Abstract
In this study, two different food-grade enzymes (i.e., bromelain from a pineapple stem (protease) and Pectinex® BE XXL (pectinase)) were successfully immobilized on chitosan beads and their application in pomegranate juice clarification was evaluated. The immobilization procedure was optimized for maximizing the specific activity of biocatalysts, and the best performance was reached using an immobilization solution containing 1.0 mgBSAeq/mL (for protease) and 1.8 mgBSAeq/mL (for pectinase). The biocatalysts were combined in a multi-enzymatic system and used in a fluidized bed reactor, varying the protease-to-pectinase ratio (1:2 or 1:4) and the treatment time (4 h or 8 h). The process carried out using the protease-to-pectinase ratio 1:2, for 8 h, was the most suitable in terms of immediate (− 49%) and potential (− 70%) turbidity depletion compared with the untreated juice, after 21 days. At the end of the storage period, this biotechnological approach allowed a significant reduction of haze-active molecules. All the enzymatically treated juices better preserved the anthocyanin pattern compared with the untreated juice over time. The best supplied treatment allowed better retaining the native chromatic properties of juice, preserving it from colloidal instability as well as from the possible related color degradation tendency.
Similar content being viewed by others
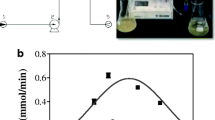
Explore related subjects
Discover the latest articles, news and stories from top researchers in related subjects.Avoid common mistakes on your manuscript.
Introduction
In the last few years, there has been an increased demand for pomegranate juice (Erkan 2011) due to its nutritional properties (Kalaycıoğlu and Erim 2016), as well as the presence of anti-carcinogenic (Bell and Hawthorne 2008), anti-microbial (Reddy et al. 2007), and anti-atherosclerotic compounds (Aviram et al. 2004).
One of the main problems, occurring throughout the production of fruit juices (especially pomegranate juice), is the presence of colloidal suspensions and/or sediments that make the product turbid and cloudy. The absence of clarity and transparency is due to the dispersion of the light beam when it hits suspended particles, thus resulting in the phenomenon known as “light scattering” (Siebert 2006). Two different types of turbidity have been described in literature, depending on the molecules and mechanisms involved in their formation: (i) “immediate turbidity,” referring to turbidity and sediment caused by polysaccharide suspensions (i.e., pectin, cellulose, hemicellulose, starch, and lignin) in fresh fruit juices (Sorrivas et al. 2006), and (ii) “haze formation,” usually occurring during juice bottle storage, mainly ascribable to protein-polyphenol complexes, which aggregate giving rise to a hydrophobic core surrounded by pectin molecules (Grassin and Fauquembergue 1996; Pinelo et al. 2010; Siebert et al. 1996; Siebert 2006).
Clarification is a crucial step during pomegranate juice processing, in order to eliminate the substances responsible for turbidity, also improving the appearance and marketability of the final product (Vardin and Fenercioglu 2003; Mirzaaghaei et al. 2016; Onsekizoglu 2013). In this context, the traditional approaches applied are based on the addition of clarifying agents (i.e., gelatin, polyvinylpolypyrrolidone (PVPP), albumin, and bentonite) and subsequent filtration/centrifugation. Several studies proved that the use of these compounds significantly affects anthocyanin levels (Vardin and Fenercioglu 2003; Turfan et al. 2012; Erkan-Koç et al. 2015) with a consequent degradation of chromatic properties of pomegranate juice (Turfan et al. 2012; Turfan et al. 2011).
There are also numerous studies on the application of ultrafiltration (UF) for clarification of pomegranate and other fruit juices. Among these, Gulec et al. (2017) evaluated the performance of UF, based on different membranes, proving that the major limits of this technique for large-scale use are (i) membrane fouling and (ii) color alterations of treated juice, due to the presence of high amount of browning compounds. Zhao et al. (2014) combined UF (using ceramic membrane) with high-pressure processing to clarify fresh apple juice, finding a significant decrease in total polyphenol content. Moreover, during storage, Zhao et al. (2014) proved that the clarity in treated samples gradually decreased probably due the polymerization of phenolic compounds with proteins that still remained in juice.
Recently, the combined use of free proteases and pectinases appeared a useful biotechnological tool for the reduction of immediate turbidity and haze formation in pomegranate juice during storage (Cerreti et al. 2016; Cerreti et al. 2017). However, it is recognized that free enzymes usually have poor stability under process conditions (i.e., pH, temperature, and natural inhibitors in food matrixes) and they cannot be recovered/reused. Their immobilization on solid supports could have positive effects on catalytic stability (Petenzi et al. 2012; Bavaro et al. 2016; Miao et al. 2012), allowing an easy separation from the reaction mixture, the recycling/continuous use of biocatalysts (O’Halloran et al. 2019), and the modulation of catalytic properties, avoiding protein contamination of the product (Gardossi et al. 2008; Karangwa et al. 2012; Cacciotti et al. 2019).
Recently, Jana et al. (2015) immobilized tannase on biocompatible substance for quality improvement of fruit juice by an adsorption-entrapment technique. The results revealed some critical issues: (I) about 58% of the enzyme was lost during immobilization in the Ca-alginate matrix through entrapment, (II) the color attribute of juice was reduced to about 31–35%, and (III) the process was conducted in batch mode rather than in continuous mode.
Despite numerous studies that investigated the effect of immobilized pectinases or proteases for fruit juice clarification (Di Cosimo et al. 2013; Deng et al. 2019), to the best of our knowledge, no research has been conducted on their combined use, in immobilized form, applied into continuous bioreactors.
To fill the gap, this investigation deals with the study on the development of a food-grade multi-enzymatic system, based on proteases and pectinases (immobilized on chitosan carriers) for exploiting their synergistic action in the selective hydrolysis of haze-active molecules in pomegranate juice, without affecting chromatic properties. This novel treatment has been carried out, for the first time, into a fluidized bed reactor (FBR), varying the protease-to-pectinase ratio (1:2 or 1:4) and the treatment time (4 h or 8 h). The evolution of haze-active molecules in pomegranate juice, as well as the changes of its chromatic properties, has been monitored during 21-day bottle storage at 4 °C.
Materials and Methods
Materials
The selected protease, i.e., bromelain from pineapple stem (EC number 3.4.22.32) (BR), was purchased from Sigma-Aldrich (Milan, Italy), whereas the pectinase, i.e. Pectinex® BE XXL (in liquid form, produced by Novozymes, Bagsvaerd, Denmark), was kindly supplied by Univar Inc. (Milan, Italy). The synthetic substrate Bz-Phe-Val-Arg-pNA, containing para-nitroaniline (pNA), used to test the protease activity, was purchased from Bachem (Germany). Pectins from citrus peel (used to assay the pectin-liasic activity) and the polygalacturonic acid (for testing the polygalacturonic activity) were supplied by Sigma-Aldrich (Milan, Italy). Chitosan powder from Aspergillus niger (KiO Nutrime-CsG, lot no. 12121611L4) was provided by KitoZyme S.A. (Herstal, Belgium), and polydialdehyde starch (polymeric dialdehyde), used as a cross-linker, was purchased from Carbosynth Limited (UK). The pomegranates were bought in a local market in Viterbo (Lazio, in Central Italy).
