Abstract
The study aims to characterize functions of bone morphogenetic protein 2 (BMP2) gene in the process of subcutaneous (SQ) fat deposition of bovine, thereby providing insights into mechanisms for the use of BMP2 in fat management. Our results show that BMP2 was extensively expressed in bovine and relatively rich in adipose tissue. Exogenous BMP2 significantly enhanced proliferation of bovine preadipocytes. Consistently, si-BMP2 apparently induced cell cycle arrest at G0/G1 phase and decreased proliferation of preadipocytes. Meanwhile, exogenous BMP2 mildly enhanced preadipocyte differentiation at day 3 of differentiation, as evidenced by accelerated lipid accumulation, as well as increased mRNA and protein expressions of adipogenic key transcription factor PPARγ; contrary results about lipids were found by BMP2 interference treatment. No difference was observed concerning BMP2 or si-BMP2 treatment at day − 2 and day 0 of differentiation. Additionally, LDN-193189 (inhibitor of BMP type I receptor) pretreatment diminished the enhancement of preadipocyte proliferation and differentiation induced by BMP2, as evidenced by constant proliferation rate and PPARγ expressions. Furthermore, BMP2 markedly enhanced phosphorylation level of SMAD1/5/9, and LDN-193189 could diminish the difference caused by BMP2. Thus, our results suggest that BMP2 triggers BMP/SMAD signaling pathway, promoting both hyperplasia and hypertrophy of bovine preadipocytes.
Similar content being viewed by others
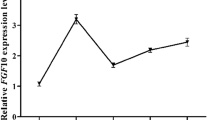
Avoid common mistakes on your manuscript.
Introduction
As a main energy repository in mammals and an active endocrine organ that synthesizes and releases a large number of cytokines, adipose tissue plays an irreplaceable role in maintaining physiological homeostasis (Hausman et al. 2014). Excessive fat accumulation may cause human diseases such as metabolic syndrome (Kloting and Bluher 2014). In addition, fat deposition is also closely relevant to productivity for farm animals (Hausman et al. 2009). Redundant subcutaneous (SQ) fat, for instance, results in reduced net meat ratio, thus the waste of feed energy. Thus, disclosing molecular mechanisms involved in fat development is beneficial to both human health and animal production (Dodson et al. 2010).
Adipose tissue is composed mainly of a large number of adipocytes, which are generated through the hyperplasia and hypertrophy of preadipocytes. The hyperplasia refers to the number increase of adipocytes; the hypertrophy means adipocytes continuously synthesize and accumulate lipids, and finally form large mature adipocytes (Jo et al. 2009; Wei et al. 2013). There are many factors affecting the number and size of adipocytes, among which the molecular regulation of major genes is the key factor determining fat deposition (Cristancho and Lazar 2011). Exploring major genes related to hyperplasia and hypertrophy may help us in better understanding fat accumulation and thus improving animal production efficiency via molecular means.
Bone morphogenetic proteins (BMPs) are members of transforming growth factor beta (TGF-β) superfamily, which have important roles in development and tissue homeostasis (Heldin and Moustakas 2016). Of the BMPs, BMP2 was originally found as an inducer of bone and cartilage formation (Barboza et al. 1999). In addition, emerging evidences have implicated a critical role of BMP2 in adipogenesis regulation. In murine cell lines, BMP2 stimulated differentiation of 3T3-L1 cells if applied together with rosiglitazone (an agonist of PPARγ) (Sottile and Seuwen 2000); BMP2 induced commitment of murine C3H10T1/2 pluripotent stem cells to the adipose lineage (Hata et al. 2003; Huang et al. 2009). In farm animals, BMP2 significantly increased lipid content in porcine intramuscular fat (IMF) preadipocytes, and BMP2 knockdown markedly reduced PPARγ expression in porcine IMF and SQ preadipocytes (Wang et al. 2013). Recently, BMP2 was also reported to promote adipogenesis and slightly inhibit proliferation of human abdominal preadipocytes (Denton et al. 2019). In our previous study, BMP2 was found to be differentially expressed in preadipocytes derived from Wagyu and Angus, which might play a role for the breed differences in fat deposition (Wei et al. 2015). Although the function of promoting preadipocyte differentiation for BMP2 is gradually known, roles of BMP2 in preadipocyte proliferation are rarely studied; furthermore, little is known concerning the roles of BMP2 in adipocytes of bovine. Thus, by using bovine fat-derived preadipocytes, this study aims to unravel BMP2 mechanisms in regulating preadipocyte hyperplasia and hypertrophy, as both number and size increase contribute to fat accumulation. Additionally, BMP2 signaling pathway and expression patterns were also investigated, providing clues for regulating fat expansion.
