Abstract
The hooded pitcher plant, Sarracenia minor, is a carnivorous facultative wetland species native to the southeastern USA and is listed as threatened by the state of Florida. Pitchers of S. minor possess white, semitranslucent spots (areoles), which have been hypothesized to aid in the capture of prey (= visual lures) by increasing the amount of light entering the back of the hood, which persuades insects to enter and fall into the base of the pitcher. In this study, the role of the areoles in prey capture abundance and diversity were experimentally investigated under variable lighting conditions in situ. Plants in two populations experiencing different light intensities, which varied in the amount of canopy cover and incident light reaching the plants, were experimentally manipulated by coloring varying percentages of areoles, ranging from 0 to 100 % (in increments of 25 %), with indelible ink. After 2 months, pitchers were collected and dissected to determine the number and identity of prey captured (approximately 18,000 prey were sampled). Although total prey abundance was approximately five times higher at McGirt’s Creek (sunny site) compared to UNF (shaded site), the effect of areoles on prey capture rates and biodiversity was site dependent. Reducing the number of visual lures of plants at the sunny site produced a significant decrease in the number of prey captured, but prey biodiversity (community composition) was unaffected. However, total prey capture was unaffected at the shaded site, while prey biodiversity was negatively correlated with the percent of areoles colored. Results from the current study suggest that areoles conditionally act as visual lures, but their overall importance is dependent on local environmental variables—especially canopy cover and the amount of incident sunlight reaching the plants.
Similar content being viewed by others
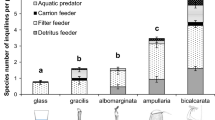
Avoid common mistakes on your manuscript.
Introduction
Carnivorous plants have long been of interest to the scientific community because of their ability to thrive in environments with low levels of soil nitrogen. These predatory plants thrive in nitrogen-poor ecosystems because they have evolved structures that enable them to attract, capture, and digest prey. Carnivorous plants use a combination of mechanisms to maximize their prey capture (Gibson 1991; Newell and Nastase 1998; Ellison and Gotelli 2001; Ellison and Farnsworth 2005; Farnsworth and Ellison 2008). Some of these mechanisms such as visual lures, which reduce surface area available to capture sunlight, create a need for an increased uptake of carbon and nitrogen (Thoren et al. 2003). Nitrogen deficiency can be ameliorated by carnivory; evolutionary ecologists have shown great interest in determining the potential costs and benefits of carnivory in nutrient-poor environments (Newell and Nastase 1998; Ellison and Gotelli 2001; Brewer 2003; Ellison and Farnsworth 2005). While it has been suggested that the prey capture efficiency of carnivorous plants can be low, especially if light or water are limited, it is unclear whether the benefits of increased nutrients from captured prey outweigh the costs of producing nectar and other adaptations that attract prey and lower photosynthetic rates of the leaves (Cresswell 1991, 1992; Heard 1998).
In particular, pitcher plants have several adaptations that aid in the attraction and capture of prey: pitcher morphological traits such as the size and shape of the leaves (Cresswell 1992; Ellison and Gotelli 2001; Ellison and Farnsworth 2005; Barthlott et al. 2007; Farnsworth and Ellison 2008), extrafloral nectaries (Heard 1998; Joel 1988; Newell and Nastase 1998), and colored visual lures and ultraviolet patterns (Joel et al. 1985; Moran 1996; Schaefer and Ruxton 2008). Joel (1988) examined the relationship between extrafloral nectaries and capture of ants and suggested that ants and carnivorous plants have a limited mutualistic relationship. Carnivorous plants attract ants with a source of nutrition then capture a portion of the members of the ant community as a source of nutrients. A combination of extrafloral nectaries at the mouth of the pitcher, visual lures of red and white pigmentation to mimic flowers, and pitcher size have been shown to attract and capture the most prey (Cresswell 1991; Newell and Nastase 1998; Green and Horner 2007; Bhattarai and Horner 2009).