Chitosan Bead Preparation and Characterization
The chitosan beads, used as immobilization carriers, were prepared according to the precipitation method with some modification as reported by Cappannella et al. (2016). Chitosan powder was dissolved in an aqueous solution of acetic acid (5% v/v) and kept, under stirring conditions, until complete solubilization was achieved. The solution was then added dropwise, through a peristaltic pump (Minipuls 3, Gilson, Italy), into a gently stirred coagulation liquid (2 M sodium hydroxide and 26% v/v ethanol). The obtained beads were filtered and washed with distilled water (Birò et al. 2008).
The morphology and the surface roughness of the obtained beads were investigated with a field emission gun scanning electron microscope (FEG-SEM, Cambridge Leo Supra 35, Carl Zeiss), after drying at 40 °C for 48 h in an oven and sputter-coating with gold under an argon atmosphere (25 mA, 120 s). The thermal properties and the occurred cross-linking were also studied by differential scanning calorimetry (DSC, TA Instruments Q2000) in the following conditions: sample weight ~ 5 mg, nitrogen atmosphere (N2 flow rate 50 cc/min), range of temperature 20–400 °C, and heating rate 10 °C/min.
Immobilization Procedure
The functionalization of chitosan beads with polydialdehyde starch cross-linker was carried out as described by Chen et al. (2010) and Cappannella et al. (2016). The activated beads were collected and washed with distilled water. For each enzyme, different immobilization solutions with increasing protein concentration (0.25–4.00 mgBSAeq/mL) were prepared in 0.2 M sodium phosphate buffer (pH 7). One gram of carrier was placed in contact with 10 mL of the different immobilization solutions and left under shaking at 150 rpm, overnight at 20 °C. Then, the unbound proteins were removed from the carriers by extensively washing with 2 M ammonium sulfate and 0.1 M citrate buffer (pH 3.6). The amount of immobilized protein was indirectly calculated, as the difference between the proteins initially present in the immobilization solution and those found in the supernatant, quantified by the Bradford colorimetric assay (Bradford 1976), using BSA as the standard protein.
Polyacrylamide Gel Electrophoresis
In order to prove the stability of the covalent linkage between the functionalized chitosan beads and the enzyme, the presence of proteins into the last washing solution was investigated by SDS-PAGE (Laemmli 1970), using precast commercial gels of 4–15% (Bio-Rad, Richmond, California, USA). All samples were prepared adding 20 μL of the last washing solution to 20 μL of loading buffer (4× Laemmli sample buffer) and then boiling at 90 °C for 5 min. After the run, the proteins were stained with a 0.1% solution of Coomassie Brilliant Blue G-250.
Bromelain Activity Assay
BR activity, in free and immobilized (1 g biocatalyst, wet weight) form, was evaluated in model pomegranate juice (0.1 M citrate buffer, pH 3.6) using the synthetic substrate Bz-Phe-Val-Arg-pNA (250 μM). The activity assays were conducted, monitoring the absorbance variation vs time (over 2 min for free enzyme and over 10 min for immobilized protease) at 410 nm (A410), using a UV-visible spectrophotometer (Shimadzu UV 2450, Milan, Italy). The latter biocatalyst was kept under shaking at 80 rpm on a Loopster Digital Rotating Mixer (IKA-LOOPSTER digital), and the reaction mixture was removed after 1, 2, 3, 4, 5, 8, and 10 min for the spectrophotometric measurement. The specific activity was calculated in IU of pNA produced (εmmol = 8.480 1/mM cm for pNA, as reported by Hale et al. 2005), and it was expressed as IU per milligram of free or immobilized protein (IU/mgBSAeq). A blank correction was carried out using a sample without the enzyme. All measurements were taken in triplicate.
Pectinex® BE XXL Activity Assay
Taking into account data reported on a technical sheet, Pectinex® BE XXL activity (in free and immobilized (1 g biocatalyst, wet weight) form) was characterized by both polygalacturonase (PG) and pectin lyase (PL) activity assays, which were conducted in model pomegranate juice. As described for BR, PL activity was monitored over 2 min for free enzyme and over 10 min for the immobilized form (keeping under shaking at 80 rpm on a Loopster Digital Rotating Mixer (IKA-LOOPSTER digital)). PG activity was tested over 10 min for both free and immobilized forms.
PG activity, measured using polygalacturonic acid (1% w/v in model juice) as substrate, was tested according to the 3′-5′-dinitrosalicylic (DNS) acid method (Kashyap et al. 2000) and monitoring the absorbance variation at 530 nm (A530), which results from the galacturonic acid released from the substrate (Miller 1959). PL activity was evaluated using pectin from a citrus peel (1% w/v in model juice) as substrate. The assay was carried out spectrophotometrically, monitoring the increase in absorbance at 235 nm (A235) due to the formation of a conjugated double bond of the Δ4:5 unsaturated uronide formed during the reaction (Busto et al. 2006). The specific activity was expressed as IU per milligram of free or immobilized protein, calculated according to the equation described by Albersheim (1966).
Kinetic Characterization of Free and Immobilized Enzymes
In order to compare the catalytic activity of free and immobilized enzymes, their kinetic characterization was performed in model pomegranate juice, using different concentrations of each substrate (Bz-Phe-Val-Arg-pNA, 0–500 μM for BR; polygalacturonic acid, 0–15 mg/mL for PG; pectin from a citrus peel, 0–10 mg/mL for PL). Kinetic parameters (Vmax (maximum velocity at which the enzyme catalyzes the reaction) and KM (Michaelis-Menten constant)) were estimated according to the Michaelis-Menten equation, using a non-linear regression procedure (GraphPad Prism 5.01, GraphPad Software, Inc.). Moreover, catalytic constant or turnover number (kcat = Vmax/[E]tot, where [E]tot is the molar enzyme concentration) and affinity constant (Ka = kcat/KM) were calculated. The goodness of fit for each data set (to their best fit theoretical kinetic curves) was assessed as the square of the correlation coefficients (R2).
Optimization of Process Parameters in a Fluidized Bed Reactor
The FBR consisted of a glass column (total volume of about 300 mL), equipped with an external water jacket for temperature control and connected to a thermostat (MPM Instrument Type M 900-TI). The nitrogen (N2) gas insufflation (1-bar flow) from the bottom of the column and the use of a peristaltic pump (Heidolph Pumpdrive 5206) allowed the suspension of the immobilized biocatalysts, as well as the recirculation of the model or real pomegranate juice.