Materials and methods
Tissue samples
Four Simmental steers that were 24 mo of age were slaughtered at a local abattoir (Nanjing, Jiangsu, China). The Experimental Animal Care and Use Committee of Nanjing Agricultural University approved the use of animals and procedures in this research. Immediately after slaughter, pieces of heart, liver, spleen, lung, kidney, intestine, SQ fat, and longissimus dorsi muscle tissue were obtained. Intramuscular fat tissue pieces were dissected from muscle tissues via ophthalmic scissors and forceps. Sufficient care was taken to avoid contaminating the muscular tissues and the intramuscular fat tissues connected to them.
Cell isolation and culture
Adipose-derived stromal vascular (SV) cells were used as the primary preadipocyte model in this study. The isolation and culture of bovine SV cells were performed as previously described with minor alterations (Wei et al. 2018a). Briefly, SQ fat tissue was washed under sterile conditions and then cut into approximately 1-mm3 sections. The tissue was digested with type I collagenase at 37 °C for 60 min in a shaking water bath, followed by filtration through a 200-μm cell strainer. The filtrate was centrifuged at 1200 rpm for 10 min. After centrifugation, the supernatant containing mature adipocytes was collected for RNA extraction. The pellet containing preadipocytes was re-suspended in DMEM/F12 (HyClone, Logan, UT) + 10% FBS (Sciencell, San Diego, CA) media and incubated at 37 °C in a humidified atmosphere containing 5% CO2. DMEM/F12 medium used in this study was obtained supplemented with 100 IU/mL penicillin and 100 μg/mL streptomycin. All media were changed every 2 or 3 d.
Interference of BMP2 and inhibition of BMP signaling
Three small interfering RNAs (siRNAs) targeting bovine BMP2 and a negative control siRNA (si-NC) were synthesized by GenePharma (Shanghai, China). Using Lipofectamine 2000 (Invitrogen, Carlsbad, CA), preadipocytes were transfected with siRNAs for 6 h following the manufacturer’s protocol. After 48 h of transfection, the cells were collected and BMP2 mRNA levels were detected to select the most effective siRNA. Cells transfected with si-NC were served as the control. Sequences of the siRNAs are listed in Table 1.
To verify whether the activation of BMP signaling was responsible for BMP2-induced proliferation and differentiation, the selective BMP signaling inhibitor LDN-193189 (Selleck, S2618, BMP type I receptor blocking agent) was added to the culture media 1 h before BMP2 treatment. The effectiveness of LDN-193189 was then verified.
Cell proliferation and cell cycle analysis
Bovine preadipocytes were seeded in 96-well culture plates. Cells were incubated with exogenous recombinant BMP2 protein for 48 h. Meanwhile, preadipocytes were transfected with si-BMP2 or si-NC for 6 h, followed by BMP2 treatment for 48 h. Then, effects of BMP2 and si-BMP2 on cell proliferation were assessed using a cell counting kit-8 (CCK-8; Vazyme, Nanjing, China) according to the manufacturer’s protocol, followed by absorbance measurement at 450 nm by a microplate reader (Thermo Fisher Scientific, Waltham, MA).
For cell cycle assay, preadipocytes were seeded in 6-well culture plates and transfected with si-BMP2 or si-NC for 6 h. After 24 h transfection, cell cycle was analyzed as previously described (Wei et al. 2018b). Briefly, cells were collected and fixed with cold 70% ethanol at − 20 °C. The fixed cells were stained with propidium iodide (PI)-RNase staining buffer (BD Pharmingen, San Diego, CA) for 15 min at room temperature. Then, cells were detected by flow cytometer (Becton Dickinson, CA) and at least 10,000 events were recorded per sample. The proliferative index was determined as the percentage of cells in S/G2/M-phase.
Adipogenic differentiation
Two days after confluence (day 0), differentiation of preadipocytes was induced by a standard DMI (inductive differentiation medium) cocktail, containing 1 μg/mL insulin, 1 μM dexamethasone, 0.5 mM isobutylmethylxanthine, and 2 μM rosiglitazone, for 6 d and then maintained in insulin (1 μg/mL) supplemented medium for 2 d. To investigate BMP2 effect on adipogenic differentiation and its underlying mechanisms, preadipocytes were treated with BMP2 or si-BMP2 at day 2, day 0, or day 3 of differentiation, respectively. RNA and protein were extracted by TRIzol (Ambion, Austin, TX) reagent at day 8 of differentiation. Moreover, at day 8 of differentiation, adipocytes were stained with Oil Red-O and observed under an inverted fluorescence microscope (CKX41; Olympus, Tokyo, Japan). Lipid contents were quantified based on the absorbance values at 510 nm of destained Oil Red-O extracted from the cells by a microplate reader.
