Abstract
Biological denitrification is the most promising alternative approach for the removal of nitrate from wastewater. MBBR inoculated with activated sludge is a widely studied approach, but very few studies have focused on the bioaugmentation of biofilm forming bacteria in MBBR. Our study revealed that the use of special microbial seed of biofilm forming denitrifying bacteria Diaphorobacter sp. R4, Pannonibacter sp. V5, Thauera sp. V9, Pseudomonas sp.V11, and Thauera sp.V14 to form biofilm on carriers enhanced nitrate removal performance of developed MBBR. Various process parameters C/N ratio 0.3, HRT 3 h at Nitrate loading 2400 mg L−1, Filling ratio 20%, operated with Pall ring carrier were optimized to achieve highest nitrate removal. After 300 days of continuous operation results of whole genome metagenomic studies showed that Thauera spp. were the most dominant and key contributor to the denitrification of nitrate containing wastewater and the reactor was totally conditioned for denitrification. Overall, findings suggest that bench-scale MBBR developed with biofilm forming denitrifying microbial seed accelerated the denitrification process; therefore in conclusion it is suggested as one of the best suitable and effective approach for removal of nitrate from wastewater.
Similar content being viewed by others
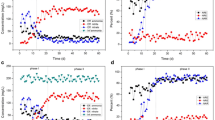
Explore related subjects
Discover the latest articles, news and stories from top researchers in related subjects.Avoid common mistakes on your manuscript.
Introduction
Nitrate is a widespread pollutant, which adversely affects human health and the environment. Sources of nitrate contamination in water include septic tank emissions, manure, domestic wastewater, industrial sewage, and excessive use of fertilizers (Qambrani et al. 2013). Excessive nitrate in water may cause infant cyanosis syndrome or “blue baby syndrome” due to methemoglobinemia (Skold et al. 2011), cancer attributed to nitrosamines formation, and eutrophication in groundwater due to harmful algal bloom, fish hypoxia, and toxin production (Camargo and Alonso 2006). The heterotrophic denitrification process is one of the most efficient, cost-effective, and environment-friendly process for the removal of nitrate from wastewater (Ashok and Hait 2015). Biological denitrification is an anaerobic process where denitrifying bacteria reduce nitrate to N2O or N2 in low dissolved oxygen aquatic environments (Suhr et al. 2013). In recent years, number of denitrifying bacteria such as Corynebacterium pollutisoli SPH6 (Liu et al. 2018), Pannonibacter phragmitetus (Bai et al. 2019), Paracoccus denitrificans Z195 (Chakravarthy et al. 2011; Zhang et al. 2020), Pseudomonas sp. H117 (Su et al. 2020) and bioaugmentation of denitrifier with biofilm-formation and nitrogen removal capacities (Hong et al. 2020) have been reported to have potential applications in nitrate and nitrogen removal from different wastewaters thus underlining the usefulness of biological denitrification.
Biofilm reactors have replaced conventional activated sludge processes (CAS) as they posses several advantages like high biomass concentrations, good removal efficiency, less space requirement, negligible sludge recycling, and upgradability from CAS to MBBR (Ødegaard 2006). MBBR is highly effective biofilm reactor in which biofilm grows on specially designed carriers that move freely in the reactor (Ødegaard 2006). It has also proven to be very suitable for the removal of different pollutants from wastewaters (Leyva-Díaz et al. 2017).
There are some practical problems for treating wastewater in MBBR where the microorganisms are immobilized in biofilms on the carrier matrix. MBBR is generally inoculated with sludge rich in microorganisms from wastewater treatment plants, to form the biofilm on the surface of the carriers. However, it tends to often poor performance due to lack of functional microorganisms, which can negatively affect pollutant removal efficiency and overall reactor performance capacity. Presence of biofilm forming bacteria only without the requisite functional attribute may not effectively achieve pollutant removal. Moreover, simply mixing of nonfunctional biofilm-forming bacteria with degrading bacteria to facilitate adherence to the matrix, may cause mutual growth inhibition, slow biofilm development, complex operation among other issues (Li et al. 2016).
The biofilm forming bacteria contribute through specific surface molecules which play a significant role in the production of multispecies biofilms in various environments. Amyloid are surface associated proteins widely present in natural biofilms and flocs of activated sludge (Larsen et al. 2007). Amyloid proteins are folded as β-sheet rich fibrils, stacked perpendicular to the fibrillar axis (Chapman et al. 2002). They have been studied well in Streptomycetes, E. coli, Salmonella species, fungi, etc. (Gebbink et al. 2005). Since amyloids are the basis of biofilm structure of bacteria, amyloid producing bacteria should form a stable biofilm. In this perspective, the aim of the present study was to develop a moving bed biofilm reactor with specially screened amyloid producing bacteria with denitrification ability. Detailed performance analysis of this MBBR, optimization of different factors affecting nitrate removal efficiency, and analysis of community structure in the denitrifying MBBR after long term operation were the studies undertaken. This work should provide a promising prospect for the bioaugmentation of a specific consortium of biofilm forming denitrifying bacteria in bench-scale MBBR for the treatment of nitrate containing wastewater.
Materials and methods
Isolation of biofilm forming denitrifying bacteria
Isolation was carried out from activated sludge flocs collected from three different sewage treatment plants. The samples were further inoculated in Winogradsky’s column for enrichment of denitrifying bacteria with sodium acetate as a carbon source and potassium nitrate as a nitrogen source. After every three days, denitrifiers were enumerated by most probable number (MPN) test in Peptone Nitrate broth (PNB) contained in a test tube with inverted Durham’s tube (0.5 g Peptone, 0.3 g Beef extract, 1 g Potassium nitrate in 100 mL distilled water). The isolation of denitrifiers was carried out on CPNA (Congo red peptone nitrate agar) medium (0.5 g Peptone, 0.3 g Beef extract, 1 g Potassium nitrate, 2 g Agar, 0.005 g Congo red in 100 mL of distilled water).