In order to maximize the product release rate (RP) of the immobilized multi-enzymatic system, for the continuous treatment of pomegranate juice in FBR, preliminary trials were conducted in model pomegranate juice at 30 °C. In detail, 5 g (wet weight) of immobilized BR or immobilized Pectinex® BE XXL was placed in the FBR containing model juice, fortified with 250 μM Bz-Phe-Val-Arg-pNA (for BR) or with 10 mg/mL polygalacturonic acid (for Pectinex® BE XXL). Thus, the continuous hydrolysis of each specific substrate was performed in FBR over 40 min. The flow rate (Qv, independent variable) was varied from 112 to 1064 mL/min in order to modify the recirculation of the model pomegranate juice, and for each immobilized biocatalyst (BR or Pectinex® BE XXL), the optimal flow rate was identified by evaluating the concentration of the product release rate (RP). Moreover, the following process parameters (dependent variables) were calculated: (i) space velocity (Sv given by the ratio between Qv and FBR volume), (ii) residence time (τ, corresponding to 1/Sv), (iii) concentration of product release (CP, given by the ratio between the ΔAbs/min and molar extinction coefficient), and (iv) product release rate (RP, corresponding to CP × Qv).
Preparation of Pomegranate Juice and Treatment in FBR
The pomegranate juice was prepared by pressing the fresh fruits, previously washed and cut in half, by means of a manual press. The resulting juice was pasteurized (90 °C for 1 min) in order to inactivate the endogenous enzymes, centrifuged (ROTINA 420 R, Hettich GmbH & Co) at 10,000 rpm for 15 min, and then analyzed (pH, 3.17, titratable acidity, 11.25 g/L (as anhydrous citric acid), soluble solid content, 15.60 °Bx). Afterwards, the samples were treated using two different protease-to-pectinase ratios (1:2 or 1:4) for 4 h or 8 h, in the FBR at 30 °C. The obtained juices were designed as follows: (i) control untreated juice, (ii) C1 ratio 1:2 for 4 h, (iii) C2 ratio 1:2 for 8 h (iv) C3 ratio 1:4 for 4 h, and (v) C4 ratio 1:4 for 8 h.
Turbidity Measurement and Heat Stability
The immediate turbidity of the juice measured using a HD 25.2 turbidimeter (Delta Hom, Padova, Italy) and expressed as nephelometric turbidity units (NTU) was evaluated right after the enzymatic treatment (t0) and after 7, 14, and 21 days of storage at 4 °C in the dark. The potential turbidity, which accounts for the haze formation tendency, was determined by the heat test (80 °C for 6 h and 4 °C for 16 h) (Vincenzi et al. 2011). The ΔNTU was calculated as the difference between the turbidity of the juice after (NTU2) and before (NTU1) the heat test (on samples at room temperature).
Haze-Active Molecule Determination
The haze forming tendency of pomegranate juice was evaluated by measuring the reactivity to gelatin and tannic acid, according to Cerreti et al. (2016). Two stock solutions were prepared by dissolving 6 g/L of gelatin and 5 g/L of tannic acid in distilled water. The reactivity of proteins and phenols was evaluated as described by Siebert et al. (1996) with appropriate modifications. For each determination, 0.4 mL of stock solution was added to 7.6 mL of juice. The samples were incubated at 25 °C for 30 min under constant stirring. The reactivity was determined by the difference between the turbidity (HD 25.2 turbidimeter) after and before the addition of each stock solution.
Spectrophotometric Analysis
Total anthocyanin index was evaluated recording the absorbance spectrum (230–700 nm) of pomegranate juice after diluting 1:30 with ethanol/water/HCl (70:30:1) (Baiano et al. 2009). The total anthocyanin index, expressed as milligrams per liter of cyanidine-3-glucoside, was calculated as
Total anthocyanin index (mg/L) = E × 15.17 × dilution factor where E is the maximum absorbance value between 536 and 540 nm and 15.17 is the factor deduced by the molar A of cyanidine-3-glucoside (ε = 29,600 1/mM cm; PM = 449.2 g/mol) (Di Stefano and Guidoni 1989).
Monomeric anthocyanin index was determined after juice elution through a polyvinylpyrrolidone (PVP) column, capable of retaining anthocyanin combined with tannins, with ethanol/water/HCl (70:30:1), as reported by Ribéreau-Gayon et al. (2000). The formula previously used for determining total anthocyanin content was also employed to calculate the monomeric anthocyanin index.
Color indexes of the juice were estimated using an UV-vis spectrophotometer (UV-2450 spectrophotometer, Shimadzu). The absorbance spectrum (230–700 nm) was recorded using a 1-mm quartz cuvette (Hellma, Milan, Italy). The following color indexes were calculated: color intensity (CI = A420 + A520 + A620), tonality (T = A420 / A520), and percentage of yellow (%Y = A420 / CI × 100), red (%R = A520 / CI × 100), and blue (%B = A620 / CI × 100) (Sepúlveda et al. 2010; Glories 1984).
Statistical Analysis
All determinations were conducted in triplicate, and the results were expressed as mean ± standard deviation. Data were analyzed using one-way analysis of variance (ANOVA) through the Excel® Add-in Macro DSAASTAT program, in order to test for significant differences between treatments. Tukey’s (HSD) post hoc test (confidence interval, 95%) was performed when significance was reached.
Results and Discussion
Characterization of Chitosan Beads
The occurred functionalization with polydialdehyde starch was demonstrated by observation with SEM of the bead surface before and after the reaction with the selected cross-linker and also by DSC measurements. In Fig. 1, high-magnification SEM micrographs of all the produced dried A. niger chitosan beads, before and after functionalization, are compared, in order to evidence the influence of the cross-linking on the surface roughness and topography. The obtained beads presented a rough surface both before and after the functionalization, even if the functionalization seemed to promote the obtainment of a more compact and uniform surface with respect to that before functionalization, characterized by the presence of typical island-like structures.
The efficient functionalization was further confirmed by DSC analyses. Heating scan DSC curves of chitosan beads before and after polydialdehyde starch cross-linking are compared in Fig. 2. As expected, the decomposition temperature (Sakurai et al. 2000; Zeng et al. 2004) is shifted at higher values in the case of cross-linked beads (e.g., 278 °C vs 297 °C for unfunctionalized and functionalized beads, respectively). Moreover, whereas in the case of unfunctionalized beads, a broad endothermic peak at approximately 100 °C was detected and ascribed to both the residual solvent removal and, mainly, to the dissociation process of chitosan interchain hydrogen bonding (Chuang et al. 1999), it was not revealed in the case of functionalized beads. This experimental evidence can be justified by the less moisture and testified the occurred cross-linking which led to stronger chitosan chains.
Moreover, the used cross-linker resulted more efficient than the glutaraldehyde, commonly used for chitosan cross-linking, since the latter one did not seem to significantly influence the chitosan surface morphology and led to a minor thermal decomposition increment (287 °C vs 297 °C for glutaraldehyde and polydialdehyde starch, respectively), as reported in one of our previous works (Benucci et al. 2016).