Prey capture is positively correlated with the size or capture area of pitchers of Sarracenia purpurea (L.) (Creswell 1992; Heard 1998) and S. alata (Alph. Wood; Green and Horner 2007; Bhattarai and Horner 2009). Red coloration has also been shown to increase the capture rate of flying insects in pitchers of the genus Nepenthes (Schaefer and Ruxton 2008). However, while Newell and Nastase (1998) found that prey such as ants were more likely to visit pitchers of S. purpurea; with more red venation than those that had minimal red venation, Bennett and Ellison (2009) demonstrated that sugar (i.e., nectar) not color was attractive to prey. Biesmeijer et al. (2005) noted that hymenopterans had a preference for the dark centers, stripes, and peripheral dots of pitcher plants indicating nectar sources, while Schaefer and Ruxton (2008) suggested that the red coloration may act as a signaling pathway to lead prey to the nectaries and the trap. A comprehensive study by Joel et al. (1985) found that ultraviolet patterns of carnivorous plants such as S. flava (L.) likely attract prey to nectaries. Nectar-producing areas showed significant ultraviolet absorption that visibly contrasted with the surrounding nectar-free areas of the pitcher or the nectar itself (composed primarily of carbohydrates which do not absorb UV radiation near the visible part of the spectrum).
Additionally, among the families of carnivorous plants, Nepenthaceae (Asia) and Sarraceniaceae (Americas) have purportedly developed some distinct lures to aid in the capture of prey (Ellison and Gotelli 2001; Schnell 2002; Ellison and Farnsworth 2005; Barthlott et al. 2007; Farnsworth and Ellison 2008; Moran et al. 2012). For instance, within the Nepenthaceae species such as Nepenthes aristolochioides (Jebb and Cheek) and N. klossii (Ridl.) have pitchers with translucent domes (Moran et al. 2012). Similarly, the pitchers of several species of Sarraceniaceae including Darlingtonia californica [Torr.], Sarracenia minor [Walter], S. leucophylla [Raf.], and S. psittacina [Michx.]) possess clear apigmented spots (called fenestrations) and/or areoles, which are similar to fenestrations except they contain a white pigment (Schnell 2002; Thomas 2002; McPherson 2006). It has been hypothesized that these pitcher characteristics (i.e., translucent domes, fenestrations, and areoles) attract prey into the pitcher (Thomas 2002; Barthlott et al. 2007; McPherson 2006) although this role has received only limited testing (e.g., Moran et al. 2012). Ants that have been attracted to the pitcher by extrafloral nectaries are the primary prey of pitcher plants, but depending on the characteristics of the individual pitcher species, pitcher plants may capture a wide variety of other prey (Fish 1976; Cresswell 1991, 1992; Heard 1998; Meyer et al. 2001; Bhattarai and Horner 2009).
The hooded pitcher plant, Sarracenia minor, ranges from the southern tip of North Carolina down the eastern coast through central Florida in shaded pine flatwoods (Sheridan 1996; Schnell 2002; Thomas 2002). Sarracenia minor plants exist as clusters of pitchers, which originate from common below-ground rhizomes, thus individual pitcher clusters (ramets) may be part of a larger single genetic individual (genet) potentially consisting of multiple pitcher clusters. Flowering of S. minor occurs at the same time as the growth of new pitchers in the late spring to early summer. Erect pitchers average 25–30 cm in length with a prominent wing (or keel) and range from green to red in coloration. The lid of the pitcher forms a hood (or operculum) that completely covers the mouth of the pitcher, which creates darkness inside the pitcher; the hood has a small gap which leads to the opening of the pitcher. Covering the back and the hood of the pitcher are white pigmented spots (areoles) that lack chlorophyll and allow light to filter into the dark pitcher (Fig. 1). A lower photosynthetic rate may be seen in pitchers with more areoles or fenestrations because there is less available area for photosynthesis (Ellison and Gotelli 2001; Brewer 2003). It has been suggested that areoles and fenestrations act as “visual lures” to entice prey further into the pitcher, but this function has only recently been tested experimentally (Schaefer and Ruxton 2008; Bennett and Ellison 2009; Moran et al. 2012). The primary prey of S. minor are ants (Fish 1976; Newell and Nastase 1998; Meyer et al. 2001; Moon et al. 2010). Ants are attracted to the pitcher by the extrafloral nectaries and apparently continue inside the pitcher because of the attractive presence of areoles (Fish 1976; Schnell 1976; Slack 1979). Other insects have also been found in the pitchers, but in much lower abundance (Fish 1976; Meyer et al. 2001; Beaulac et al. 2002). It has been hypothesized that these insects are attracted to the pitchers by “visual lures” that direct the insects to the location of the extrafloral nectaries. Pitchers of S. minor may also suffer high rates of herbivory by the pitcher plant-mining noctuid, Exyra semicrocea (Gueneé), and ants have been shown to reduce damage caused by this moth (Moon et al. 2008, 2010).