Denitrification test
Qualitative denitrification test was carried out by inoculating 100 µL of a culture of the isolates in 5 mL of (PNB) with Durham’s tube and incubated at 37 °C for 48 h in static condition. Gas in Durham’s tube was considered a positive test.
Denitrification efficiency measurement
The isolates were grown in PNB for 18–24 h and centrifuged at 8000 rpm for 7 min. The cell pellet was washed twice with phosphate buffered saline (PBS) and resuspended in PBS with absorbance set at 0.5 OD600nm. 1% of this was inoculated in 100 mL of flask containing 50 mL MM2 medium prepared from 0.2 g MgSO4·7H2O, 0.2 g K2HPO4, 0.05 g FeSO4·7H20, 0.02 g CaCl2·2H2O, 0.002 g MnCl2·4H2O, 0.001 g NaMoO4·2H2O, 1 g KNO3, 0.6% Sodium acetate, 0.5 g Yeast extract per liter of distilled water. Experimental flasks were incubated at 37 °C for 48 h in static conditions, aliquots were drawn at 12 h intervals, and assayed for Nitrate, Nitrite, and Ammonia (Srinandan et al. 2011).
Denitrification efficiency was calculated by the following formula
Microtiter plate assay for biofilm formation
For microtiter plate assay isolates were grown in PNB for 18–24 h and centrifuged at 8000 rpm for 7 min. The cell pellet was washed twice with PBS and resuspended in PBS with an absorbance of 0.5 OD600nm. 40 μL of cells were inoculated in 24 wells microtiter plate with 1960 μL of MM2 medium. After 48 h of incubation, the wells were rinsed five times with 1.5 mL of sterile PBS to remove any adhering planktonic cells. The biofilm formed on the plate was then stained with 1.5 mL of 1% crystal violet for 45 min, rinsed five times with 1.5 mL of distilled water, and destained with 70% ethanol for 15 min. The absorbance of the decanted solution was measured in Tecan (InfiniteM200pro) microtiter plate reader at 595 nm (Srinandan et al. 2010).
Thioflavin T staining for amyloid production
Qualitative amyloid production by the selected isolates was detected by the Thioflavin T staining method. The stock solution was prepared by dissolving 32 mg of Thioflavin T in 1 mL distilled water and the solution was stored in dark at 4 °C. The working solution was freshly prepared by diluting the stock solution 1:2 and used immediately. The smears were treated with Thioflavin T solution for 5 min in dark, rinsed with distilled water, and air dried before observing under an Olympus BX41 fluorescence microscope (Berg et al. 2010).
Identification of selected isolates
Identification of isolates was carried out using the 16s rRNA sequencing method with bacterial universal primers 8F (AGAGTTTGATCCTGGCTCAG) and 1492R (GGTTACCTTGTTACGACTT). The gene sequences of isolates were compared to those in the GenBank database using the Basic Local Alignment Search Tool (BLAST).
Inoculum preparation for continuous MBBR studies
A special bacterial seed consortium was developed by growing all the selected isolates in PNB for 24 h. The absorbance of 0.5 OD600nm was set and 400 µL of each isolates were pooled to make 2 mL final volume. The cell pellet obtained after centrifugation at 8000 rpm for 7 min was washed twice with PBS and resuspended in the same volume of PBS. 1 mL of this suspension of the consortium was then added in the 100 mL of MM2 medium and incubated at 37 °C under the static condition for 24 h to be used as inoculum.
Bench-scale denitrifying MBBR developed with special microbial seed
A schematic representation of the denitrifying MBBR used in this study is shown in Fig. 1. The reactor was constructed from the polyacrylic material of 45 cm in height and 16 cm in width with a working volume of 10 L. A submersible pump fixed at the center of the reactor facilitated the movement of the carrier inside the reactor. Synthetic effluent (MM2 medium) was continuously fed from the inlet tank to the reactor with the peristaltic pump (Masterflex®). pH 8, DO-0.1–0.8 mg L−1, and turbidity was 15–350 NTU was maintained throughout in the operation of MBBR.100 mL inoculum prepared as mentioned in previous section was added in the 10 L reactor containing carriers upon which the biofilm was allowed to form for 10 days. For continuous reactor studies, synthetic effluent was continuously fed from the inlet tank into the reactor with the peristaltic pump and treated effluent was collected in the outlet tank and assayed for nitrate, nitrite, ammonia, pH, turbidity, biomass, and DO. Various operational parameters to gauge the performance of MBBR were optimized such as (C/N) ratio (the amount of available carbon source consumed to the amount of nitrogen compounds reduced), HRT (time spent by the influent inside the reactor), Nitrate loading (concentration of influent nitrate), Different carrier designs (Pall ring, Kaldnes K1, and Fluidized biomedia) and Filling ratio (amount of carriers added in the reactor in %).
Analytical methods
Nitrate, Nitrite, Ammonia, COD estimation methods were performed according to APHA 1998. DO was measured using a DO probe (Thermo Scientific) and the turbidity was checked using a Turbidity meter (Hanna instruments). Quantification of biomass on the carriers was carried out by drying carrier material at 105 °C for 1 h. Biomass was scraped from carrier material and the dry weight of biomass was measured.
Analysis of biofilm composition
Biomass adhered to carriers collected from four locations in the reactor were scraped off and EPS extracted using the cation exchange resin (CER) method. 30 mL of EPS was centrifuged (8000 rpm, 15 min, 4 °C). The extracted biomass was washed twice with PBS (pH ~ 7.0) and resuspended to a volume of 30 mL with CER (70 g g−1 dry cells). Centrifuge tubes were shaken at 250 rpm for 2 h at 4 °C and then kept static for 5 min to settle the CER. Then, the suspensions were centrifuged (10,000×g, 15 min, 4 °C) and filtered using a 0.45 μm acetate filter membrane. Finally, the EPS was obtained and stored at − 20 °C until analysis (Hong et al. 2020). Carbohydrate was estimated by Phenol sulfuric method (Masuko et al. 2005), Protein by Folin Lowry method (Chen 1996), and Lipid by Phosphovanillin method (Frings et al. 1972).