Real-time quantitative PCR
The real-time quantitative PCR (RT-qPCR) was performed using the StepOne real-time PCR system (Applied Biosystems, Foster, CA) and the SYBR Premix Ex Taq™ (Takara, Dalian, China). Primers were designed and synthesized by Shanghai Sangon Biological Engineering Technology Services Co., Ltd (Shanghai, China). Primer information is shown in Table 2. Glyceraldehyde-3-phosphate dehydrogenase (GAPDH) was used as the reference gene. The data were processed using the 2−△△CT method.
Western blotting analysis
Protein concentrations were measured using a BCA (bicinchoninic acid) protein assay kit (Cell Signaling Technology, Danvers, MA). Equal amounts (10 μg) of protein from each sample were separated by sodium dodecyl sulfate polyacrylamide gel electrophoresis and transferred to polyvinylidene fluoride membranes. After blocking with 5% non-fat milk for 1 h, the membranes were immunoblotted with primary antibodies specific to PPARγ (sc-7196; 1:1000; Santa Cruz Biotechnology, Santa Cruz, CA), p-SMAD1/5/9 (AF8313; 1:1000; Affinity Biosciences, Changzhou, China), SMAD1/5/9 (AF0614; 1:1000; Affinity Biosciences), or GAPDH (AF7021; 1:1000; Affinity Biosciences) overnight at 4 °C, followed by incubation with anti-rabbit IgG secondary antibody for 1 h at room temperature. Then, protein bands were visualized using an enhanced chemiluminescence detection system (Amersham, Piscataway, NJ). Protein band density was quantified and normalized to the GAPDH content.
Statistical analysis
All data were obtained from at least three independent experiments. Results were expressed as mean ± SEM. Statistics were calculated with SPSS software (version 20.0). One-way ANOVA was used for comparisons among groups, followed by Tukey’s test. The two-tailed t test was used to determine a difference between two groups. Differences with P values less than 0.05 and 0.01 were deemed statistically significant and very significant, respectively.
Results
Tissue expressions of BMP2 and its receptor genes in bovine
The tissue expression profile of BMP2 mRNA in bovine is shown in Fig. 1A. BMP2 was widely expressed in various tissues in bovine. The richest expression tissue for BMP2 is intestine, followed by lung, spleen, SQ fat, liver, kidney, IMF, heart, and muscle sequentially. Moreover, mRNA expressions of BMP2 and its receptor genes (Fig. 1B) as well as adipogenic-related genes (Fig. 1C) in SQ and IMF were compared. mRNA expressions of BMP2, BMPR1A, and FABP4 gene in SQ fat were significantly higher than those in IMF tissue (P < 0.05), and mRNA expressions of BMPR2, PPARγ, and C/EBPα were very significantly higher than those in IMF tissue (P < 0.01). No difference was observed for mRNA expressions of BMPR1B gene between SQ and IMF tissue (P > 0.05).
Cellular expression patterns of BMP2 and its receptor genes
During preadipocyte differentiation, mRNA expression of BMP2 was gradually decreased from day 0 to day 6, and then increased at day 8 (Fig. 2A). Similar trend was observed for BMPR1A (Fig. 2B), BMPR1B (Fig. 2C), and BMPR2 (Fig. 2) gene expressions. Compared with day 0 of differentiation, BMP2 and BMPR2 mRNA expressions at day 4 and day 6 decreased significantly (P < 0.05 or P < 0.01), and BMPR1A and BMPR1B decreased at days 2, 4, 6, and 8 of differentiation (P < 0.05 or P < 0.01). Additionally, preadipocytes and mature adipocytes directly isolated from bovine SQ tissue were collected to compare the BMP2 and its receptor gene expressions. As shown in Fig. 2E, mRNA expressions of BMP2 (P < 0.05), BMPR1A (P < 0.01), BMPR1B (P < 0.05), and BMPR2 (P < 0.01) in mature adipocytes were significantly higher than those in preadipocytes (Fig. 2E).
Expressions of BMP2 and its receptor genes in bovine adipocytes. Relative mRNA expressions of BMP2 (A), BMPR1A (B), BMPR1B (C), and BMPR2 (D) at indicated time points during preadipocyte differentiation. Relative mRNA expressions of BMP2, BMPR1A, BMPR1B, and BMPR2 in bovine preadipocytes and mature adipocytes (E). *P < 0.05; **P < 0.01.
Detection of the interference effect of siRNAs targeting BMP2
Three siRNAs (si-BMP2-1, si-BMP2-2, and si-BMP2-3) targeting BMP2 were transfected into bovine preadipocytes, respectively, to detect the interference effect on the cells. Transfection of si-NC was used as the control. After 48 h of transfection, relative BMP2 mRNA levels were measured (Fig. 3). Compared with si-NC-treated group, mRNA levels of BMP2 in si-BMP2-3-treated group very significantly decreased by 70% (P < 0.01), whereas no significant difference was found in si-BMP2-1- and si-BMP2-2-treated groups (P > 0.05). These results suggest that the si-BMP2-3 was usable, and then, it was labeled as si-BMP2 for the further study.