Enzyme Immobilization and Kinetic Characterization in Model Juice
The immobilization procedure of bromelain and Pectinex® BE XXL was optimized varying the initial protein concentration in the immobilization solution (0.25–4.0 mgBSAeq/mL) in contact with the functionalized chitosan beads.
The SDS-PAGE (conducted on the last washing solution after the immobilization) showed that, for both enzymes and regardless of the initial protein concentration in the immobilization solution, bands of molecular weight similar to those of the corresponding free forms (used as the reference standard) were not observed (Fig. 3). This result proves the stability of the covalent linkage between the functionalized carriers, based on chitosan from A. niger, and both enzymes.
SDS-PAGE of the last washing solution for evaluating the stability of the covalent linkage between the functionalized chitosan beads and the enzyme. Trials were conducted for bromelain (BR) and Pectinex® BE XXL, using different immobilization solutions with increasing protein concentration (0.25–4.00 mgBSAeq/mL)
Figure 4 shows the loading capacity of the carriers and the specific activity of the immobilized biocatalysts. For both enzymes, the immobilized proteins significantly increased with increasing concentration of the immobilization solution, to finally reach the highest value of 10 mgBSAeq/g carrier for BR and 5.5 mgBSAeq/g carrier for Pectinex® BE XXL (using an immobilization solution at 4.00 and 1.80 mgBSAeq/mL, respectively) (Fig. 4a, b). The different observed loading capacities could be ascribed to the different molecular weights of the enzymes (Cao 2005), as it appears by the carrier saturation revealed only for Pectinex® BE XXL, which is characterized by a higher molecular weight with respect to BR (25.6 kDa for BR; about 40 kDa and 50.7 kDa for Pectinex® BE XXL, Fig. 3).
Effect of the protein concentration in the immobilization solution (0.25–4.00 mgBSAeq/mL) on the immobilized protein (mgBSAeq/g carrier) and on the specific activity of immobilized biocatalysts. a BR (bromelain expressed as mIU/mgBSAeq). b Pectinex® BE XXL (PG—polygalacturonase activity and PL—pectin lyase activity, both expressed as IU/mgBSAeq). Reported data are mean ± SD of triplicate analysis. Values with different capital letters (A, B, C, D) differ significantly (Tukey’s test, p < 0.05) in terms of immobilized protein. Values with different small letters differ significantly (Tukey’s test, p < 0.05) in terms of specific activity (BR and PL (a, b, c, d) and PG (α, β, γ, δ))
Concerning the specific activity, it significantly went up with the increase in immobilized proteins to the maximum value and then it decreased (Fig. 4), probably due to steric hindrance phenomena, as also described for other biocatalysts (Tischer and Wedekind 1999). For BR, the highest specific activity (0.65 mIU/mgBSAeq) was revealed using an initial protein amount of 1.0 mgBSAeq/mL (Fig. 4a). Moreover, both activities associated with Pectinex® BE XXL (PG and PL) showed the maximum value (0.82 IU/mgBSAeq for PG and 0.35 IU/mgBSAeq for PL) at an initial protein concentration of 1.8 mgBSAeq/mL (Fig. 4b). Overall, these results prove that the use of an immobilization solution containing 1.0 mgBSAeq/mL (for BR) and 1.8 mgBSAeq/mL (for Pectinex® BE XXL) appeared the suitable condition for maximizing the specific activity of immobilized biocatalysts.
The kinetic study, carried out in model juice toward the specific substrate, demonstrated that all enzymes (both in free and immobilized forms) followed the hyperbolic behavior of the Michaelis-Menten equation (Fig. 5). As expected, the immobilization considerably reduced the catalytic properties of the biocatalyst, with a significant reduction of Vmax, kcat, and Ka which was revealed for BR, PG, and PL (Table 1). This reduction, after covalent immobilization, is a common phenomenon, and it could be attributable to steric hindrance and diffusion issues as well as to the low amount of enzyme immobilized on chitosan supports (Jiang et al. 2005).
Specific activity (S.A.) of a bromelain (BR), b polygalacturonase (PG, Pectinex® BE XXL), and c pectin lyase (PL, Pectinex® BE XXL) in free (■) and immobilized form (●). The kinetic study was carried out in model pomegranate juice, toward the specific substrate of each enzymatic activity (Bz-Phe-Val-Arg-pNA, 0–500 μM for BR; polygalacturonic acid, 0–15 mg/mL for PG; pectin from a citrus peel, 0–10 mg/mL for PL)
Optimization of Model Juice Treatment in a Fluidized Bed Reactor
Data in Table 2 show the process parameters of FBR, containing immobilized bromelain or immobilized Pectinex® BE XXL, obtained varying the flow rate (Qv). As reported by other authors (Saponjić et al. 2010; Laudani et al. 2007), the relationship between RP and Sv is represented by a bell-shape curve (Fig. 6), with a maximum of product formation rate (free pNA for BR and galacturonic acid for PG) at a Sv of 1.87 1/min, corresponding to a Qv of 560 mL/min and a τ of 0.54 min, for both biocatalysts (Table 2). It was observed that there is a positive linear relationship between Qv and Sv, whereas an inverse relationship between Qv and τ, following a negative hyperbolic curve, was revealed. The further increase in Sv, at values higher than 1.87 1/min, resulted in an evident reduction of the product formation rate, probably due to the greater turbulence at high flow rates, as well as to the reduction of contact-time between the immobilized enzyme and the substrate, leading to the decrease in the RP (Saponjić et al. 2010; Zhou et al. 2014).
Relationship between space velocity (Sv) and product formation rate (RP) in a fluidized bed reactor (FBR), containing immobilized bromelain (●, BR activity) or immobilized Pectinex® BE XXL (■, PG activity). FBR was fed at varying flow rates with model pomegranate juice fortified with 250 μM Bz-Phe-Val-Arg-pNA (for BR activity) or with 10 mg/mL polygalacturonic acid (for PG activity).
Effect of Multi-enzymatic Treatment on the Haze Stability of Pomegranate Juice During Storage
Immediate and Potential Turbidity
The immediate turbidity and the potential turbidity of pomegranate juice untreated and treated in the FBR, then stored for 21 days at 4 °C, are reported in Fig. 7 a and b, respectively.