Microbial community analysis of biofilm developed in optimized MBBR
After 300 days of continuous reactor operation, gDNA was extracted using the CTAB method from three biofilm samples of twelve carriers each collected from different locations in the reactor (Andreou 2013). It was further purified by using a Geneipure quick PCR purification kit. The whole-genome metagenome sequencing was outsourced to MEDGENOME Bangalore, India. Libraries were prepared using NEBNext and sequencing was done by using Illumina HiSeq X system to generate 2X150 bp sequence reads. Around 7–9 Gb data was generated for all the samples. Further, de novo metagenome assembly was carried out using metaspades assembler (v3.11.1) and Open reading frame (ORF) prediction was done using Prodigal (v2.6.3). Organism’s abundance was predicted up to species level by using the Kraken2 tool. Kegg-Kaas was used to predict genes involved in different metabolic pathways.
Results
Isolation, screening, and identification of biofilm forming denitrifying bacteria for the development of special microbial seed
Isolation of amyloid producing denitrifying bacteria carried out from activated sludge sample yielded 33 morphologically different isolates on CPNA plates. Congo red dye has been used to distinguish amyloid and non amyloid producing bacteria as the former exhibit red colonies when grown on agar plates containing Congo red. Congo red specifically binds to the β-sheets of the amyloid proteins imparting red color to the colonies. Out of 33 isolates obtained on CPNA, 24 isolates also showed gas production in PNB indicating that they possessed denitrification activity. Further screening of the isolates based on their denitrification efficiency, lack of nitrite accumulation, and biofilm formation revealed that out of 24 isolates 9 isolates showed denitrification efficiency above 80%; 12 isolates showed good biofilm forming ability (Supplementary Fig. 1b, c) out of which 5 isolates showed denitrification efficiency above 80%, good biofilm forming ability, and also did not show nitrite accumulation. Gas accumulated by bacteria can be nitrous oxide, nitric oxide, and nitrogen all of which are the products of denitrification pathway (Gamble et al. 1977). Accumulation of nitrite by the isolates was checked because it is even more toxic than nitrate due to the formation of carcinogenic N-nitroso compounds (Skold et al. 2011).
Finally, a consortium of the five selected isolates R4, V5, V9, V11, and V14 showing high denitrification efficiency, biofilm forming ability coupled with no nitrite accumulation termed here as special seed consortium was used in the operation of the MBBR for optimization studies. Identification of the selected R4, V5, V9, V11, and V14 isolates in two separate analysis showed 99%, 99%, 100%, 100%, and 99% sequence similarity with Diaphorobacter sp., Pannonibacter sp., Thauera sp., Pseudomonas sp., and Thauera sp., respectively and its sequences were deposited in the GenBank database with the accession number MN880203, MN880207, MN880204, MN880206, and MN880205, respectively. Further, confirmation of amyloid production by the selected isolates was carried out by Thioflavin T staining. It is the most widely used “gold standard” for selectively staining and identifying amyloid produced by the bacteria. The amyloid proteins produced by the bacteria extracellularly coat the cell surface and take up Thioflavin and display green fluorescence all over the bacterial cell. All the five isolates were stained fluorescent green by Thioflavin T thereby confirming production of extracellular amyloid protein.
Denitrification efficiency measurement
Flask level studies depicted in Fig. 2a showed that Diaphorobacter sp. R4, Pannonibacter sp. V5, Thauera sp.V9, Pseudomonas sp.V11 and Thauera sp.V14 gave, 78%, 80% 64%, 100% and 95.5% nitrate removal, respectively. On the other hand, the consortium of all the isolates showed complete nitrate reduction at initial nitrate concentration of 200 mg L−1 from 10 h onwards. Individually isolates Diaphorobacter sp. R4, Pannonibacter sp. V5 and Pseudomonas sp.V11 showed nitrite accumulation between 12 and 48 h, which decreased after 48 h while the Thauera sp.V9, Thauera sp.V14 and consortium, showed no nitrite accumulation from the beginning itself (Fig. 2b). No ammonia was detected in the flask. All the isolates showed synergistic effect and cooperated to give enhanced nitrate removal efficiency and no nitrite accumulation, which clearly showed that there is a distinct advantage in using the bacterial seed consortium.
Denitrification studies in continuous denitrifying MBBR developed with special microbial seed
MBBR performance at different C/N ratio
In heterotrophic denitrification process acetate has been one of the most widely used external carbon source that was found to increase denitrification rate in comparison to methanol and glucose (Gong et al. 2013). In the continuous reactor studies, here too acetate was used as a carbon source. As seen in Fig. 3a at different C/N ratios 0.7, 0.4 and 0.3 nitrate removal was above 95% with an initial nitrate concentration of 620 mg L−1. However, COD was above permissible range (i.e. 250 mg L−1) at C/N ratio 0.7, whereas C/N ratio 0.4, 0.3, 0.2 showed 96%, 100% and 78% nitrate removal, respectively and COD below permissible range (Fig. 3a). Thus, overall results of Fig. 3a revealed that higher C/N ratio of 0.7 increased COD concentration in wastewater and can cause secondary pollution in wastewater; on the other hand, lower C/N ratio of 0.2 decreased nitrate removal efficiency. Hence, 0.3 C/N ratio was selected for further studies as the reactor run at this ratio was able to remove 100% of nitrate (620 mg L−1) at the same time reducing the COD below permissible range (i.e.250 mg L−1).
MBBR performance at different a C/N ratio, b HRT, c Nitrate loading (n = 3), d Nitrate removal (n = 3) (P < 0.05) studies with Pall ring, Kaldnes K1 and Fluidized biomedia carriers, e COD removal (n = 3) (P > 0.05) studies with Pall ring, Kaldnes K1 and Fluidized biomedia carriers, f Nitrate removal (n = 3) (P < 0.05) studies at 20%, 30% and 40% of filling ratio, g COD removal (n = 3) (P > 0.05) studies at 20%, 30% and 40% of filling ratio
MBBR performance at different HRT
Appropriate Hydraulic retention time (HRT), the length of time that nitrate remains in the bioreactor is important for the removal of nitrate. The data shown in Fig. 3b indicates that at HRT 8, 6 and 3 h nitrate removal was 100% with initial nitrate loading of 620 mg L−1 keeping the COD below permissible range. This means that at HRT 8, 6, and 3 h wastewater had sufficient contact time with the bacteria in the reactor to achieve complete nitrate reduction. Whereas at 2 h HRT nitrate removal efficiency decreased from 100 to 70%, as the contact time was insufficient to achieve complete nitrate removal. Here, HRT 3 h is the short time with maximum nitrate removal efficiency. Increasing HRT above 3 h did not influence the nitrate removal. Hence, 3 h was selected as an optimum HRT for the special seed.