BMP2 increases hyperplasia of bovine preadipocytes
Bovine preadipocytes were treated with various concentrations of exogenous BMP2 for 48 h, and cell proliferation was assessed. As shown in Fig. 4A, 50, 100, and 200 ng/mL BMP2 markedly enhanced proliferation of preadipocytes (P < 0.01). Furthermore, preadipocytes were transfected with si-BMP2, followed by BMP2 treatment for 48 h, and cell proliferation was tested. BMP2 interference very significantly decreased preadipocyte proliferation rate (Fig. 4B; P < 0.01). In the presence of si-BMP2, 50 ng/mL BMP2 could eliminate the difference between the si-BMP2 group and the control group (si-NC treatment group; P > 0.05). Compared with the control group, si-BMP2 with 100 ng/mL BMP2 group enhanced cell proliferation (P < 0.05), and si-BMP2 with 200 ng/mL BMP2 group very significantly increased cell proliferation (P < 0.01).
Effect of BMP2 on proliferation and cell cycle of bovine preadipocytes. Preadipocytes were treated with 0, 50, 100, and 200 ng/mL BMP2 for 48 h and cell proliferation was assessed (A). Preadipocytes were transfected with si-BMP2 or si-NC, followed by 0, 50, 100, and 200 ng/mL BMP2 treatment for 48 h, and cell proliferation was assessed (B). Preadipocytes were transfected with si-BMP2 or si-NC; then, cell cycle (C) and proliferation index (D) were determined after 24 h transfection. *P < 0.05; **P < 0.01.
Preadipocytes were transfected with si-BMP2. After 24 h transfection, the effects of BMP2 on cell cycle distribution were assessed. As shown in Fig. 4C, BMP2 inference very significantly induced G0/G1 cell cycle arrest (P < 0.01), and decreased the percentage of S-phase cells (P < 0.01) and the percentage of G2/M-phase cells (P < 0.05). In addition, the proliferation index was markedly decreased after knocking down BMP2 expression (Fig. 4D). These results indicate that BMP2 is a crucial factor regulating bovine preadipocyte proliferation.
BMP2 enhances hypertrophy of bovine preadipocytes
To determine whether BMP2 has a direct effect on bovine adipocyte differentiation, preadipocytes were induced to differentiate and treated with 100 ng/mL BMP2 (0 ng/mL BMP2 treatment was the control) or si-BMP2 (si-NC treatment was the control) at days − 2, 0, and 3 of differentiation, respectively (Fig. 5). At day 8 of differentiation, lipid accumulation was monitored with Oil Red-O staining under a microscope, followed by quantification of lipid contents at day 8 of differentiation. BMP2 treatment at day 3 significantly enhanced lipid accumulation (P < 0.05; Fig. 5A and C), while si-BMP2 treatment significantly decreased lipid content of adipocytes (P < 0.05; Fig. 5B and C). No significant difference about lipid content was found for groups treated at day − 2 and day 0 of differentiation (P > 0.05; Fig. 5A and B).
Effect of BMP2 on differentiation of bovine preadipocytes. Preadipocytes were induced to differentiate and treated with 100 ng/mL BMP2 (A) for 48 h or si-BMP2 (B) for 6 h at day − 2, day 0, and day 3 of differentiation, respectively. At day 8 of differentiation, cells were stained with Oil Red-O staining, followed by quantification of lipid contents. For cells treated by BMP2 or si-BMP2 at day 3 of differentiation, lipid accumulation was monitored with Oil Red-O staining under a microscope (C; × 200 magnification). *P < 0.05.
With the treatment of BMP2 at day 3 of differentiation, mRNA expressions of key adipogenic genes (PPARγ, C/EBPα, and FABP4), as well as BMP2 receptors (including BMPR1A, BMPR1B, and BMPR2) at day 8 of differentiation were determined. As shown in Fig. 6A, compared with blank control, BMP2 treatment at day 3 dramatically increased expressions of PPARγ (P < 0.01), FABP4 (P < 0.05), and BMPR1B (P < 0.05). Consistently, protein expression levels of PPARγ in BMP2-treated cells were slightly enhanced on day 8 of differentiation (P < 0.01; Fig. 6B). No difference was observed for mRNA expressions of C/EBPα, BMPR1A, and BMPR2 (Fig. 6A). Results from both lipid contents and marker gene expression analysis for cells treated with BMP2 at day 3 indicated that BMP2 promoted differentiation of bovine preadipocytes.