Immediate turbidity (a) and potential turbidity (b) evaluated right after the enzymatic treatment (t0) and after 7, 14, and 21 days of storage at 4 °C in the dark. Samples were control (untreated juice), C1 (protease-to-pectinase ratio 1:2, treatment time in FBR 4 h), C2 (protease-to-pectinase ratio 1:2, treatment time in FBR 8 h), C3 (protease-to-pectinase ratio 1:4, treatment time in FBR 4 h), and C4 (protease-to-pectinase ratio 1:4, treatment time in FBR 8 h). Reported data are mean ± SD of triplicate analysis of juice samples. For each storage time, data with different letters differ significantly (Tukey’s test, p < 0.05) (t0 (A, B, C, D), 7 days (a, b, c, d), 14 days (α, β, γ, δ), and 21 days (W, X, Y, Z))
By comparing data right after the enzymatic treatment (t0), it appears that the immediate turbidity of the control was approximately 30 NTU and the multi-enzymatic treatment in FBR had no significant effects, except for the C2 sample, for which this parameter decreased to a value of about 20 NTU (Fig. 7a). During storage, the immediate turbidity gradually increased in control (+ 30% after 14 days) and then it remained almost constant. A similar trend was observed in C3, although the increase in immediate turbidity (observed until the end of storage time) resulted in a lesser extent (+ 19% after 21 days). Concerning C1 and C4, a decrease in this parameter was observed after 7 days and it did not change further in the subsequent period. At the end of storage, both C1 and C4 showed lower immediate turbidity than the control (about − 20%), whereas in C2 a significant reduction was revealed right after the treatment in FBR (− 35% with respect to control) and then it remained constant (Fig. 7a). These data prove the efficiency of the multi-enzymatic treatment in FBR in reducing the immediate turbidity of pomegranate juice after 21 days of storage. In particular, the treatment carried out using the protease-to-pectinase ratio 1:2 for 8 h (C2) was the most suitable, with a significant reduction (− 35% with respect to control) evident immediately after the treatment (t0). These findings agree with previous studies (Cerreti et al. 2016; Cerreti et al. 2017) where the combined application of proteases and pectinases, in free form (ratio 1:2), led to a relevant reduction of the immediate turbidity of pomegranate juice. These treatments could probably interfere with the mechanisms that lead to the formation of turbidity in fruit juices. The latter is due to the protein-polyphenol interactions that give rise to a hydrophobic core surrounded by the pectin shell. The synergistic action of the multi-enzymatic system results in an initial degradation of the pectic shell leading to the exposition of proteins (responsible for the formation of turbidity) to the action of protease (Grassin and Fauquembergue 1996; Pinelo et al. 2010).
The same synergistic effect was not evident either using the protease-to-pectinase ratio 1:2 (C1, probably for the short duration of the treatment) or using the protease-to-pectinase ratio 1:4 (C3 and C4). Perhaps, the latter could be due to the limited amount of immobilized BR, resulting in an excessive decrease in protease activity. Overall, the unbalanced ratio 1:4 (C3 and C4) had limited the efficacy in the reduction of immediate turbidity, which was not increased when the time treatment was extended (8 h instead of 4 h).
The effect of multi-enzymatic treatment in FBR on the potential turbidity (ΔNTU) of pomegranate juice, which accounts for the haze formation tendency, was also evaluated by the heat test (Fig. 7b). By comparing data right after the enzymatic treatment (t0), it appears that the potential turbidity of control was about 7 ΔNTU, whereas in treated samples, this parameter was always higher (compared with control). This increase could be ascribable to the initial destabilization of the colloidal systems (pectin-proteins) induced by the enzymatic treatment (Fig. 7b). During storage, the potential turbidity of control increased (+ 56% with respect to the initial value), whereas the treated samples showed an opposite trend with a significant decrease compared with control (about − 55% for C1, C3, and C4; about − 70% for C2) (Fig. 7b). These data confirm that, during storage at refrigerated conditions, pomegranate juices treated in FBR appeared less susceptible to the development of heat-induced turbidity compared with the control. These findings appear even more evident when the treatment was carried out in the FBR for 8 h, using the biocatalyst in the ratio 1:2 (C2).
It is widely recognized that the presence of a proteinaceous core surrounded by pectin molecules, in fruit juices, is the initial key factor in haze formation (Grassin and Fauquembergue 1996; Pinelo et al. 2010). The synergistic action of immobilized biocatalysts results from the initial breakdown of pectic envelope exerted by pectinases, thus exposing part of the underlying positively charged proteins. The latter became easily achievable to the protease, which exerts its proteolytic action on the haze active proteins, preventing the following binding with haze-active phenols.
Haze-Active Molecule
As reported by Siebert (2006), the addition of gelatin or tannic acid in fruit juice usually causes an increase in the level of turbidity, resulting from the presence of reactive phenols or proteins. Data in Fig. 8 show the evolution of haze-active (HA) phenols and proteins in juice untreated and treated in the FBR and then stored for 21 days at 4 °C. In untreated juice (control) and in C1 (Fig. 8a), a comparable increase in HA phenols was observed until the end of the storage period (about + 40%). In C3 and C4, the initial reactivity (t0) was slightly greater compared with that in the control and then, it reduced, with a decrease of about 37% with respect to control at the end of storage. Moreover, after 21 days, C2 showed a trend similar to that of C3 and C4 (Fig. 8a), with an analogous reduction of HA phenols (about − 43% in comparison with control). These findings prove that the enzymatically treated samples C2, C3, and C4 were characterized by the lowest tendency to react with gelatin compared with control, thus confirming the efficiency of the multi-enzymatic treatment in FBR in reducing the amount of HA phenols in pomegranate juice.
Haze-active (HA) phenols (a) and proteins (b) evaluated right after the enzymatic treatment (t0) and after 7, 14, and 21 days of storage at 4 °C in the dark. Samples were control (untreated juice), C1 (protease-to-pectinase ratio 1:2, treatment time in FBR 4 h), C2 (protease-to-pectinase ratio 1:2, treatment time in FBR 8 h), C3 (protease-to-pectinase ratio 1:4, treatment time in FBR 4 h), and C4 (protease-to-pectinase ratio 1:4, treatment time in FBR 8 h). Reported data are mean ± SD of triplicate analysis of juice samples. For each storage time, data with different letters differ significantly (Tukey’s test, p < 0.05) (t0 (A, B, C, D), 7 days (a, b, c, d), 14 days (α, β, γ, δ), and 21 days (W, X, Y, Z))
Concerning the evolution of HA proteins, data in Fig. 8b show that, right after the treatment in FBR (t0), a comparable amount of HA proteins was found in control, C3, and C4. It is noteworthy that the HA proteins in C1 and C2 (at t0) were reduced by 75% compared with control. In the latter, HA proteins doubled over the first week of storage (+ 50% compared with t0) and then, they remained almost constant. However, in all the treated samples, the reactivity to tannic acid was no longer detected throughout the conservation, starting from the first week. This trend (Fig. 8b) could be explained taking into account the low content of HA proteins in the initial juice (0.48 NTU), which probably were totally hydrolyzed by the immobilized bromelain, in particular when used in the highest dosage (C1 and C2).
These data are consistent with the findings of Cerreti et al. (2016), which proved the synergic effect between protease and pectinase (applied in free form), especially when bromelain was used in the enzymatic formulation, leading to a relevant change of the haze-forming behavior.