MBBR performance at different nitrate loading
The nitrate concentrations in wastewater tend to fluctuate widely even in the same wastewater. Considering this the effect of input nitrate content was studied by increasing the initial nitrate concentration in synthetic wastewater from 620 to 2400 mg L−1 in different individual runs. As shown in Fig. 3c the denitrification efficiency in the MBBR was 100%, 92.25%, 93.02%, 80.43%, 72.23%, 70.45% at 620, 744, 930, 1116, 1500 and 2400 mg L−1 of NO3− concentration accompanied every time by COD reduction below permissible range i.e. 250 mg L−1. An overall result as shown in Fig. 3a–c suggests that the special microbial seed was able to reduce nitrate up to 2400 mg L−1 at optimized C/N ratio 0.3 and HRT of 3 h.
MBBR performance with carriers with different designs
Three different types of carrier designs were checked having surface area, 275 m2/m3 (Pall ring), 500 m2/m3 (Kaldnes K1), and 400 m2/m3 (Fluidized biomedia). As shown in Fig. 3d and e Pall ring carriers showed the highest nitrate removal efficiency from 620 to 2400 mg L−1 nitrate concentration and COD reduction below permissible range, while the biomass quantified from Pall ring, Kaldnes K1, and Fluidized biomedia was 35, 11.6, and 12 mg/carrier, respectively. Maximum biomass was developed on Pall ring carriers. Low biomass content on the carriers with Kaldnes K1 and Fluidized biomedia must have been possibly due to shedding of biomass upon collision of carriers with each other as the shape of carriers supported more biomass on the outer surface of the carriers. In EPS component analysis proteins were found to be most abundantly present in the biofilms obtained from all the carriers. Here, Pall ring carriers contain highest EPS components.
MBBR performance at different filling ratio
Filling fraction of carrier is also one of the key factor for performance of MBBR. The filling fractions should be below 70% for the carriers to move freely in the reactor (Ødegaard 2006). As represented in Fig. 3f and g, at 20% filling ratio highest denitrification nitrate removal efficiency was achieved in the MBBR. However, filling ratio did not influence COD reduction (Fig. 3g). As filling ratio increased from 20 to 40% denitrification efficiency was decreased and concomitantly biomass on the carrier material too decreased from 35 to 12 mg/carrier, respectively. It was also observed that 20% filling ratio allowed proper circulation of the carriers and uniform biofilm formation, while at 30% and 40% filling ratio biofilm development was scanty.
Studies on kinetics of denitrification in MBBR
Modified Stover-Kincannon model was applied to experimental results from the continuously operated MBBR for removal of nitrate from synthetic effluent and kinetic constants denitrification were determined (Derakhshan et al. 2018). Figure 4a indicates the relationship between predicted and observed effluent nitrate concentration in developed MBBR. There is a linear relationship between observed and predicted effluent nitrate concentrations with R2 = 0.981 regression coefficient indicating that kinetic constants can be used in predicting effluent nitrate concentration of developed MBBR. Figure 4b illustrates the results of plotting the model graph i.e., inverse of specific substrate removal rate Q (Si-Se)/V versus inverse of total loading rate QSi/V for different nitrate loading. The kinetic constants KB and Umax can be estimated as 17.10 mg L−1.day and 20.54 mg L−1.day, respectively from Fig. 4b. Experimental data was applied at high correlation (R2 of 0.96) to the model (Fig. 4b). This suggests that optimized MBBR was efficient for nitrate removal.
Comparative study between suspended reactor and MBBR developed with special microbial seed
Comparative nitrate removal studies between optimized MBBR developed with special microbial seed consortium and suspended reactor system in the same reactor showed that the MBBR yielded higher nitrate removal than suspended reactor (Fig. 5a and b).
Microbial community analysis of biofilm developed in optimized denitrifying MBBR
Whole genome metagenomic approach followed here gave a comprehensive characterization of microbial taxa, and metabolic pathways present in the microhabitat, which facilitated an opportunity to understand the function of MBBR in the present case. As depicted in Fig. 6a, Proteobacteria was the most abundant phylum in all the three biofilm samples collected from different locations of the MBBR. Results of this study were consistent with the cited literature where MBBR developed with activated sludge had Proteobacteria as the dominant phylum (Guo et al. 2019). Further, at genera and species level Thauera and Thauera humireducens and Thauera sp. MZ1T was the most abundant in all biofilm samples (Fig. 6b and c). Among the five members of the consortium used as seed Thauera spp. were found to be most dominant and functionally efficient in long term operated MBBR.
Microbial community analysis of continuous MBBR after 300 days. a Abundance of different phylum in biofilm, b Abundance of different genera in biofilm, c Abundance of different species in biofilm, d Correlation between different Phylum by PCA, e Correlation between different genera by PCA, f Correlation between different species by PCA, g Correlation between different genes involved in nitrogen metabolism pathways by PCA, h Circos depicting distribution of carbon, nitrogen and energy metabolism pathways present in biofilms of MBBR
Principle component analysis (PCA) of data revealed that at phylum level Cyanobacteria, Chloroflexi, Bacteroidetes, Verrucomicrobia, Firmicutes are positively correlated with each other (Fig. 6d). At genera level Pseudomonas, Thauera, Azoarcus positively correlated with each other and negatively with Paracoccus (Fig. 6e). Also, all the Thauera species are positively correlated with each other at species level (Fig. 6f). Detailed studies of genes involved in nitrogen metabolism as shown in Fig. 6g demonstrates that all the genes involved in denitrification process are positively correlated with each other, and highly abundant in the reactor implying that the potential for denitrification was well developed in the denitrifying MBBR. A relative abundance of genes involved in energy, carbohydrate, nitrogen, lipid metabolic pathways indicate that the functional capabilities were widespread in biofilms developed in MBBR (P > 0.05) (Fig. 6h).
