Abstract
Toona sinensis (Juss.) M.Roem., is an economically important seasonable vegetable tree with a wide range of nutritional, medicinal, and industrial values. The existing breeding methods can no longer sustainably meet the growing and annual production demands for T. sinensis buds. An efficient and cost-effective in vitro large-scale micropropagation procedure for T. sinensis was developed from shoot stems with axillary or terminal buds through the processes of callus elimination, multiple shoot induction and hydroponic acclimation of the regenerated plantlets. Plant growth regulators, explant type, explant age and activated charcoal (AC) play an important role in multiple shoot induction and callus reduction. The highest shoot induction frequency (97.8%) with a mean number of shoots equal to 7.6 and a relatively smaller callus (0.3 cm in diameter) was obtained from the in vitro culture of 75-day-old shoot stems with axillary buds in Murashige and Skoog (MS) medium augmented with 1.0 mg l−1 N6-benzyladenine (6-BA) and 0.2 mg l−1 indole-3-acetic acid (IAA). In addition, a complete plantlet with strong roots and mature leaves could be obtained directly by culturing the shoot stem segment on medium containing at least 0.5 g l−1 AC, which reveals a new direction for the industrialized propagation of T. sinensis. Among the PGRs tested, a low concentration (0.5 mg l−1) of zeatin (ZT) was found to be the most conducive to shoot elongation. We also found that low concentrations of auxin, activated carbon, sucrose and a low macronutrient strength are more beneficial for in vitro adventitious root formation. The highest rooting response (100%) with 5.7 roots produced per shoot was achieved in 1/4 MS medium with the addition of 0.5 mg l−1 potassium salt of indole butyric acid (K-IBA), 0.5 g l−1 AC, and 10.0 g l−1 sucrose. In addition, the major challenge of the low transplanting survival rate of regenerated T. sinensis plants was solved by adopting an ex-vitro hydroponic domestication strategy in a mini-incubator. The plants were successfully hardened in a transplanting mixture of garden soil, perlite, vermiculite and Pindstrup substrate (3:1:1:3), and the transplanting survival rate was 95.3%. Inter simple sequence repeat (ISSR) assessment revealed no difference between the regenerated plants and the mother plants. The micropropagation protocol established in this study will lay the foundation for germplasm conservation of T. sinensis and its sustainable utilization in food.
Key message
An efficient and genetically stable in vitro large-scale micropropagation procedure for Toona sinensis was developed from shoot stem with through multiple shoot induction and hydroponic domestication.
Similar content being viewed by others
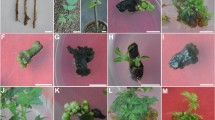
Avoid common mistakes on your manuscript.
Introduction
Toona sinensis Roem. (Cedrela sinensis), also known as Chinese toon, belongs to the family Meliaceae and is an economically important vegetable tree with great nutritional and medicinal value (Qin et al. 2006). This fast-growing perennial tree is native to East Asia and Southeast Asia (Liao et al. 2009; Sun et al. 2016b) and is widely cultivated in China, India, Korea, Taiwan, and Malaysia (Chih-Yao et al. 2012; Yu et al. 2012). This plant species have been cultivated for more than 2300 years (Chen et al. 2008) and serves as an important source of seasonal vegetables and traditional Chinese medicine in China (Liao et al. 2007). Fresh tender sprouts of T. sinensis are very popular as a nutritious vegetable in the Chinese diet due to their unique aromatic flavor, therapeutic value, and abundant contents of vitamins, minerals and antioxidants (Wang et al. 2021; Zhang et al. 2014). In recent years, T. sinensis has once again attracted the attention of the world for its high edible value mentioned above.
In addition to its edible function, T. sinensis is famous for its traditional medicinal value in China, and almost all of its organs can be used for medicine (Chen et al. 2017; Luo et al. 2000). Its leaves are employed to treat gastric ulcers, rheumatoid arthritis, and itchiness (Kakumu et al. 2014; Yang et al. 2020). Its seeds are used for the treatment of gastritis, duodenal ulcer, and headache (Dong et al. 2013), and its bark is used for the treatment of blood and skin diseases. Further phytochemical investigations of T. sinensis have shown that the active components of the extract were mainly terpenoids, flavonoids, polyphenols, alkaloids, saponin steroids, polysaccharides, and volatile oil (Kakumu et al. 2014; Mu et al. 2007; Shi et al. 2017). The extract of T. sinensis has a variety of pharmacological activities, such as anticancer (Wei et al. 2014; Yang et al. 2020), antioxidation (Chen et al. 2013; Jiang et al. 2019), anti-aging (Li et al. 2017) and anti-inflammation (Hsiang et al. 2013; Kuo et al. 2020) activities. Moreover, due to the high density and dark color of its wood, the trunk of T. sinensis is considered a good source of hardwood that can be used in furniture making (Yousheng and Sziklai 1985).