Effect of Multi-enzymatic Treatment on the Phenolics and Color of Pomegranate Juice During Storage
Phenolic Compound Indexes
The tone and intensity of the red color, in addition to the degree of clarity of the pomegranate juice, are important visual parameters that influence the quality of the product and partially affect the final consumer’s choice. Anthocyanins are the main compounds that account for the red shade of pomegranate juice, as well as its stability over time. The initial juice had a total anthocyanin content of 219 mg/L, whereas the monomeric anthocyanin index was 167 mg/L (Fig. 9). These values appeared consistent with those found by Tzulker et al. (2007) and Sepúlveda et al. (2010) in pomegranate juices from Israeli and Chilean varieties. Although the initial level (t0) of total anthocyanins was comparable between samples, only in control, a remarkable decrease was observed during storage. Among the samples enzymatically treated in the FBR, C2 was characterized by the highest content of total anthocyanins after 14 days and 21 days of storage. Similarly, also monomeric forms were comparable between samples at t0, with a relevant decrease in control during storage. The samples enzymatically treated in the FBR better preserved their phenolic pattern, showing a significantly higher content (with respect to control) for both indexes after 21 days of storage. A similar result was found after the enzymatic treatment conducted in batch mode, combining protease and pectinase in free form (Cerreti et al. 2016). By comparing multi-enzymatic treatments in FBR, no remarkable differences were observed among samples either for total or for monomeric anthocyanins (Fig. 9). Contrariwise, numerous studies reported that alternative clarifying treatments, based on the use of gelatin, PVPP, and bentonite, lead to a significant decrease in the anthocyanin levels in pomegranate juice (Vardin and Fenercioglu 2003; Turfan et al. 2012).
Total anthocyanin index (a) and monomeric anthocyanin index (b) evaluated right after the enzymatic treatment (t0) and after 7, 14, and 21 days of storage at 4 °C in the dark. Samples were control (untreated juice), C1 (protease-to-pectinase ratio 1:2, treatment time in FBR 4 h), C2 (protease-to-pectinase ratio 1:2, treatment time in FBR 8 h), C3 (protease-to-pectinase ratio 1:4, treatment time in FBR 4 h), and C4 (protease-to-pectinase ratio 1:4, treatment time in FBR 8 h). Reported data are mean ± SD of triplicate analysis of juice samples. For each storage time, data with different letters differ significantly (Tukey’s test, p < 0.05) (t0 (A, B, C, D), 7 days (a, b, c, d), 14 days (α, β, γ, δ), and 21 days (W, X, Y, Z))
Color Indexes
It is recognized that the changes in the phenolic pattern could strongly influence the chromatic characteristics of pomegranate juice (Alper and Acar 2004; Vardin and Fenercioglu 2003). The changes in color intensity (CI), tonality (T), and percentages of yellow (%Y), red (%R), and blue (%B) of pomegranate juice were monitored during storage (21 days at 4 °C). As reported in Table 3, all samples showed a similar initial CI (t0), ranging between 0.65 and 0.77, which was comparable with values indicated in literature (Sepúlveda et al. 2010). As expected, CI decreased during storage in all samples. This reduction was higher in control and in C4 (− 18% and − 15% compared with t0, respectively), whereas in the other samples, CI decreased to a lesser extent (− 1.5% in C1; about − 4% in C2 and C3 compared with t0).
Concerning T, no significant differences were observed between the samples at t0 (Table 3), whereas, during storage, this parameter increased in control, as also observed by Turfan et al. (2011, 2012). These data suggest that a faster evolution of color (from red to yellow/orange nuances) occurred in the control sample (indicating a detrimental effect in juice color), probably due to treater anthocyanin degradation discussed above. It is interesting to note that C2 was characterized by the highest CI and the lowest T values after 21 days of storage (Table 3). Regarding the color composition, data presented in Table 2 appeared in agreement with the results reported by other authors (Cerreti et al. 2016; Turfan et al. 2011; Turfan et al. 2012; Sepulveda et al. 2010), indicating that most of the color of pomegranate juice is attributable to the red component (about 63%), followed by the yellow (about 33%) and to a lesser extent by the blue component (about 4%). Furthermore, no appreciable changes were observed in the color composition throughout the storage. The only exception was revealed in control juice, with a slight increase in the percentage of yellow (+ 2%) and a corresponding reduction of the percentage of red (− 2%), proving that, after 21 days, the untreated juice was noticeably subjected to degradation phenomena involving the color (Table 3).
Conclusions
Two different food-grade enzymes (i.e., bromelain from pineapple stem (protease) and Pectinex® BE XXL (pectinase)) were successfully immobilized on A. Niger chitosan beads and applied for the first time for pomegranate juice clarification. The covalent immobilization procedure was optimized in order to maximize the catalytic activity of the protease- and pectinase-based biocatalysts and was demonstrated by means of SEM and DSC investigations. The multi-enzymatic system (containing protease, polygalacturonase, and pectin lyase activities) was applied in a fluidized bed reactor (FBR), varying the protease-to-pectinase ratio (1:2 or 1:4) and the treatment time (4 h or 8 h), for preventing the haze formation in pomegranate juice.
All the enzymatic treatments were useful for the reduction of the immediate and potential turbidity of the pomegranate juice (stored at 4 °C for 21 days), by the selective hydrolysis of molecules involved in haze formation. Among treatments, the one carried out using the protease-to-pectinase ratio 1:2, for 8 h, was the most suitable in terms of immediate and potential turbidity depletion. The samples enzymatically treated in the FBR better retained the native phenolic pattern, showing a higher content of both total and monomeric anthocyanins with respect to the untreated juice, for which a faster and detrimental evolution of color from red to yellow/orange nuances was revealed. At the end of storage, the pomegranate juice subjected to the best applied treatment in FBR (protease-to-pectinase ratio 1:2 for 8 h) was characterized by the highest color intensity and the lowest tonality. Considering these promising results, the multi-enzymatic system, immobilized on chitosan beads, could be applied for an efficient, selective, and long-lasting clarification in other fruit juices.
References
Albersheim, P. (1966). Pectin lyase from fungi. Methods in Enzymology, 8, 628–631.
Alper, N., & Acar, J. (2004). Removal of phenolic compounds in pomegranate juices using ultrafiltration and laccase-ultrafiltration combinations. Nahrung/Food, 48(3), 184–187.
Aviram, M., Rosenblat, M., Gaitini, D., Nitecki, S., Hoffman, A., Dornfeld, L., Volkova, N., Presser, D., Attias, J., Liker, H., & Hayek, T. (2004). Pomegranate juice consumption for 3 years by patients with carotid artery stenosis reduces common carotid intima-media thickness, blood pressure and LDL oxidation. Clinical Nutrition, 23(3), 423–433.
Baiano, A., Terracone, C., Gambacorta, G., & La Notte, E. (2009). Phenolic content and antioxidant activity of Primitivo wine: comparison among winemaking technologies. Journal of Food Science, 74, 258–267.
Bavaro, T., Cattaneo, G., Serra, I., Benucci, I., Pregnolato, M., & Terreni, M. (2016). Immobilization of neutral protease from Bacillus subtilis for regioselective hydrolysis of acetylated nucleosides: application to capecitabine synthesis. Molecules, 21, 1621.