Discussion
Due to lack of functional microorganisms, MBBR often showed poor performance and decreased pollutant removal efficiency. Li et al. (2013) suggested immobilization of specific pollutant degrading bacteria and biofilm based technology as the key to achieve proficient degradation in a bioaugmentation system. Hence, to develop cost effective and environmental friendly strategy a consortium of biofilm forming denitrifying bacteria was prepared for inoculation as a special microbial seed in MBBR. Amyloid producing bacteria possess the ability to form biofilm where these fibrous proteins are associated with functional biofilm. Also, amyloid producing bacteria are abundantly found in sludge flocs of effluent treatment plants (Larsen et al. 2007). Thus to obtain good biofilm forming bacteria, isolation of amyloid producing bacteria was carried out from different activated sludge samples on CPNA. Total five isolates were selected for their high denitrification efficiency, biofilm forming ability, amyloid production ability, lack of nitrite accumulation, and identified as Diaphorobacter sp. R4, Pannonibacter sp. V5, Thauera sp.V9, Pseudomonas sp.V11, and Thauera sp.V14. All the selected isolates namely, Diaphorobacter sp. (Chakravarthy et al. 2011), Pseudomonas stutzeri NL5a (Srinandan et al. 2011), Thauera sp. FDN-01 (Lu et al. 2019), Pannonibacter sp. (Bai et al. 2019) are known denitrifiers.
The stable coexistence of different species within a single microbial consortium is a prerequisite for the construction of a microbial consortium (Qian et al. 2020). Here, consortium of the selected five isolates showed synergistic effect to each other and showed highest nitrate removal and no nitrite accumulation which is the important attribute for the development of the special seed. Consortium of Pseudomonas sp. KW1 and Bacillus sp. YW4 (Rajakumar et al. 2008), Pseudomonas stutzeri and Pseudomonas putida (Cyplik et al. 2013), Nitrosomonas europaea and Paracoccus denitrificans (Uemoto & Saiki 2000), pure cultures of Pseudogulbenkiania 2002 and Thiobacillus denitrificans (Kiskira et al. 2017) have been reported for nitrate and nitrogen removal from wastewater, suggesting that mixed cultures or consortia are highly effective in efficient removal of nitrate from wastewater.
Process parameters required for efficient nitrate and COD removal optimized in continuous bench scale MBBR showed that the organic carbon source plays an important role in the biological denitrification process as it acts as electron donor while nitrate acts as electron acceptor. However, low C/N ratio limits the electron supply for reductive half-reactions and thereby leading to accumulation of denitrification intermediates (NO2−, NO, and N2O), which is harmful to the environment and human health. On the contrary, use of excess electron donor results in wastage of expensive electron source and increases the effluent COD (Mohan et al. 2016). Therefore in the present study 0.3 C/N ratio was selected for the reason that it did not cause secondary contamination in synthetic effluent as well as showed complete denitrification. At 3 h HRT developed MBBR showed highest (100%) nitrate removal efficiency (at initial loading of 620 mg L−1 nitrate concentration) within shortest HRT (3 h) compared to other reports in the literature that required more time to remove nitrate such as MBBR developed with Pseudomonas sp. SZF15 reported HRT of 11.96 h for removal of 47.64 mg L−1 nitrate with 79.78% removal efficiency (Su et al. 2016). It also suggests that short HRT condition can also effectively remove nitrate from wastewater.
Results obtained in this study also showed highest nitrate removal than other reports published previously by specific microorganisms such as Brevundimonas diminuta MTCC, Pseudomonas butanovora, Pseudomonas sp. SZF15 in MBBR (Kavitha et al. 2009; Kesseru et al. 2003; Su et al. 2016) as cited in Table 1. In MBBR surface area, size, and shape of carriers have a profound effect on the biofilm formed on it. Biofilm is the key parameter of MBBR performance (Ødegaard 2006). Here, pall ring carriers have more surface area inside the carriers, which prevented the biofilm biomass from sloughing off. On the contrary fluidized carriers and Kaldnes K1 carriers have more area on the outer surface, which facilitated sloughing off during collision between carriers, as a result causing reduction in overall biomass associated with the carriers. Moreover, a higher filling ratio of pall ring carriers in the MBBR showed reduction in nitrate removal due to the insufficient area for circulation of the carriers, which renders the biofilm to be formed easily and increases particle–particle collision, and enhance the shear stress on the biofilm (Wang et al. 2005). Zhang et al. (2016a, b) have also reported that at a 20% filling ratio sponge carrier achieved maximum biomass amount per gram of sponge.
In the present study, compared to suspended reactor biofilm reactor developed with special microbial seed showed better performance due to biomass accumulation and retention thereby giving higher reaction rate was observed, which is also reported by Nicolella et al. (2000). Biomass washout in suspended reactor is also a strong reason why its performance was poorer than the MBBR. The results here are similar to Falas et al. (2012) and Mazioti et al. (2015) who also demonstrated that biomass in moving bed biofilm carriers have a higher pollutant removal capacity potential than biomass in the suspended reactor system.