Effect of BMP2 on relative expressions of adipogenic-related genes in bovine preadipocytes. Preadipocytes were induced to differentiate and treated with 100 ng/mL BMP2 at day 3 of differentiation for 48 h. Then, cells were harvested for RT-qPCR analysis (A) and Western blotting test (B) at day 8 of differentiation. *P < 0.05; **P < 0.01.
BMP2 promotes growth of preadipocytes via SMAD1/5/9 signaling
To verify whether the activation of BMP/SMAD signaling was responsible for BMP2-induced proliferation and differentiation, the BMP signaling inhibitor LDN-193189 was added to the culture media 1 h before BMP2 treatment. The effectiveness of LDN-193189 was verified by CCK-8, RT-qPCR, and Western blotting. In the presence of LDN-193189, exogenous BMP2 failed to change proliferation rate (Fig. 7A). Moreover, under the use of LDN-193189, BMP2 treatment did not change both mRNA (Fig. 7B) and protein (Fig. 7C) expressions PPARγ (a key adipogenic marker gene). Results from both proliferative ability and marker gene expression analysis indicated that LDN-193189 pretreatment distinctively eliminated the alteration about proliferation and differentiation induced by exogenous BMP2.
Effect of BMP2 and its receptor inhibitor on differentiation and proliferation of bovine preadipocytes. Preadipocytes were pretreated with LDN-193189 for 1 h, followed by 100 ng/mL BMP2 treatment for 48 h. Then, cell proliferation was assessed (A). Preadipocytes were induced to differentiate and treated with LDN-193189 at day 3 of differentiation for 1 h, followed by 100 ng/mL BMP2 treatment for 48 h. Then, cells were harvested for RT-qPCR analysis (B) and Western blotting test (C) at day 8 of differentiation. **P < 0.01.
Furthermore, effect of BMP2 and LDN-193189 on SMAD signaling of bovine preadipocytes was examined. As shown in Fig. 8, phosphorylation level of SMAD1/5/9 increased significantly by BMP2. Additionally, pretreatment with LDN-193189 eliminated the p-SMAD1/5/9 difference induced by BMP2, indicating the BMP2/SMAD signaling play a vital role to induce hyperplasia and hypertrophy of bovine preadipocytes.
Discussion
The regulation of fat deposition is an important topic of research in both human health and farm animal production (Hausman et al. 2014; Dodson et al. 2015). As adipocyte number and size increase regulated by key genes contribute to fat accumulation (Jo et al. 2009; Lee et al. 2018; Schleinitz et al. 2014), exploring potential genes regulating proliferation and differentiation is a vital approach to develop potential molecular means that modulate fat development. The present study provides the first evidence that BMP2 enhances both number increase and lipid accumulation of bovine preadipocytes in vitro. In addition, our data indicate that the simulative effect of BMP2 on growth of adipocytes is mediated through BMP/SMAD signaling pathway.
To rapidly acquire valuable information about bovine BMP2, tissue expression profile of BMP2 mRNA in bovine was first detected. As shown by our results, BMP2 was widely expressed in various tissues and relatively rich in adipose tissue. In addition, expressions of BMP2 and its receptors were higher in SQ fat than in IMF, which was consistent with adipogenic gene expression modes in these two fat tissues, indicating BMP2 might play a positive role in bovine fat tissue accumulation.
It has been shown that the expression of BMP2 is markedly upregulated at day 3 and day 5 and then slightly downregulated during the differentiation of porcine SQ and IMF preadipocytes, respectively (Wang et al. 2013). Additionally, during differentiation of human SQ preadipocytes, only a tiny fluctuation exists for the expression of BMP2 (Denton et al. 2019). Unlike these reports, in the present study, based on results of bovine preadipocytes and mature adipocytes, BMP2 expression was sharply decreased at day 4 and recovered at the late stage of differentiation, with the highest expression of BMP2 in mature adipocytes. These results infer expression pattern of BMP2 during adipogenesis which is different for various species and there might be differences concerning BMP2 functions in preadipocytes among diverse species.
Previous studies have indicated that BMP2 has notable effects on cell proliferation in several tumor cell types (Wang et al. 2017; Fukuda et al. 2021). Moreover, BMP2 (10–200 ng/mL) promotes proliferation in 3T3-F442A cells (Skillington et al. 2002), while 100 ng/mL BMP2 reduces proliferation in human adipose stem cells (Vanhatupa et al. 2015) and human SQ preadipocytes. To assess whether BMP2 regulates proliferation of bovine preadipocytes, we used the gain-of-function and reduction-of-function assays. In this study, 50–200 ng/mL BMP2 was found to profoundly enhance cell proliferation by 51–83%. Although BMP2 has been shown to exert the pro-proliferative effect in a dose-dependent manner (Skillington et al. 2002), such an event was not observed in this study. Consistent with BMP2 treatment data, BMP2 interference reduced 42% cell proliferation rate, with the reduction of proliferation index. The inhibitory effect on cell growth appears to be due to the cell cycle arrest at the G0/G1 phase after BMP2 knockdown. Moreover, BMP2 treatment effectively eliminated the proliferative difference caused by BMP2 interference, further confirming the notable pro-proliferative effect of BMP2 on bovine preadipocytes.