The objectives of this study were to: (1) determine whether areoles play a role in attracting prey to the individual pitcher by manipulating the number of areoles visible and whether the number of areoles visible affects the rate at which prey are captured, and (2) examine the relationship between prey diversity and the number of areoles. Additionally, because S minor is a facultative wetland species, it inhabits ecosystems that range from open sunny bogs and seepage savannas to shaded mixed wetland-pine ecosystems that have high levels of canopy cover. As a result, the relative strength of these effects were compared under variable lighting conditions in situ using native populations of the hooded pitcher plant from an open sunny site and a densely wooded and shaded site.
Materials and methods
Study system
Although plants were initially identified in March 2009, this study was conducted from July to September, when new pitchers are developing and the growth of pitchers is greatest (Meyer et al. 2001; Beaulac et al. 2002; Moon et al. 2008). Two sites with large populations of S. minor were used for this study: (1) shaded site in the wet flatwoods within the Sawmill Slough Nature Preserve on the campus of University of North Florida (UNF), Jacksonville, FL (30°15′59″, −81°−31′6″), which contains heavy canopy cover (Meyer et al. 2001; Beaulac et al. 2002), and (2) sunny site located in an open powerline corridor in the McGirt’s Creek Preserve, Jacksonville, FL (30°14′21″, −81°−48′23″).
Experimental methods
A total of 120 individual pitcher plant ramets of S. minor were haphazardly selected for the study (60 from each site). Only pitcher plant ramets that possessed newly opened pitchers were used in the study, and, of those, only pitchers that were newly opened and displayed no signs of herbivory were used for sampling. Within each cluster of pitchers (ramet), each newly opened pitcher was marked with a wire tag. The following measurements were taken for each newly opened pitcher in the ramet: (1) pitcher height, measured with a meter stick from the ground to the highest point of each pitcher (to the nearest 0.1 cm); (2) width of each pitcher, measured at the widest point across the back of the pitcher with digital calipers (to the nearest 0.1 mm); (3) width of the keel of the pitcher, at its widest point, measured with digital calipers (to the nearest 0.1 mm). In addition, the total number of pitchers per ramet was counted. The number and size of areoles on each pitcher was also recorded (see below).
Although pitchers of S. minor typically possess red pigmentation, its role in attracting prey is poorly understood. For instance, the vision of most insects extends farther into the ultraviolet range of the electromagnetic spectrum (compared to animals such as birds or mammals) at the expense of decreased acuity in the red wavelengths of the spectrum. However, red pigmentation may provide sufficient contrast to the generally green background of many plant structures as to be attractive to insects. Lastly, it has been suggested that red pigmentation may be non-adaptive and it is a response of the plant to stress because red anthocyanins tend to increase under N and P deficiencies which typically characterize the habitat of carnivorous plants (reviewed by Schaefer and Ruxton 2008). Pitchers of S. minor were manipulated to determine the effect of areoles on the capture rates of the pitchers as well as the diversity of prey captures because it has been shown that red pigmentation has a strong effect on attracting prey to pitcher plants, especially dipterans (Newell and Nastase 1998; Schaefer and Ruxton 2008). The areoles on the top and the back of the pitcher hood were filled in with a black Sharpie® marker to darken the areoles at least as much as the surrounding pigmented tissue. Black, rather than a colored Sharpie®, was chosen to avoid possibly attracting more prey; all individual pitchers of each plant cluster (ramet) were filled in, and, at the end of the study, a single randomly selected pitcher was selected and had its pitcher contents sampled. Neither uncolored areoles nor those filled in with a Sharpie® had different ultraviolet patterns than the rest of the pitcher (unpublished data). Attempts were made to color in the areoles with a green Sharpie® marker, but it was observed that very little light was blocked by the green pigment.