Long term performance of the developed MBBR demonstrates that the conditions prevalent in the developed MBBR were most suitable for Thauera spp. In literature too, it has been reported that Thauera sp. played an important role in anaerobic/anoxic/oxic reactor (Zhao et al. 2018) and denitrifying biofilter (Cui et al. 2017). This result emphasizes that Thauera spp. are playing key role in MBBR. Consequently, Thauera has emerged as an important genus in the investigation of metabolic versatility and remediation of environmental pollutants (Liu et al. 2013). Results of PCA revealed that addition of special microbial seed promoted the functionally active denitrifiers which were responsible for directly increasing the nitrate removal efficiency of the reactor system. The high abundances of genes encoding nitrate reduction and denitrification demonstrated high nitrogen metabolism potential of the denitrifying MBBR. Moreover, genes involved in reduction of nitrite to ammonium (nirA), nitric oxide reductase gene (norc) that are part of aerobic pathway were negatively correlated with genes involved in denitrification, which means that anoxic conditions were maintained in the reactor and ammonia release was absent. Overall, studies suggested that inoculation of special microbial seed of denitrifying bacterial could maintain the highly functional microorganisms in the reactor and consequently make the MBBR system more efficient for the treatment of nitrate containing wastewater. This study also suggested that special microbial seed of biofilm forming denitrifying bacteria can be used as effective strategy for bioremediation of nitrate containing wastewater as inoculation of special seeds increased the population of functional bacteria in the MBBR.
Conclusion
Biofilm forming denitrifying microbial seed of Diaphorobacter sp. R4, Pannonibacter sp. V5, Thauera sp. V9, Pseudomonas sp.V11, and Thauera sp.V14 accelerated denitrification of synthetic nitrate containing wastewater. 0.3 C/N ratio, 3 h HRT at 2400 mg L−1 Nitrate loading, 20% filling ratio in the MBBR operated with Pall ring carrier in continuously mode for 300 days gave the highest nitrate removal clearly showing that bioaugmentation combined with optimization of process parameter appreciably enhanced the reactor performance. Notably, whole genome metagenomic studies showed that Thauera spp. and Pseudomonas sp. flourished in the reactor after 300 days of operation; three out of five consortium members belonged to these two genera. The reactor was dominated by Thauera spp. genes involved in different metabolic pathways and denitrification was abundantly present in the developed biofilm indicating that the reactor was conditioned completely for denitrification. This study gave an effective suggestion that bioaugmentation of special bacterial seed comprising functional bacteria to MBBR made the system more efficient for denitrification of nitrate containing wastewater.
References
Andreou LV (2013) Preparation of genomic DNA from bacteria. Methods Enzymol 529:143–151. https://doi.org/10.1016/B978-0-12-418687-3.00011-2
APHA (1998) Standard methods for the examination of water and wastewater, 537, 20th edn. United Book Press, USA
Ashok V, Hait S (2015) Remediation of nitrate-contaminated water by solid-phase denitrification process—a review. Environ Sci Pollut Res 22:8075–8093. https://doi.org/10.1007/s11356-015-4334-9
Bai H, Liao S, Wang A, Huang J, Shu W, Ye J (2019) High-efficiency inorganic nitrogen removal by newly isolated Pannonibacter phragmitetus B1. Bioresour Technol 271:91–99. https://doi.org/10.1016/j.biortech.2018.09.090
Barber WP, Stuckey DC (2000) Nitrogen removal in a modified anaerobic baffled reaction (ABR). 1 Denitrification. Water Res 34:2413–2422. https://doi.org/10.1016/S0043-1354(99)00425-X
Berg I, Nilsson PPR, Thor S, Hammarström P (2010) Efficient imaging of amyloid deposits in Drosophilamodels of human amyloidoses. Nat Protoc 5:935–944. https://doi.org/10.1038/nprot.2010.41
Camargo JA, Alonso Á (2006) Ecological and toxicological effects of inorganic nitrogen pollution in aquatic ecosystems: a global assessment. Environ Int 32:831–849. https://doi.org/10.1016/j.envint.2006.05.002
Chakravarthy SS, Pande S, Kapoor A, Nerurkar AS (2011) Comparison of denitrification between Paracoccus sp. and Diaphorobacter sp. Appl Biochem Biotechnol 165:260–269. https://doi.org/10.1007/s12010-011-9248-5
Chapman MR, Robinson LS, Pinkner JS, Roth R, Heuser J, Hammar M, Normark S, Hultgren SJ (2002) Role of Escherichia coli curli operons in directing amyloid fiber formation. Science 295:851–855. https://doi.org/10.1126/science.1067484
Chen JP (1996) Production of ethyl butyrate using gel-entrapped Candida cylindracea lipase. J Ferment Bioeng 82:404–407. https://doi.org/10.1016/0922-338X(96)89160-4
Cui B, Liu X, Yang Q, Li J, Zhou X, Peng Y (2017) Achieving partial denitrification through control of biofilm structure during biofilm growth in denitrifying biofilter. Bioresour Technol 238:223–231. https://doi.org/10.1016/j.biortech.2017.04.034
Cyplik P, Juzwa W, Marecik R, Powierska-Czarny J, Piotrowska-Cyplik A, Czarny J, Drozdzyńska A, Chrzanowski Ł (2013) Denitrification of industrial wastewater: influence of glycerol addition on metabolic activity and community shifts in a microbial consortium. Chemosphere 93:2823–2831. https://doi.org/10.1016/j.chemosphere.2013.09.083