Given the abovementioned industrial characteristics and the potential global economic importance of T. sinensis, the interest in this plant species has been growing in recent years. At present, the research on T. sinensis mainly focuses on its effective ingredients (Cao et al. 2019), pharmacological values (Su et al. 2020), composition of odorants (Ren et al. 2021; Yang et al. 2019), and postharvest quality (Lin et al. 2019). Recently, the large-scale cultivation of T. sinensis has been increasingly encouraged by the Chinese government. However, although T. sinensis has a long history of cultivation in China, it remains in a “wild” state. This species is usually planted sporadically in the front and back of houses, and there is a lack of systematic research on breeding technology. In addition, great differences in taste, active components, and other agronomic traits of T. sinensis are found among different lines in different regions, which would affect its long-term domestication and extensive cultivation (Su et al. 2020). Therefore, the selection of excellent T. sinensis lines with regional characteristics as planting materials for raising commercial plantations can ensure higher economic and social benefits.
Conventionally, T. sinensis is propagated mainly through seeds. However, the low germination rate caused by low seed vigor is the primary factor hampering the natural propagation of this tree (Li et al. 2011). In addition, the seedlings obtained by seed propagation exhibit high heterozygosity, which cannot maintain the agronomic characteristics of the selected genotypes. Moreover, T. sinensis can also be propagated by cuttings, but the limitations of the industrialized application of this technology lie in the availability of materials, the limitation of season, and the difficulty of adventitious root induction (Bingkun et al. 2002). In addition, another important problem in the production of T. sinensis seedlings by the natural reproduction method is that as a seasonal vegetable, the tender sprouts grow in early spring, and the supply period is only approximately three weeks. The leaves then become older and fibrous, the aroma intensity decreases, and the toxicologically relevant compounds (nitrites) increase, which makes the species no longer suitable for consumption (Yang et al. 2019). To a certain extent, this phenomenon severely reduces the commercial and nutritional values of Chinese toon buds. Therefore, the traditional breeding methods mentioned above can no longer meet the growing demand of T. sinensis seedlings in the nutraceutical, food, and pharmaceutical industries. As modern biotechnology, plant tissue culture technology has been widely applied in the commercial propagation of many important tree species because of its advantages of high regeneration efficiency and no restriction of season and materials, but its application to T. sinensis is very limited. In recent years, researchers have tried to establish a regeneration system for T. sinensis by using leaves (Liqiong and Bingkun 2007) and nodal stems (Qin et al. 2006) as explants. However, for leaf regeneration, shoots are obtained through direct shoot organogenesis, which is not ideal for the production of genetically selected plantlets (Liqiong and Bingkun 2007). For nodal stem regeneration, the proliferation coefficient, rooting frequency, and survival rate remain very low (Qin et al. 2006). In addition, during the whole regeneration process, many calli are inevitably formed at the base of explants on different culture media, and the shoots obtained were seriously vitrified (Kang et al. 2002; Qin et al. 2006). Tissue culture-mediated in vitro micropropagation technology can be applied on a large scale only under the premise of a low cost, and the cost-effectiveness depends on the propagation efficiency and the survival rate of acclimatized regenerated plants. The survival of regenerated plantlets is affected by many environmental conditions, and it is very important to ensure proper domestication before transplanting into the field. Different domestication methods affect the survival rate of regenerated plants, and among those protocols, hydroponic acclimation system is a promising method for improving the survival rate of regenerated plants of many plant species. For example, the hydroponic acclimation could greatly improve the survival rate of regenerated apple plants (Xie et al. 2015) and grape plants (Cai et al. 2019). However, to our knowledge, the domestication of regenerated plantlets of T. sinensis using hydroponic technology has never been reported. In addition, the major constraint of the tissue culture technique is that it is impossible to completely exclude the possibility of in vitro regenerated plant variation (Kaeppler et al. 2000). Although these variations may contribute to the improvement of plant lines, they are undesirable for the preservation of selected superior genotypes (Oh et al. 2007). In the developed genetic stability detection techniques, inter simple sequence repeat (ISSR) is highly praised for its simple operation, strong universality, and stable amplification products. Hence, considering the abovementioned problems in the commercial cultivation and production of T. sinensis, the purpose of the present study was to develop an efficient, stable, and repeatable micropropagation scheme for T. sinensis through direct shoot organogenesis, hydroponic domestication and ISSR genetic stability analysis, which would be of great significance for the germplasm conservation and commercial utilization of this important plant species without any seasonal constraint.