In July 2009, ten pitcher plant ramets were chosen at random from the 60 marked at each site which were used for the levels of manipulation in the study. Depending upon the treatment, plant areoles were colored in at intervals of 25, 50, 75, or 100 %. Although the control group of plants had none of their areoles colored (0 %), they were rubbed with the cap of the marker to simulate the disturbance caused by coloring in the areoles on treated plants. The newly opened pitchers of the second set of ten ramets had 25 % of the total number of areoles of each pitcher colored in with a black Sharpie® marker. This method was repeated again to color in 50, 75, and 100 % of the total number of areoles of each set of ten pitcher plant ramets constituting a treatment group. The final set of ten pitcher plant ramets (negative control) had their pitchers marked with the Sharpie® in areas around the areoles to examine the effect of the pitchers being colored and to eliminate the possibility that any potential pattern was caused by the location of the marker on the pitcher, rather than coloring areoles in particular.
Once all pitcher treatments were established, plants were monitored for approximately 2 months (July 2009–September 2009). During this period, pitchers were checked weekly for the growth of existing areoles or the development of new ones; these new areoles were colored in according to the plant’s treatment group. Pitchers were also examined for any signs of herbivory by the presence of any discoloration of the pitcher [herbivory by the pitcher plant-mining moth E. semicrocea (Noctuidae)] and by the presence of any chewed or damaged portions of the pitcher [herbivory by other insects: orthopterans or hymenopterans, particularly members of the grass carrying wasp Isodontia mexicana (de Saussure) (Sphecidae)]. Any pitcher that had sustained damage was removed because herbivory could affect or preclude prey capture. Before it was removed, the final height, hood width, and keel width were measured in the field and the date of collection was recorded. After approximately 2 months, the remaining pitchers were measured for their final height, hood width, and keel width in the field. Each pitcher was cut off at the base of the plant, bagged in a zip-top bag, and kept cool to be transported back to the laboratory. Ten of the 60 pitchers collected from each site were randomly selected, and the average area of their areoles, which fell along the midline of the pitcher through the region of the hood, was measured using a digital leaf area meter (Model CID-201; CID, Inc., Vancouver, WA, USA). Each pitcher was cut open along the keel with a razor blade, and the contents of the pitcher were emptied into sterile plastic tubes containing 70 % ethanol. Some of the contents of the pitchers had to be loosened from the base of the pitcher using forceps or a fine-bristled brush and then flushed with 70 % ethanol. The contents of each pitcher were examined under a dissecting microscope (6.3×–60× mag.), and the captured prey were counted and identified to the family level when possible. Prey organisms were counted by head capsules, and any thorax or abdomen lacking a head was discarded and not included in the final count. Along with the prey, pitcher plant commensals and herbivores were counted and identified to family, but their numbers were excluded from the total number of prey captured for each pitcher because they were not prey per se. Specimens excluded from the prey count included: larvae and any adults of E. semicrocea that were observed to be inhabiting the pitcher, Sarcophagidae larvae inhabiting the prey remains in the base of the pitcher, any spiders (Araneae) observed to be inhabiting the upper portions of the pitcher, all mites considered to be commensals of the pitcher (Histiostomatidae and Phytoseiidae), and any prey (primarily Gryllidae) observed to have been placed in a grass nest within the pitcher by I. mexicana. Overall mean family-level species biodiversity, measured as total number of families, as well as Shannon and Simpson indices for arthropod families were compared using t tests. Diversity of prey captured was determined using family richness, Simpson’s diversity index and the Shannon’s index. Simpson’s index of diversity (D s) was calculated by D s = 1 − Σn i (n i − 1)/(N(N − 1), where n i is the number of individuals in each family, and N is the total number of individuals in the sample. Shannon’s diversity index (H′) was calculated by H′ = −Σ[(p i )(ln p i )], where p i is the proportional abundance of individuals in each family.
The intensity of light reaching the pitchers was determined using a handheld light meter, and the average estimation of overstory density (= canopy cover) was determined using a densitometer by randomly choosing 30 of the 60 pitcher plant specimens at each site and recording values level with the top of each pitcher. These measurements were taken once at each site, around noon, during similar weather conditions.