Derakhshan Z, Ehrampoush MH, Mahvi AH, Dehghani M, Faramarzian M, Ghaneian MT, Mokhtari M, Ebrahimi AA, Fallahzadeh H (2018) Evaluation of a moving bed biofilm reactor for simultaneous atrazine, carbon and nutrients removal from aquatic environments: modeling and optimization. J Ind Eng Chem 67:219–230. https://doi.org/10.1016/j.jiec.2018.06.032
Falås P, Baillon-Dhumez A, Andersen HR, Ledin A, La Cour JJ (2012) Suspended biofilm carrier and activated sludge removal of acidic pharmaceuticals. Water Res 46:1167–1175. https://doi.org/10.1016/j.watres.2011.12.003
Foglar L, Gašparac D (2013) Continuous-flow biological denitrification with zeolite as support for bacterial growth. Desalin Water Treat 51:7157–7165. https://doi.org/10.1080/19443994.2013.792162
Frings CS, Fendley TW, Dunn RT, Queen CA (1972) Improved determination of total serum lipids by the sulfo-phospho-vanillin reaction. Clin Chem 18:673–674. https://doi.org/10.1093/clinchem/18.7.673
Gamble TN, Betlach MR, Tiedje JM (1977) Numerically dominant denitrifying bacteria from world soils. Appl Environ Microbiol 33:926–939. https://doi.org/10.1128/aem.33.4.926-939.1977
García-Martínez Y, Chirinos J, Bengoa C, Stüber F, Font J, Fortuny A, Fabregat A (2017) Nitrate removal in an innovative up-flow stirred packed-bed bioreactor. Chem Eng Process Process Intensif 121:57–64. https://doi.org/10.1016/j.cep.2017.08.001
Gebbink MFBG, Claessen D, Bouma B, Dijkhuizen L, Wösten HAB (2005) Amyloids—a functional coat for microorganisms. Nat Rev Microbiol 3:333–341. https://doi.org/10.1038/nrmicro1127
Gong L, Huo M, Yang Q, Li J, Ma B, Zhu R, Wang S, Peng Y (2013) Performance of heterotrophic partial denitrification under feast-famine condition of electron donor: a case study using acetate as external carbon source. Bioresour Technol 133:263–269. https://doi.org/10.1016/j.biortech.2012.12.108
Guo X, Li B, Zhao R, Zhang J, Lin L, Zhang G, Li RH, Liu J, Li P, Li Y, Li XY (2019) Performance and bacterial community of moving bed biofilm reactors with various biocarriers treating primary wastewater effluent with a low organic strength and low C/N ratio. Bioresour Technol. https://doi.org/10.1016/j.biortech.2019.121424
Hong P, Wu X, Shu Y, Wang C, Tian C, Wu H, Xiao B (2020) Bioaugmentation treatment of nitrogen-rich wastewater with a denitrifier with biofilm-formation and nitrogen-removal capacities in a sequencing batch biofilm reactor. Bioresour Technol 303:122905. https://doi.org/10.1016/j.biortech.2020.122905
Islas-Lima S, Thalasso F, Gómez-Hernandez J (2004) Evidence of anoxic methane oxidation coupled to denitrification. Water Res 38:13–16. https://doi.org/10.1016/j.watres.2003.08.024
Kavitha S, Selvakumar R, Sathishkumar M, Swaminathan K, Lakshmanaperumalsamy P, Singh A, Jain SK (2009) Nitrate removal using Brevundimonas diminuta MTCC 8486 from ground water. Water Sci Technol 60:517–524. https://doi.org/10.2166/wst.2009.378
Kesserü P, Kiss I, Bihari Z, Polyák B (2003) Biological denitrification in a continuous-flow pilot bioreactor containing immobilized Pseudomonas butanovora cells. Bioresour Technol 87:75–80. https://doi.org/10.1016/S0960-8524(02)00209-2
Kiskira K, Papirio S, van Hullebusch ED, Esposito G (2017) Influence of pH, EDTA/Fe(II) ratio, and microbial culture on Fe(II)-mediated autotrophic denitrification. Environ Sci Pollut Res 24:21323–21333. https://doi.org/10.1007/s11356-017-9736-4
Krishna Mohan TV, Nancharaiah YV, Venugopalan VP, Satya Sai PM (2016) Effect of C/N ratio on denitrification of high-strength nitrate wastewater in anoxic granular sludge sequencing batch reactors. Ecol Eng 91:441–448. https://doi.org/10.1016/j.ecoleng.2016.02.033
Larsen P, Nielsen JL, Dueholm MS, Wetzel R, Otzen D, Nielsen PH (2007) Amyloid adhesins are abundant in natural biofilms. Environ Microbiol 9:3077–3090. https://doi.org/10.1111/j.1462-2920.2007.01418.x
Leyva-Díaz JC, Martín-Pascual J, Poyatos JM (2017) Moving bed biofilm reactor to treat wastewater. Int J Environ Sci Technol 14:881–910. https://doi.org/10.1007/s13762-016-1169-y
Li C, Li Y, Cheng X, Feng L, Xi C, Zhang Y (2013) Immobilization of Rhodococcus rhodochrous BX2 (an acetonitrile-degrading bacterium) with biofilm-forming bacteria for wastewater treatment. Bioresour Technol 131:390–396. https://doi.org/10.1016/j.biortech.2012.12.140
Li C, Yue Z, Feng F, Xi C, Zang H, An X, Liu K (2016) A novel strategy for acetonitrile wastewater treatment by using a recombinant bacterium with biofilm-forming and nitrile-degrading capability. Chemosphere 161:224–232. https://doi.org/10.1016/j.chemosphere.2016.07.019
Liu B, Frostegård Å, Shapleigh JP (2013) Draft genome sequences of five strains in the genus Thauera. Genome Announc 1:1–2. https://doi.org/10.1128/genomeA.00052-12
Liu X, Wang L, Pang L (2018) Application of a novel strain Corynebacterium pollutisoli SPH6 to improve nitrogen removal in an anaerobic/aerobic-moving bed biofilm reactor (A/O-MBBR). Bioresour Technol 269:113–120. https://doi.org/10.1016/j.biortech.2018.08.076
Lu L, Wang B, Zhang Y, Xia L, An D, Li H (2019) Identification and nitrogen removal characteristics of Thauera sp. FDN-01 and application in sequencing batch biofilm reactor. Sci Total Environ 690:61–69. https://doi.org/10.1016/j.scitotenv.2019.06.453
Masuko T, Minami A, Iwasaki N, Majima T, Nishimura SI, Lee YC (2005) Carbohydrate analysis by a phenol-sulfuric acid method in microplate format. Anal Biochem 339:69–72. https://doi.org/10.1016/j.ab.2004.12.001