As both increased number and expanded volume of adipocytes contribute to fat accumulation, we further focused BMP2 functions in differentiation of bovine preadipocytes. Previous reports have indicated that BMP2 effects on adipogenesis are variable and depend on cell types and experimental conditions (Margoni et al. 2012; Lee 2018). Via stimulating PPARγ expression, BMP2 is known to induce adipogenic commitment as well as differentiation in multiple cell culture models including 10T1/2 cells (Huang et al. 2009), 3T3-L1 cells (Sottile and Seuwen 2000), and human ASCs (Lee et al. 2014). Contradictory results have also been reported. In human ASCs, BMP2 pretreatment failed to translate stem cells to adipose lineage cells (Denton et al. 2019) and exhibited a donor-dependent role for adipogenic or osteogenic differentiation (Vanhatupa et al. 2015); in 3T3-F442A cells, BMP2 slightly increases differentiation in the absence of insulin, but inhibits insulin-induced adipocyte differentiation (Skillington et al. 2002). Moreover, BMP2 is shown to regulate differentiation of preadipocytes in a depot-specific manner (Wang et al. 2013; Denton et al. 2019). Our data show that BMP2 had no effect on differentiation of bovine SQ preadipocytes when cells were treated at day − 2 or day 0 of differentiation, which was in line with the report that exogenous BMP2 treatment does not change lipid accumulation of pig SQ preadipocytes (Wang et al. 2013). However, we found that BMP2 treatment in preadipocytes at day 3 (mid-stage) of differentiation accelerated lipid accumulation in bovine adipocytes, as evidenced by morphologic and quantitative data of the amount of triglyceride; contrary results were observed by si-BMP2 treatment. Furthermore, our data show that 100 ng/mL BMP2 treatment at day 3 significantly increased expressions of adipogenic marker genes. Taken together, the combined results above strongly suggest that BMP2 positively regulates differentiation of bovine preadipocytes at the mid-stage of differentiation. Although different cell types and the differential BMP2 expression patterns during adipogenesis may provide explanations for the contradictory effects of BMP2 functions in various adipocytes, further studies are also needed to reconcile the differences.
As previously reported, BMPs execute their biological function via forming a complex with type I and II serine/threonine kinase receptors. These receptors, in turn, phosphorylate and activate receptor-mediated SMAD proteins, including SMAD1, 5, 8, or 9 (Lee 2018; Massagué and Wotton 2000; Nohe et al. 2002). Consistently, we show that BMP2 receptors, including BMPR1A, BMPR1B, and BMPR2, were present in bovine fat tissue as well as fat cells. Similarly with BMP2 expression mode, expressions of these receptors were decreased at mid-stage and recovered at late stage of differentiation. To investigate whether the activation of BMP-SMAD signaling was responsible for BMP2-induced proliferation and differentiation, the BMP signaling inhibitor, LDN-193189 (type I receptor inhibitor), was used to pretreat preadipocytes. Results from both cell numbers and PPARγ expressions indicated that LDN-193189 pretreatment significantly compromised BMP2-induced upregulation in both proliferation and differentiation. Additionally, BMP2 significantly induced phosphorylation of SMAD1/5/9, and LDN-193189 pretreatment effectively blocked the signal activation. Therefore, our data demonstrate that bovine preadipocytes possessed the functional BMP signaling pathway with BMP receptors and SMAD1/5/9; activation of BMP/SMAD signaling by BMP2 could lead to preadipocyte hypertrophy and hyperplasia in bovine.
Conclusion
BMP2 functions in adipocytes may vary according to the species, cell types, and dosage and time. To the best of our knowledge, this is the first study demonstrating BMP2 functions in bovine preadipocytes. The present study uncovers expression patterns of bovine BMP2 in various tissues and adipocytes in different differentiation stages. We show that BMP2 was relatively abundant in fat tissue and was lowest when expressed at the mid-stage of differentiation. Additionally, BMP2 appeared to notably promote proliferation of bovine preadipocytes via regulating G0/G1 cell cycle. It is worth mentioning that various treatment time points were performed to detect BMP2 effect on differentiation, and BMP2 mildly enhanced preadipocyte differentiation when co-incubating with BMP2 or si-BMP2 only at the mid-stage of differentiation. Increased expressions of adipogenic transcription factors including PPARγ and FABP4 contribute to the pro-adipogenic effects of BMP2. Furthermore, both the pro-proliferative and the pro-adipogenic effects of BMP2 on bovine preadipocytes were through BMP2/BMPRs/SMAD1/5/9 signaling. Together, our study helps to elucidate the differentiation patterns of bovine preadipocytes and further reveals the function of the BMP2 gene in bovine adipocytes, thus providing insights into mechanisms for the use of BMP2 in fat management.