Statistical analyses
Site characteristics and some plant parameters, including light intensity, canopy cover, number of areoles per pitcher, and prey capture rates, between the shaded site (UNF) and sunny site (McGirt’s Creek) were compared using either z or t tests (depending on sample size). For all statistical tests, recently opened pitchers, which had not yet caught any prey, were not included in the analyses (these did not differ between the groups). Linear regression and correlation analyses were used to assess the relationship between number of areoles with pitcher height and width for both sites. Although prey capture rates were log-transformed to meet the normality assumption of ANOVA, results are presented non-transformed for clarity. Lastly, to examine the relationship between prey capture and biodiversity and areoles, mean abundance and family-level species richness and the percent of areoles filled in were assessed using linear regression and correlation analyses for each site. All statistical tests were performed using Systat 11 (Systat Software, Inc., 1735 Technology Drive, Suite 430, San Jose, CA 95110, USA).
Results
Prey capture rates of pitchers that had 0 % of their areoles filled in (areoles were rubbed with the cap of the marker) did not differ significantly from those that had the area surrounding their areoles colored at either site (P = .826; df = 18 at McGirt’s Creek and P = .366; df = 18 at UNF) which indicates that the coloring technique employed in the study did not confound treatment effects. As expected, the two sites had highly significant differences in light intensity (z = 64.880; P < .001; df = 58) and average estimation of overstory density (z = 30.349; P < .001; df = 58) between the two sites. The open sunny site (McGirt’s Creek) had a mean (±SD) of 21 ± 5 % canopy cover and an average light intensity of 60,200 ± 660 lum/m2 compared to the shaded site (UNF) which had an average 80 ± 9 % canopy cover and an average light intensity of 10,030 ± 410 lum/m2. Moreover, pitchers at McGirt’s Creek captured 6–7 times more prey than those from UNF which resulted in a highly significant difference between the two sites (t = 34.452; P < .001; df = 118; Fig. 2). However, the total number of areoles per pitcher was not significantly different between the two sites (t = 1.859; P = .068; df = 118); plants at McGirt’s Creek had an average of 80.6 ± 30 areoles while those at UNF had an average 71.4 ± 23. Additionally, the mean surface area of the areoles did not differ significantly between the two sites (t = 1.972; P = .064; df = 18); areolar area averaged 3.0 ± 1.9 and 2.0 ± 1.9 m2 at McGirt’s Creek and UNF, respectively. At both sites, the total number of areoles was positively correlated with the height and width of the pitcher, although strength of the relationship varied substantially (i.e., 10–21 % of the variation in number of areoles explained by pitcher height at McGirt’s Creek and UNF, respectively, while 12–38 % of the variation in number of areoles was explained by pitcher width at McGirt’s Creek and UNF, respectively; Fig. 3).
When only prey were assessed, nine arthropod orders (Hymenoptera, Diptera, Coleoptera, Collembola, Orthoptera, Hemiptera, Araneae, Thysanoptera, and Lepidoptera) were represented among the captured prey at the UNF and McGirt’s Creek sites. Of the total of 2976 prey captured at UNF (27 families), hymenopterans (just ants) were most common (1811; ~61 %) followed by dipterans (853; ~29 %). Of the 15,088 prey captured at McGirt’s Creek (41 families), hymenopterans (just ants) were most common (12,823; ~85 %) followed by dipterans (1874; ~12 %). Overall prey diversity indices were significantly lower for McGirt’s Creek compared to UNF (for Simpson’s index McGirt’s Creek D s = 0.2721, UNF D s = 0.5892; t = 30.54, P < .001; df = 66 and for Shannon’s index McGirt’s Creek H′ = 0.8805, UNF H′ = 1.4973; t = 16.26, P < .001; df = 66); for a complete list of pitcher contents see Supplemental Tables 1 and 2).