Mazioti AA, Stasinakis AS, Pantazi Y, Andersen HR (2015) Biodegradation of benzotriazoles and hydroxy-benzothiazole in wastewater by activated sludge and moving bed biofilm reactor systems. Bioresour Technol 192:627–635. https://doi.org/10.1016/j.biortech.2015.06.035
Nicolella C, Van Loosdrecht MCM, Heijnen JJ (2000) Wastewater treatment with particulate biofilm reactors. J Biotechnol 80:1–33. https://doi.org/10.1016/S0168-1656(00)00229-7
Ødegaard H (2006) Innovations in wastewater treatment: the moving bed biofilm process. Water Sci Technol 53:17–33. https://doi.org/10.2166/wst.2006.284
Qambrani NA, Jung SH, Ok YS, Kim YS, Oh SE (2013) Nitrate-contaminated groundwater remediation by combined autotrophic and heterotrophic denitrification for sulfate and pH control: batch tests. Environ Sci Pollut Res 20:9084–9091. https://doi.org/10.1007/s11356-013-1623-z
Qian X, Chen L, Sui Y, Chen C, Zhang W, Zhou J, Dong W, Jiang M, Xin F, Ochsenreither K (2020) Biotechnological potential and applications of microbial consortia. Biotechnol Adv 40:107500. https://doi.org/10.1016/j.biotechadv.2019.107500
Rajakumar S, Ayyasamy PM, Shanthi K, Thavamani P, Velmurugan P, Song YC, Lakshmanaperumalsamy P (2008) Nitrate removal efficiency of bacterial consortium (Pseudomonas sp. KW1 and Bacillus sp. YW4) in synthetic nitrate-rich water. J Hazard Mater 157:553–563. https://doi.org/10.1016/j.jhazmat.2008.01.020
Skold A, Cosco DL, Klein R (2011) Methemoglobinemia: pathogenesis, diagnosis, and management. South Med J 104:757–761. https://doi.org/10.1097/SMJ.0b013e318232139f
Srinandan CS, Jadav V, Cecilia D, Nerurkar AS (2010) Nutrients determine the spatial architecture of Paracoccus sp. biofilm. Biofouling 26:449–459. https://doi.org/10.1080/08927011003739760
Srinandan CS, Shah M, Patel B, Nerurkar AS (2011) Assessment of denitrifying bacterial composition in activated sludge. Bioresour Technol 102:9481–9489. https://doi.org/10.1016/j.biortech.2011.07.094
Su J, Fan Y, Huang T, Wei L, Gao C (2020) Modified PVA (polyvinyl alcohol) biomaterials as carriers for simultaneous removal of nitrate, Cd(II), and Mn(II): performance and microbial community. Environ Sci Pollut Res 27:28348–28359. https://doi.org/10.1007/s11356-020-09114-3
Su JF, Luo XX, Wei L, Ma F, Zheng SC, Shao SC (2016) Performance and microbial communities of Mn(II)-based autotrophic denitrification in a Moving Bed Biofilm Reactor (MBBR). Bioresour Technol 211:743–750. https://doi.org/10.1016/j.biortech.2016.03.101
Suhr KI, Pedersen PB, Arvin E (2013) End-of-pipe denitrification using RAS effluent waste streams: effect of C/N-ratio and hydraulic retention time. Aquacult Eng 53:57–64. https://doi.org/10.1016/j.aquaeng.2012.11.005
Uemoto H, Saiki H (2000) Distribution of Nitrosomonas europaea and Paracoccus denitrificans immobilized in tubular polymeric gel for nitrogen removal. Appl Environ Microbiol 66:816–819. https://doi.org/10.1128/AEM.66.2.816-819.2000
Wang RC, Wen XH, Qian Y (2005) Influence of carrier concentration on the performance and microbial characteristics of a suspended carrier biofilm reactor. Process Biochem 40:2992–3001. https://doi.org/10.1016/j.procbio.2005.02.024
Zhang Q, Ji F, Xu X (2016a) Optimization of nitrate removal from wastewater with a low C/N ratio using solid-phase denitrification. Environ Sci Pollut Res 23:698–708. https://doi.org/10.1007/s11356-015-5308-7
Zhang X, Chen X, Zhang C, Wen H, Guo W, Ngo HH (2016b) Effect of filling fraction on the performance of sponge-based moving bed biofilm reactor. Bioresour Technol 219:762–767. https://doi.org/10.1016/j.biortech.2016.08.031
Zhang H, Li S, Ma B, Huang T, Qiu H, Zhao Z, Huang X, Liu K (2020) Nitrate removal characteristics and 13C metabolic pathways of aerobic denitrifying bacterium Paracoccus denitrificans Z195. Bioresour Technol 307:123230. https://doi.org/10.1016/j.biortech.2020.123230
Zhao B, Cheng DY, Tan P, An Q, Guo JS (2018) Characterization of an aerobic denitrifier Pseudomonas stutzeri strain XL-2 to achieve efficient nitrate removal. Bioresour Technol 250:564–573. https://doi.org/10.1016/j.biortech.2017.11.038
Acknowledgements
This work was supported by the Department of Biotechnology (DBT), (Grant No. BT/PR15531/BCE/8/1156/2016) GOI for fellowship, and Department of Science and Technology, GOI, FIST programme for instrumentation facility.
Funding
The authors acknowledge the Department of Biotechnology (DBT), (Grant No.BT/PR15531/BCE/8/1156/2016) GOI for fellowship, and Department of Science and Technology, GOI, FIST programme for instrumentation facility.
Author information
Authors and Affiliations
Contributions
Conceptualization & design of experiments—RJP & ASN, Performance of experiments—RJP, Design of Reactor—UDP, Reagents/Materials/Equipments—ASN, Manuscript writing, analysis & discussion—RJP & ASN.
Corresponding author
Ethics declarations
Conflict of interest
The authors have no conflicts of interest to declare that are relevant to the content of this article.
Additional information
Publisher's Note
Springer Nature remains neutral with regard to jurisdictional claims in published maps and institutional affiliations.
Supplementary Information
Below is the link to the electronic supplementary material.
Rights and permissions
About this article
Cite this article
Patel, R.J., Patel, U.D. & Nerurkar, A.S. Moving bed biofilm reactor developed with special microbial seed for denitrification of high nitrate containing wastewater. World J Microbiol Biotechnol 37, 68 (2021). https://doi.org/10.1007/s11274-021-03035-0
Received:
Accepted:
Published:
DOI: https://doi.org/10.1007/s11274-021-03035-0