Bell, C., & Hawthorne, S. (2008). Ellagic acid, pomegranate and prostate cancer: a minireview. Journal of Pharmacy and Pharmacology, 60(2), 139–144.
Benucci, I., Lombardelli, C., Cacciotti, I., Liburdi, K., Nanni, F., & Esti, M. (2016). Chitosan beads from microbial and animal sources as enzyme supports for wine application. Food Hydrocolloids, 61, 191–200.
Birò, E., Nemeth, A. S., Sisak, C., Feczko, T., & Gyenis, J. (2008). Preparation of chitosan particles suitable for enzyme immobilization. Journal of Biochemical and Biophysical Methods, 70(6), 1240–1246.
Bradford, M. M. (1976). A rapid and sensitive method for the quantification of microgram quantities of protein utilizing the principle of protein-dye binging. Analytical Biochemistry, 72(1-2), 248–254.
Busto, M. D., García-Tramontín, K. E., Ortega, N., & Perez-Mateos, M. (2006). Preparation and properties of an immobilized pectinlyase for the treatment of fruit juices. Bioresource Technology, 97(13), 1477–1483.
Cacciotti, I., Lombardelli, C., Benucci, I., & Esti, M. (2019). Clay/chitosan biocomposite systems as novel green carriers for covalent immobilization of food enzymes. Journal of Materials Research and Technology, In press, 8(4), 3644–3652.
Cao, L. (2005). Covalent enzyme immobilization (2 Eds). In Carrier-bound immobilized enzymes (pp. 169–316). KGaA: WILEY-VCH Verlag GmbH & Co.
Cappannella, E., Benucci, I., Lombardelli, C., Liburdi, K., Bavaro, T., & Esti, M. (2016). Immobilized lysozyme for the continuous lysis of lactic bacteria in wine: bench-scale fluidized-bed reactor study. Food Chemistry, 210, 49–55.
Cerreti, M., Liburdi, K., Benucci, I., & Esti, M. (2016). The effect of pectinase and protease treatment on turbidity and on haze active molecules in pomegranate juice. LWT-Food Science and Technology, 73, 326–333.
Cerreti, M., Liburdi, K., Benucci, I., Emiliani Spinelli, S., Lombardelli, C., & Esti, M. (2017). Optimization of pectinase and protease clarification treatment of pomegranate juice. LWT -Food Science and Technology, 82, 58–65.
Chen, H., Liu, L., Lv, S., Liu, X., Wang, M., Song, A., & Ji, X. (2010). Immobilization of Aspergillus niger xylanase on chitosan using dialdehyde starch as a coupling agent. Applied Biochemistry and Biotechnology, 162(1), 24–32.
Chuang, W. Y., Young, T. H., Yao, C. H., & Chiu, W. Y. (1999). Properties of the poly(vinyl alcohol)/chitosan blend and its effect on the culture of fibroblast in vitro. Biomaterials, 20(16), 1479–1487.
Deng, Z., Wang, F., Zhou, B., Li, J., Li, B., & Liang, H. (2019). Immobilization of pectinases into calcium alginate microspheres for fruit juice application. Food Hydrocolloids, 89, 691–699.
Di Cosimo, R., McAuliffe, J., Poulose, A. J., & Bohlman, G. (2013). Industrial use of immobilized enzymes. Chemical Society Reviews, 42, 6437–6474.
Di Stefano, R., & Guidoni, S. (1989). Metodi per lo studio dei polifenoli dei vini. L' Enotecnico, 25, 81–89.
Erkan, M. (2011). Pomegranate (Punica granatum L.). In E. M. Yahia (Ed.), Postharvest biology and technology of tropical and subtropical fruits (pp. 287–311). Oxford: Woodhead Publishing Limited.
Erkan-Koç, B., Türkyılmaz, M., Yemis¸, O., & Ozkan, M. (2015). Effects of various protein- and polysaccharide-based clarification agents on antioxidative compounds and colour of pomegranate juice. Food Chemistry, 184, 37–45.
Gardossi, L., Ebert, C., Ferrario, V., Braiuca, P., Basso, A., & Vaccari, L. (2008). Immobilizzazione di enzimi: ottimizzazione di biocatalizzatori industriali. La chimica e l’industria, 1, 94–100.
Glories, Y. (1984). La couleur des vins rouges. Mesure, origine et interprétation. Partie I. Connaissance de la Vigne et du Vin, 18, 195–217.
Grassin, C., & Fauquembergue, P. (1996). Application of pectinases in beverages. Progress in Biotechnology, 14, 453–462.
Gulec, H. A., Bagci, P. O., & Bagci, U. (2017). Clarification of apple juice using polymeric ultrafiltration membranes: a comparative evaluation of membrane fouling and juice quality. Food and Bioprocess Technology, 10(5), 875–885.
Hale, L. P., Greer, P. K., Trinh, C. T., & James, C. L. (2005). Proteinase activity and stability of natural bromelain preparations. International Immunopharmacology, 5, 783–793.
Jana, A., Halder, S. K., Ghosh, K., Paul, T., Vágvölgyi, C., Mondal, K. C., & Mohapatra, P. K. D. (2015). Tannase immobilization by chitin-alginate based adsorption-entrapment technique and its exploitation in fruit juice clarification. Food and Bioprocess Technology, 8(11), 2319–2329.
Jiang, D. S., Long, S. Y., Huang, J., Xiao, H. Y., & Zhou, J. Y. (2005). Immobilization of Pycnoporus sanguineus laccase on magnetic chitosan microspheres. Biochemical Engineering Journal, 25(1), 15–23.
Kalaycıoğlu, Z., & Erim, F. B. (2016). Total phenolic contents, antioxidant activities, and bioactive ingredients of juices from pomegranate cultivars worldwide. Food Chemistry, 221, 496–507.
Karangwa, E., Hayat, K., Rao, L., Nshimiyimana, D. S., Foh, M. B. K., Li, L., Ntwali, J., Raymond, L. V., Xia, S., & Zhang, X. (2012). Improving blended carrot-orange juice quality by the addition of cyclodextrins during enzymatic clarification. Food and Bioprocess Technology, 5(6), 2612–2617.
Kashyap, D. R., Chandra, S., Kaul, A., & Tewari, R. (2000). Production, purification and characterization of pectinase from a Bacillus sp. DT7. World Journal of Microbiology and Biotechnology, 16(3), 277–282.
Laudani, C. G., Habulin, M., Knez, Z., Della Porta, G., & Reverchon, E. (2007). Immobilized lipase-mediated long-chain fatty acid esterification in dense carbon dioxide: bench-scale packed-bed reactor study. The Journal of Supercritical Fluids, 41(1), 74–81.
Laemmli, U. K. (1970). Cleavage of structural proteins during the assembly of the head of bacteriophage T4. Nature, 227(5259), 680–685.