References
Barboza E, Caula A, Machado F (1999) Potential of recombinant human bone morphogenetic protein-2 in bone regeneration. Implant Dent 8:360–367. https://doi.org/10.1097/00008505-199904000-00006
Cristancho AG, Lazar MA (2011) Forming functional fat: a growing understanding of adipocyte differentiation. Nat Rev Mol Cell Biol 12:722–734. https://doi.org/10.1038/nrm3198
Denton NF, Eghleilib M, Al-Sharifi S, Todorcevic M, Neville MJ, Loh N et al (2019) Correction to: Bone morphogenetic protein 2 is a depot-specific regulator of human adipogenesis. Int J Obes (lond) 43:2593. https://doi.org/10.1038/s41366-019-0475-0
Dodson MV, Allen RE, Du M, Bergen WG, Velleman SG, Poulos SP et al (2015) INVITED REVIEW: Evolution of meat animal growth research during the past 50 years: adipose and muscle stem cells. J Anim Sci 93:457–481. https://doi.org/10.2527/jas.2014-8221
Dodson MV, Hausman GJ, Guan L, Du M, Rasmussen TP, Poulos SP et al (2010) Lipid metabolism, adipocyte depot physiology and utilization of meat animals as experimental models for metabolic research. Int J Biol Sci 6:691–699. https://doi.org/10.7150/ijbs.6.691
Fukuda T, Fukuda R, Koinuma D, Moustakas A, Miyazono K, Heldin CH (2021) BMP2-induction of FN14 promotes protumorigenic signaling in gynecologic cancer cells. Cell Signal 87:110146. https://doi.org/10.1016/j.cellsig.2021.110146
Hata K, Nishimura R, Ikeda F, Yamashita K, Matsubara T, Nokubi T et al (2003) Differential roles of Smad1 and p38 kinase in regulation of peroxisome proliferator-activating receptor gamma during bone morphogenetic protein 2-induced adipogenesis. Mol Biol Cell 14:545–555. https://doi.org/10.1091/mbc.e02-06-0356
Hausman GJ, Basu U, Wei S, Hausman DB, Dodson MV (2014) Preadipocyte and adipose tissue differentiation in meat animals: influence of species and anatomical location. Annu Rev Anim Biosci 2:323–351. https://doi.org/10.1146/annurev-animal-022513-114211
Hausman GJ, Dodson MV, Ajuwon K, Azain M, Barnes KM, Guan LL et al (2009) Board-invited review: the biology and regulation of preadipocytes and adipocytes in meat animals. J Anim Sci 87:1218–1246. https://doi.org/10.2527/jas.2008-1427
Heldin CH, Moustakas A (2016) Signaling receptors for TGF-β family members. Cold Spring Harb Perspect Biol 8(8):a022053. https://doi.org/10.1101/cshperspect.a022053
Huang H, Song TJ, Li X, Hu L, He Q, Liu M et al (2009) BMP signaling pathway is required for commitment of C3H10T1/2 pluripotent stem cells to the adipocyte lineage. Proc Natl Acad Sci U S A 106:12670–12675. https://doi.org/10.1073/pnas.0906266106
Jo J, Gavrilova O, Pack S, Jou W, Mullen S, Sumner AE et al (2009) Hypertrophy and/or hyperplasia: dynamics of adipose tissue growth. PLoS Comput Biol 5:e1000324. https://doi.org/10.1371/journal.pcbi.1000324
Kloting N, Bluher M (2014) Adipocyte dysfunction, inflammation and metabolic syndrome. Rev Endocr Metab Disord 15:277–287. https://doi.org/10.1007/s11154-014-9301-0
Lee MJ (2018) Transforming growth factor beta superfamily regulation of adipose tissue biology in obesity. Biochim Biophys Acta Mol Basis Dis 1864:1160–1171. https://doi.org/10.1016/j.bbadis.2018.01.025
Lee SY, Lee JH, Kim JY, Bae YC, Suh KT, Jung JS (2014) BMP2 increases adipogenic differentiation in the presence of dexamethasone, which is inhibited by the treatment of TNF-alpha in human adipose tissue-derived stromal cells. Cell Physiol Biochem 34:1339–1350. https://doi.org/10.1159/000366341
Lee VR, Barr KJ, Kelly JJ, Johnston D, Brown CFC, Robb KP et al (2018) Pannexin 1 regulates adipose stromal cell differentiation and fat accumulation. Sci Rep 8:16166. https://doi.org/10.1038/s41598-018-34234-9
