mutant human thioredoxin glutathione reductase defines its reaction and regulatory mechanisms. FEBS J 285:542–558. https://doi.org/10.1111/febs.14357
Chu S, Wang L, Mao X, Peng W (2016) Improvement of Huangqi decoction on endothelial dysfunction in 5/6 Nephrectomized rats. Cell Physiol Biochem 40:1354–1366. https://doi.org/10.1159/000453188
Zeng J, Zhou J, Huang K (2009) Effect of selenium on pancreatic proinflammatory cytokines in streptozotocin-induced diabetic mice. J Nutr Biochem 20:530–536. https://doi.org/10.1016/j.jnutbio.2008.05.012
Das JK, Sarkar S, Hossain SU, Chakraborty P, Das RK, Bhattacharya S (2013) Diphenylmethyl selenocyanate attenuates malachite green induced oxidative injury through antioxidation & inhibition of DNA damage in mice. Indian J Med Res 137:1163–1173
Boujbiha MA, Hamden K, Guermazi F, Bouslama A, Omezzine A, Kammoun A, el Feki A (2009) Testicular toxicity in mercuric chloride treated rats: association with oxidative stress. Reprod Toxicol 28:81–89. https://doi.org/10.1016/j.reprotox.2009.03.011
Ray G, Husain SA (2002) Oxidants, antioxidants and carcinogenesis. Indian J Exp Biol 40:1213–1232
Kim HY, Gladyshev VN (2007) Methionine sulfoxide reductases: selenoprotein forms and roles in antioxidant protein repair in mammals. Biochem J 407:321–329
David LL, Shearer TR (1984) State of sulfhydryl in selenite cataract. Toxicol Appl Pharmacol 74:109–115. https://doi.org/10.1016/0041-008X(84)90276-X
Qin S, Huang K, Gao J, Huang D, Cai T, Pan C (2009) Comparison of glutathione peroxidase 1 and iodothyronine deiodinase 1 mRNA expression in murine liver after feeding selenite or selenized yeast. J Trace Elem Med Biol 23:29–35. https://doi.org/10.1016/j.jtemb.2008.11.001
Ahmadvand H, Ghasemi Dehnoo M, Cheraghi R, Rasoulian B, Ezatpour B, Azadpour M, Baharvand K (2014) Amelioration of altered serum, liver, and kidney antioxidant enzymes activities by sodium selenite in alloxan-induced diabetic rats. Rep Biochem Mol Biol 3:14–20
Thirunavukkarasu C, Premkumar K, Sheriff AK, Sakthisekaran D (2008) Sodium selenite enhances glutathione peroxidase activity and DNA strand breaks in hepatoma induced by N-nitrosodiethylamine and promoted by phenobarbital. Mol Cell Biochem 310:129–139. https://doi.org/10.1007/s11010-007-9673-5
Dougherty JJ, Hoekstra WG (1982) Stimulation of lipid peroxidation in vivo by injected selenite and lack of stimulation by selenate. Proc Soc Exp Biol Med 169:209–215
Moskovitz J, Bar-Noy S, Williams WM, Requena J, Berlett BS, Stadtman ER (2001) Methionine sulfoxide reductase (MsrA) is a regulator of antioxidant defense and lifespan in mammals. Proc Natl Acad Sci U S A 98:12920–12925. https://doi.org/10.1073/pnas.231472998
Ruan H, Tang XD, Chen ML, Joiner ML, Sun G, Brot N, Weissbach H, Heinemann SH, Iverson L, Wu CF, Hoshi T (2002) High-quality life extension by the enzyme peptide methionine sulfoxide reductase. Proc Natl Acad Sci U S A 99:2748–2753. https://doi.org/10.1073/pnas.032671199
Liang X, Fomenko DE, Hua D, Kaya A, Gladyshev VN (2010) Diversity of protein and mRNA forms of mammalian methionine sulfoxide reductase B1 due to intronization and protein processing. PLoS One 5:e11497. https://doi.org/10.1371/journal.pone.0011497
Kumari RP, Anbarasu K (2014) Protective role of C-phycocyanin against secondary changes during sodium selenite mediated cataractogenesis. Nat Prod Bioprospect 4:81–89. https://doi.org/10.1007/s13659-014-0008-4
Ojeda ML, Nogales F, Vázquez B, Delgado MJ, Murillo ML, Carreras O (2009) Alcohol, gestation and breastfeeding: selenium as an antioxidant therapy. Alcohol Alcohol 44:272–277. https://doi.org/10.1093/alcalc/agp004
Shilo S, Pardo M, Aharoni-Simon M, Glibter S, Tirosh O (2008) Selenium supplementation increases liver MnSOD expression: molecular mechanism for hepato-protection. J Inorg Biochem 102:110–118. https://doi.org/10.1016/j.jinorgbio.2007.07.027
Orhan H, Marol S, Hepsen IF, Sahin G (1999) Effects of some probable antioxidants on selenite-induced cataract formation and oxidative stress-related parameters in rats. Toxicology 139:219–232. https://doi.org/10.1016/S0300-483X(99)00128-6
Li N, Zhu Y, Deng X, Gao Y, Zhu Y, He M (2011) Protective effects and mechanism of tetramethylpyrazine against lens opacification induced by sodium selenite in rats. Exp Eye Res 93:98–102. https://doi.org/10.1016/j.exer.2011.05.001
Musik I, Kiełczykowska M, Kocot J (2013) Oxidant balance in brain of rats receiving different compounds of selenium. Biometals 26:763–771. https://doi.org/10.1007/s10534-013-9654-y
Acknowledgments
The authors wish to thank Prof. Kaixun Huang of Huazhong University of Science and Technology, P. R. China for his suggestion in the biochemical analysis.
Funding
This work was supported by grants from “Opening fund of Hubei Key Laboratory of Bioinorganic Chemistry & Materia Medica (No. BCMM201703)” and “Ph.D. research fund of Wuhan Technology and Business University (No. D2015004)”.
Author information
Authors and Affiliations
Corresponding author
Ethics declarations
Conflict of Interest
The authors declare that they have no conflict of interest.
Ethical Approval
All animal experiments complied with the ARRIVE guidelines and were carried out in accordance with the National Institutes of Health Guide for the Care and Use of Laboratory Animals (NIH Publication No. 8023, revised 1978). All experimental procedures involving animals were approved by Scientific Research Department of Wuhan Technology and Business University.
Additional information
Publisher’s Note
Springer Nature remains neutral with regard to jurisdictional claims in published maps and institutional affiliations.
Highlights
• Significant pathological changes occurred in the hippocampus and corpus striatum in the brain in cataract model rats.
• GPx, SOD, and MsrA levels were significantly decreased in the brains of selenite cataract model rats.
• Decreases in GPx, SOD, and MsrA levels and increases in MDA levels caused pathological changes.
• Pathological changes should be taken seriously during anticataract drug screening.
Electronic supplementary material
ESM 1
(PDF 1099 kb)
Rights and permissions
About this article
Cite this article
Chen, H., Zhou, J. Effects of Sodium Selenite on Oxidative Damage in the Liver, Kidney and Brain in a Selenite Cataract Rat Model. Biol Trace Elem Res 197, 533–543 (2020). https://doi.org/10.1007/s12011-019-02000-1
Received:
Accepted:
Published:
Issue Date:
DOI: https://doi.org/10.1007/s12011-019-02000-1