Miao, C., Zhonghong, L., Jianlong, W., Wupeng, G., Tianli, Y., Ronghua, L., et al. (2012). Food related applications of magnetic iron oxide nanoparticles: enzyme immobilization, protein purification, and food analysis. Trends in Food Science & Technology, 27, 47–56.
Miller, G. L. (1959). Use of dinitrosalicylic acid reagent for determination of reducing sugar. Analytical Chemistry, 31(3), 426–428.
Mirzaaghaei, M., Goli, S. A. H., & Fathi, M. (2016). Application of sepiolite in clarification of pomegranate juice: changes on quality characteristics during process. International Journal of Food Science & Technology, 51(7), 1666–1673.
O’Halloran, J., O’Sullivan, M., & Casey, E. (2019). Production of whey-derived DPP-IV inhibitory peptides using an enzymatic membrane reactor. Food and Bioprocess Technology, 12(5), 799–808.
Onsekizoglu, P. (2013). Production of high-quality clarified pomegranate juice concentrate by membrane processes. Journal of Membrane Science, 442, 264–271.
Petenzi, M., Bavaro, T., Cornaggia, C., Ubiali, D., Pregnolato, M., & Pasini, D. (2012). Synthesis, post-modification and characterization of linear polystyrene-based supports for interaction with immobilized biocatalysts. Polymer International, 61, 1611–1618.
Pinelo, M., Zeuner, B., & Meyer, A. S. (2010). Juice clarification by protease and pectinase treatments indicates new roles of pectins and proteins in cherry juice turbidity. Food and Bioproducts Processing, 88(2-3), 259–265.
Reddy, M. K., Gupta, S. K., Jacob, M. R., Khan, S. I., & Ferreira, D. (2007). Antioxidant, antimalarial and antimicrobial activities of tannin-rich fractions, ellagitannins and phenolic acids from Punica granatum L. Planta Medica, 53, 461–467.
Ribéreau-Gayon, P., Glories, Y., Maujean, A., & Dubourdieu, D. (2000). The chemistry of wine. Stabilization and treatments. In Handbook of enology volume 2 (2nd ed.). Chichester: John Wiley & Sons Ltd..
Sakurai, K., Maegawa, T., & Takahashi, T. (2000). Glass transition temperature of chitosan and miscibility of chitosan/poly (N-vinyl pyrrolidone) blends. Polymer, 41(19), 7051–7056.
Saponjić, S., Knežević-Jugović, Z. D., Bezbradica, D. I., Zuza, M. G., Saied, O. A., Bosković Vragolović, N., & Mijin, D. Z. (2010). Use of Candida rugosa lipase immobilized on sepabeads for the amylcaprylate synthesis: batch and fluidized bed reactor study. Electronic Journal of Biotechnology, 13, 1–15.
Sepúlveda, E., Sáenz, C., Peña, A., Robert, P., Bartolomé, B., & Gómez-Cordovés, C. (2010). Influence of the genotype on the anthocyanin composition, antioxidant capacity and color of Chilean pomegranate (Punica granatum L.) juices. Chilean Journal of Agricultural Research, 70, 50–57.
Siebert, K. J. (2006). Haze formation in beverages. LWT - Food Science and Technology, 39(9), 987–994.
Siebert, K. J., Carrasco, A., & Lynn, P. Y. (1996). Formation of protein-polyphenol haze in beverages. Journal of Agricultural and Food Chemistry, 44(8), 1997–2005.
Sorrivas, V., Genovese, D. B., & Lonzano, J. E. (2006). Effect of pectinolytic and amylolitic enzymes on apple juice turbidity. Journal of Food Processing and Preservation, 36, 118–133.
Tischer, W., & Wedekind, F. (1999). Immobilised enzymes: methods and applications. In W. D. Fessner et al. (Eds.), Biocatalysis-from discovery to application. Topics in current chemistry (pp. 95–126). Berlin: Springer.
Turfan, O., Turkyilmaz, M., Yemis, O., & Ozkan, M. (2011). Anthocyanin and colour changes during processing of pomegranate (Punica granatum L. cv. Hicaznar) juice from sacs and whole fruit. Food Chemistry, 129(4), 1644–1651.
Turfan, O., Turkyilmaz, M., Yemis, O., & Ozkan, M. (2012). Effects of clarification and storage on anthocyanins and color of pomegranate juice concentrates. Journal of Food Quality, 35(4), 272–282.
Tzulker, R., Glazer, I., Bar-Ilan, I., Holland, D., Aviram, M., & Amin, R. (2007). Antioxidant activity, polyphenol content and related compounds in different fruit juices and homogenates prepared from 29 different pomegranate accessions. Journal of Agriculture and Food Chemestry, 55(23), 9559–9570.
Vardin, H., & Fenercioglu, H. (2003). Study on the development of pomegranate juice processing technology: clarification of pomegranate juice. Food/Nahrung, 47(5), 300–303.
Vincenzi, S., Marangon, M., Tolin, S., & Curioni, A. (2011). Protein evolution during the early stages of white winemaking and its relations with wine stability. Australian Journal of Grape and Wine Research, 17(1), 20–27.
Zeng, M., Fang, Z., & Xu, C. (2004). Effect of compatibility on the structure of the microporous membrane prepared by selective dissolution of chitosan/synthetic polymer blend membrane. Journal of Membrane Science, 230(1-2), 175–181.
Zhao, L., Wang, Y., Qiu, D., & Liao, X. (2014). Effect of ultrafiltration combined with high-pressure processing on safety and quality features of fresh apple juice. Food and Bioprocess Technology, 7(11), 3246–3258.
Zhou, G. X., Chen, G. Y., & Yan, B. B. (2014). Biodiesel production in a magnetically stabilized, fluidized bed reactor with an immobilized lipase in magnetic chitosan microspheres. Biotechnology Letters, 36(1), 63–68.
Funding
This work was financially supported by the BioEnBi project “Biotecnologie enzimatiche innovative per processi di chiarifica sostenibili nel settore birrario” (Grant 85-2017-15362), funded by Lazio Innova Spa, Lazio Region (Italy), in the context of Progetti Gruppi di Ricerca, Lazio Innova 2018–2020.
Author information
Authors and Affiliations
Corresponding author
Additional information
Publisher’s Note
Springer Nature remains neutral with regard to jurisdictional claims in published maps and institutional affiliations.
Rights and permissions
About this article
Cite this article
Benucci, I., Mazzocchi, C., Lombardelli, C. et al. Multi-enzymatic Systems Immobilized on Chitosan Beads for Pomegranate Juice Treatment in Fluidized Bed Reactor: Effect on Haze-Active Molecules and Chromatic Properties. Food Bioprocess Technol 12, 1559–1572 (2019). https://doi.org/10.1007/s11947-019-02315-w
Received:
Accepted:
Published:
Issue Date:
DOI: https://doi.org/10.1007/s11947-019-02315-w