With the exception of pitcher height at McGirt’s Creek, pitcher size (height and width) were positively correlated with the total number of prey captured, although width was a better predictor of prey abundance (Figs. 3, 4). At the sunny site (McGirt’s Creek), mean prey abundance declined significantly (r 2 = .2415; P < .01; n = 5) by approximately 40 % when 100 % of areoles were filled compared to control pitchers that had no areoles filled in; however, no effect on prey abundance was detected for the shaded site UNF (r 2 = .0967; P = .584; n = 5; Fig. 5). Conversely, prey diversity was unaffected (r 2 = .1828; P = .719; n = 5) at the sunny site (McGirt’s Creek) while the shaded site (UNF) exhibited a significant negative correlation (r 2 = .6175; P < .01; n = 5) between the number of families captured and the percentage of areoles filled; declining approximately 30 % when areoles were completely filled in compared to control plants (Fig. 6).
Discussion
It has been suggested that pitcher plants attract prey by a variety of adaptations (pigmentation, extrafloral nectar production, ultraviolet coloration, and/or visual lures; Heard 1998; Joel 1988; Newell and Nastase 1998; Joel et al. 1985; Moran 1996; Schaefer and Ruxton 2008). However, only recently have studies (e.g., Schaefer and Ruxton 2008; Bennett and Ellison 2009; Moran et al. 2012) experimentally tested the hypothesis that fenestrations/areoles act as visual lures and whether or not areoles influence the abundance, diversity, and efficiency of prey captured. Overall, pitchers at the McGirt’s Creek site caught significantly more prey, but a lower diversity of prey than the pitchers at the UNF site. At UNF though, as the percentage of visible areoles decreased, so did the number of prey families. One factor that could affect the diversity of prey captured by the pitchers is the overall diversity of arthropods available at the site which was not assessed in this study. Not surprisingly, at both sites and across all treatment groups, prey species mostly consisted of hymenopterans (primarily ants) and dipterans. Under normal conditions, ants make up the majority of prey captured by pitchers of S. minor; it has been suggested that these plants are ant specialists (Fish 1976; Newell and Nastase 1998; Meyer et al. 2001). At both sites, the number of areoles visible did not affect the pitchers’ ability to capture ants. However, it has been shown that some insects, like dipterans, are visually attracted to pitchers by the contrasting red coloration (dotted and/or striped pattern) of the pitcher (Moran 1996; Schaefer and Ruxton 2008).
At the bright sunny site (McGirt’s Creek), prey capture rate was significantly influenced by the total number of areoles that were visible. However, no significant effect of the number of areoles on prey abundance was observed at the shaded site (UNF). This is consistent with both the life history of the pitcher plant, which often thrives in open sunny bogs, and casual observations of both sites; the open sunny site (McGirt’s Creek) typically has many more active insects (A.M.R. personal observation). Thus, lower number of prey being captured by the plants at the shaded UNF site may simply reflect lower resident prey species abundance at this site and the smaller sample sizes are likely to reduce the detectable effect of areoles on prey capture rates. While prey capture rates were significantly higher for McGirt’s Creek compared to UNF, the reverse was true for arthropod diversity; both measures of prey family species richness were significantly greater for UNF. Results from the current study suggest that areoles may be of less importance to prey biodiversity in sunny habitats where their effect on internal light intensity is probably minimal compared to their effects on internal pitcher illumination in heavily shaded sites. Higher environmental heterogeneity at UNF (combination of trees, shrubs, and grasses) also likely influenced prey diversity; more environmental heterogeneity may produce an increase in the associated insect community at UNF. McGirt’s Creek has a more homogenous environment (primarily grasses and wildflowers with few shrubs and no trees), and diversity of insects may be more uniform throughout the environment and thus less likely to decline as the percentage of visible areoles decreased. These results suggest that areoles do increase light inside the pitcher at shaded sites which attracts a more diverse collection of prey with a broader range of visual acuity.