Margoni A, Fotis L, Papavassiliou AG (2012) The transforming growth factor-beta/bone morphogenetic protein signalling pathway in adipogenesis. Int J Biochem Cell Biol 44:475–479. https://doi.org/10.1016/j.biocel.2011.12.014
Massagué J, Wotton D (2000) Transcriptional control by the TGF-beta/Smad signaling system. EMBO J 19:1745–1754. https://doi.org/10.1093/emboj/19.8.1745
Nohe A, Hassel S, Ehrlich M, Neubauer F, Sebald W, Henis YI et al (2002) The mode of bone morphogenetic protein (BMP) receptor oligomerization determines different BMP-2 signaling pathways. J Biol Chem 277:5330–5338. https://doi.org/10.1074/jbc.M102750200
Schleinitz D, Bottcher Y, Bluher M, Kovacs P (2014) The genetics of fat distribution. Diabetologia 57:1276–1286. https://doi.org/10.1007/s00125-014-3214-z
Skillington J, Choy L, Derynck R (2002) Bone morphogenetic protein and retinoic acid signaling cooperate to induce osteoblast differentiation of preadipocytes. J Cell Biol 159:135–146. https://doi.org/10.1083/jcb.200204060
Sottile V, Seuwen K (2000) Bone morphogenetic protein-2 stimulates adipogenic differentiation of mesenchymal precursor cells in synergy with BRL 49653 (rosiglitazone). FEBS Lett 475:201–204. https://doi.org/10.1016/s0014-5793(00)01655-0
Vanhatupa S, Ojansivu M, Autio R, Juntunen M, Miettinen S (2015) Bone morphogenetic protein-2 induces donor-dependent osteogenic and adipogenic differentiation in human adipose stem cells. Stem Cells Transl Med 4:1391–1402. https://doi.org/10.5966/sctm.2015-0042
Wang MH, Zhou XM, Zhang MY, Shi L, Xiao RW, Zeng LS et al. (2017) BMP2 promotes proliferation and invasion of nasopharyngeal carcinoma cells via mTORC1 pathway. Aging (Albany NY) 9: 1326–1340. https://doi.org/10.18632/aging.101230.
Wang S, Zhou G, Shu G, Wang L, Zhu X, Gao P et al (2013) Glucose utilization, lipid metabolism and BMP-Smad signaling pathway of porcine intramuscular preadipocytes compared with subcutaneous preadipocytes. Cell Physiol Biochem 31:981–996. https://doi.org/10.1159/000350116
Wei S, Fu X, Liang X, Zhu MJ, Jiang Z, Parish SM et al (2015) Enhanced mitogenesis in stromal vascular cells derived from subcutaneous adipose tissue of Wagyu compared with those of Angus cattle. J Anim Sci 93:1015–1024. https://doi.org/10.2527/jas.2014-7923
Wei S, Zhang L, Zhou X, Du M, Jiang Z, Hausman GJ et al (2013) Emerging roles of zinc finger proteins in regulating adipogenesis. Cell Mol Life Sci 70:4569–4584. https://doi.org/10.1007/s00018-013-1395-0
Wei S, Zhang M, Zheng Y, Yan P (2018a) ZBTB16 overexpression enhances white adipogenesis and induces brown-like adipocyte formation of bovine white intramuscular preadipocytes. Cell Physiol Biochem 48:2528–2538. https://doi.org/10.1159/000492697
Wei S, Zheng Y, Zhang M, Zheng H, Yan P (2018b) Grape seed procyanidin extract inhibits adipogenesis and stimulates lipolysis of porcine adipocytes in vitro. J Anim Sci 96:2753–2762. https://doi.org/10.1093/jas/sky158
Funding
This project was supported by the Fundamental Research Funds for the Central Universities of China (KYYJ202102), the Jiangsu Agricultural Science and Technology Innovation Fund (CX(21)3136), and the National Natural Science Foundation of China (31501930).
Author information
Authors and Affiliations
Corresponding author
Ethics declarations
Conflict of interest
The authors declare no competing interests.
Rights and permissions
About this article
Cite this article
Yang, L., Hao, W., Wang, H. et al. BMP2 increases hyperplasia and hypertrophy of bovine subcutaneous preadipocytes via BMP/SMAD signaling. In Vitro Cell.Dev.Biol.-Animal 58, 210–219 (2022). https://doi.org/10.1007/s11626-022-00661-2
Received:
Accepted:
Published:
Issue Date:
DOI: https://doi.org/10.1007/s11626-022-00661-2