In conclusion, this study found that areoles on the hood and back of pitchers of S. minor do play a role in the attraction and capture of prey, but the effect is dependent upon ambient light levels. Pitcher plants have evolved in high-light and low-nutrient environments (Ellison and Gotelli 2001; Schnell 2002; Brewer 2003). Since the pitchers of S. minor have a hood covering the tube of the pitcher, the only way light can enter into the pitcher is through the areoles. These illuminated areoles and fenestrations have been suggested to mimic, to prey, a path to exit the pitcher once they are done feeding on the extrafloral nectaries (Schnell 2002). Sites with more canopy cover will result in more shading and less light entering the pitchers through the areoles, thus reducing overall illumination of the inside of the pitcher. As areoles were filled in, even less light was allowed to enter into the pitcher causing a decrease in the illumination of the inside of the pitcher. This reduction in light inside the pitcher in heavily shaded habitats may have affected the diversity of prey being captured by the pitcher because fewer prey may have been misled into flying into areoles in the interior of the pitcher hood (after feeding on the extrafloral nectaries) and falling into the base of the pitcher in low-light conditions. Instead, the prey may have simply exited through the more illuminated opening between the mouth and hood of the pitcher. It is also possible that certain species or groups of insects may be differentially attracted by visual lures versus chemical lures produced by pitcher plants.
There is little doubt that the areoles and fenestrations have evolved in conjunction with pitcher morphology and other visual lures to help the pitchers capture more prey (Gibson 1991; Newell and Nastase 1998; Ellison and Gotelli 2001; Ellison and Farnsworth 2005; Farnsworth and Ellison 2008) and that they do aid in attracting prey in pitcher plants. However, their importance is likely dependent on local environmental conditions such as canopy cover and the intensity of light reaching the pitchers. Unfortunately, this study did not measure prey diversity in terms of biomass which is important for fully understanding nutrient dynamics in this species. Converting prey contents (i.e., prey counts) to biomass is difficult because the relatively long period of the study meant that prey were often disarticulated and/or partially decomposed; as a result, the only reliable way to identify specimens was by “head capsules” which tend to breakdown more slowly than other structures owing to thick layers of sclerotin; additionally, this study may be biased to find “hard-bodied” arthropods since they decompose more slowly. However, it might be possible in future studies to concurrently sample (and measure the mass) of local prey to estimate biodiversity in terms of biomass. Moreover, concurrent site sampling of arthropod diversity at each site is recommended so that estimates of the potential prey biodiversity available that is being captured can be determined and to what degree prey diversity reflects the local biodiversity of the site can be estimated.
References
Barthlott W, Porembski S, Seine R, Theisen I (2007) Sarraceniaceae: American pitcher plant family. The Curious World of Carnivorous Plants: a comprehensive guide to their biology and cultivation. Timber Press, Oregon
Beaulac D, Walguarnery J, Meyer R (2002) Baseline study of the hooded pitcher plant (Sarracenia minor): its utility as an indicator species of freshwater wetland habitats. Osprey J Ideas Inq 2:17–24
Bennett KF, Ellison AM (2009) Nectar, not colour, may lure insects to their death. Biol Lett 5:469–472
Bhattarai GP, Horner JD (2009) The importance of pitcher size in prey capture in the carnivorous plant, Sarracenia alata Wood (Sarraceniaceae). Am Midl Nat 161:264–272
Biesmeijer JC, Giurfa M, Koedam D, Potts SG, Joel DM, Dafni A (2005) Convergent evolution: floral guides, stingless bee nest entrances, and insectivorous pitchers. Naturwissenschaften 92:444–450
Brewer JS (2003) Why don’t carnivorous pitcher plants compete with non-carnivorous plants for nutrients? Ecol 84:451–462
Cresswell JE (1991) Capture rates and composition of insect prey of the pitcher plant Sarracenia purpurea. Am Midl Nat 125:1–9
Creswell JE (1992) The morphological correlates of prey capture and resource parasitism in pitcher of the carnivorous plant Sarracenia purpurea. Am Midl Nat 129:35–41
Ellison AM, Farnsworth EJ (2005) The cost of carnivory for Darlingtonia californica (Sarraceniaceae): evidence from relationships among leaf traits. Am J Bot 92:1085–1093
Ellison AM, Gotelli NJ (2001) Evolutionary ecology of carnivorous plants. Trends Ecol Evol 16:623–629
Farnsworth EJ, Ellison AM (2008) Prey availability directly affects physiology, growth, nutrient allocation and scaling relationships among leaf traits in 10 carnivorous plant species. J Ecol 96:213–221
Fish D (1976) Insect-plant relationships of the insectivorous pitcher plant Sarracenia minor. Fla Entomol 59:199–203
Folkerts D (1999) Pitcher plant wetlands of the Southeastern United States. In: Batzer DP, Rader RB, Wissinger SA (eds) Invertebrates in freshwater wetlands of North America: ecology and management. Wiley, New York
Gibson TC (1991) Differential escape of insects from carnivorous plant traps. Am Midl Nat 125:55–62
Green ML, Horner JD (2007) The relationship between prey capture and characteristics of the carnivorous pitcher plant, Sarracenia alata wood. Am Midl Nat 158:424–443
Heard SB (1998) Capture rates of invertebrate prey by the pitcher plant, Sarracenia purpurea L. Am Midl Nat 139:79–89
Joel DM (1988) Mimicry and mutualism in carnivorous pitcher plants (Sarraceniaceae, Nepenthaceae, Cephalotaceae, Bromeliaceae). Biol J Linn Soc 35:185–197
Joel DM, Juniper BE, Dafni A (1985) Ultraviolet patterns in the traps of carnivorous plants. New Phytol 101:585–593
McPherson S (2006) Sarracenia pitcher plants of the Americas. McDonald & Woodward Publishing, Virginia
Meyer R, Walguarnery J, Beaulac D, Brown T, Hughes K (2001) Morphological differences among two populations of the hooded pitcher plant, Sarracenia minor, and its usefulness as an indicator species for bog and seepage savanna communities. Osprey J Ideas Inq 1:9–12
Moon DC, Rossi A, Stokes K, Moon J (2008) Effects of the pitcher plant mining moth Exyra semicrocea on the hooded pitcher plant Sarracenia minor. Am Midl Nat 159:321–326
Moon DC, Rossi A, Depaz J, McKelvey L, Elias S, Wheeler E, Moon J (2010) Ants provide nutritional and defensive benefits to the carnivorous plant Sarracenia minor. Oecol 164:185–192
Moran JA (1996) Pitcher dimorphism, prey composition and the mechanisms of prey attraction in the pitcher plant Nepenthes rafflesiana in Borneo. J Ecol 84:515–525
Moran JA, Clarke C, Gowen BE (2012) The use of light in prey capture by the tropical pitcher plant Nepenthes aristolochioides. Plant Sign Behav 7:957–960
Newell SJ, Nastase AJ (1998) Efficiency of insect capture by Sarracenia purpurea(Sarraceniaceae), the northern pitcher plant. Am J Bot 85:88–91
Schaefer HM, Ruxton GD (2008) Fatal attraction: carnivorous plants roll out the red carpet to lure insects. Biol Lett 4:153–155
Schnell DE (1976) Carnivorous plants of the United States and Canada. John F. Blair, Winston-Salem
Schnell DE (2002) The eastern North America pitcher plants (Sarracenia). Carnivorous plants of the United States and Canada. Timber Press, Oregon
Sheridan P (1996) The hooded pitcher plant, Sarracenia minor Walt., at its southern limit. Carniv Plant Newslett 25(2):39–41
Slack A (1979) The trumpet pitchers: Sarracenia. Carnivorous plants. MIT Press, Massachusetts
Thomas DD (2002) Propagation protocol for North American pitcher plants (Sarracenia L.). Nat Plants J 3:50–53
Thoren LM, Tuomi J, Kamarainen T, Laine K (2003) Resource availability affects investment in carnivory in Drosera rotundifolia. New Phytol 159:507–511
Acknowledgments
Special thanks to Brad Norton (JEA) who provided access to the McGirt’s Creek site. We also thank three anonymous reviewers whose comments greatly improved the clarity of the manuscript. This project was funded by the Coastal Biology Program at the University of North Florida.
Author information
Authors and Affiliations
Corresponding author
Additional information
Handling Editor: Stanislav Gorb.
Electronic supplementary material
Below is the link to the electronic supplementary material.
Rights and permissions
About this article
Cite this article
McGregor, J.P., Moon, D.C. & Rossi, A.M. Role of areoles on prey abundance and diversity in the hooded pitcher plant (Sarracenia minor, Sarraceniaceae). Arthropod-Plant Interactions 10, 133–141 (2016). https://doi.org/10.1007/s11829-016-9419-y
Received:
Accepted:
Published:
Issue Date:
DOI: https://doi.org/10.1007/s11829-016-9419-y