Materials and methods
Material collection, sterilization, and shoot initiation
Fresh shoot segments were collected from a mature (8-year-old) and healthy T. sinensis tree (known as heiyouchun in China) growing in Taihe City (N33°16′, E115°62′), Anhui Province, China in April 2019 (Fig. 1A). After removing the leaves, the excised nodal segments were continuously rinsed under running tap water for 30 min to wash out the adhesive materials. Then, the nodal stems were placed into a sterile container and sterilized in 75% (v/v) ethanol solution for 30 s in a clean cabinet (Suzhou Fuqiang Purification Engineering Co. Ltd., China). The explants were transferred into 0.1% (w/v) HgCl2 solution for disinfection, and the disinfection time was 3 min. Lastly, the explants were washed with sterile distilled water 6 times. The ends of the sterilized stems that were damaged by the disinfectant were cut off, and the remaining nodal stem segments (approximately 2 cm in length) were used as initial explants. The explants were cultured vertically in Murashige and Skoog (MS) (1962) medium augmented with 0.5 mg l−1 6-benzyl adenine (6-BA), 30.0 g l−1 sucrose, and 6.5 g l−1 agar for shoot initiation and germination.
In vitro shoot initiation from in vivo nodal stems of T. sinensis. A Fresh shoot segments were collected from a mature (8-year-old) and healthy T. sinensis tree in April. B The surface-sterilized in vivo shoot stems with axillary buds in the induction medium. C Shoot induction from in vivo shoot stems with axillary buds on the MS basal medium with 0.5 mg l−1 6-BA for 4 weeks. D The germinated shoot buds were excised and cultured on the MS basal medium with 0.5 mg l−1 6-BA to acquire enough materials. E Adventitious shoots regenerated from the germinated shoots after 4 weeks of culture and the shoot stems with axillary buds or apical buds from these regenerated shoots were used as in vitro explants for the further micropropagation of T. sinensis (Bars = 1.0 cm)
Explant growth conditions
Unless otherwise specified, all media used in the present study were MS medium (1962) supplemented with 30.0 g l−1 sucrose and 6.5 g l−1 agar, and the pH value was fixed to 5.8 before autoclaving. Gibberellin (GA3), indole-3-acetic acid (IAA), and zeatin (ZT) were sterilized by a filter membrane (0.22 µm) before being added to the cooled autoclaved media (approximately 50 °C), and other plant growth regulators (PGRs) were added before autoclaving. All explants were cultured in a constant temperature culture room with a temperature of 25 ± 2 °C, a photoperiod of 14 h light/10 h dark, and a light intensity of 50 μmol s−1 m−2.
Shoot induction and callus removal
For shoot induction, the in vitro 30-day-old stem segments with axillary buds or terminal buds that regenerated from in vivo shoot stems of T. sinensis were vertically cultivated in MS medium supplemented with 0.2–5.0 mg l−1 kinetin (KT), thidiazuron (TDZ), ZT, and 6-BA, respectively. Medium devoid of PGRs were served as control. To induce multiple shoots effectively, the in vitro 30-day-old nodal stems were also transferred into medium supplemented with various concentrations of 6-BA (0.5, 1.0, or 3.0 mg l−1) in combination with low concentrations (0.1–0.5 mg l−1) of indole-3-butyric acid (IBA), 1-naphthalene acetic acid (NAA), or IAA. In addition, to reduce the callus that induced at the base of shoot stems during shoot induction, the effects of explant age (30, 45, 60, 75, and 90 d) and activated charcoal (AC) content (0.0, 0.1, 0.25, 0.5, 1.0, and 1.5 g l−1) on shoot induction were also examined. After 4 weeks of cultivation, the induction responses of shoots and calli were recorded.
Proliferation and elongation of shoots
To further realize the proliferation of shoots, the shoot clusters were transferred into fresh optimum shoot induction medium for 3 weeks. Single shoots (approximately 1.1 cm in height) were then isolated from the proliferated shoot clusters and cultured in elongation medium containing 0.2–1.0 mg l−1 ZT or different combinations of GA3 (0.2 and 0.5 mg l−1) and 6-BA (0.0, 0.5 and 1.0 mg l−1). Three weeks later, the average shoot length was measured, and the elongated shoots were used for further shoot propagation and rooting.
In vitro rooting
To induce rooting effectively, elongated shoots with at least two expanded compound leaves were cut off at the shoot base (approximately 0.5 cm) and cultivated in 1/2 MS medium fortified with 0.0–2.0 mg l−1 IBA, NAA, IAA, or potassium salt of indole-3-butyric acid (K-IBA). To reduce the rooting cost, the effects of the sucrose content (0.0, 5.0, 10.0, 15.0, 20.0, 25.0, and 30.0 g l−1) and the macronutrient strength of MS medium (1, 1/2, 1/4, 1/8, 1/16, and 0 MS) on rooting of T. sinensis were studied. Four weeks later, the rooting responses were calculated.
Domestication and transplanting of the regenerated plantlets
For further domestication of the rooted plantlets of T. sinensis, the culture vessels that contained regenerated plantlets were transferred to a conventional greenhouse for one week. The relative humidity of the greenhouse was 80% and the temperature was 20–28 °C. During this period, the relative humidity in the culture vessels was slowly reduced by loosening the caps of culture vessels. Then, the well-rooted plantlets with actively growing shoots were taken out from the vessels and rinsed thoroughly. To examine the effect of the composition and ratio of the planting substratum on the survival of the plantlets, the washed plants were transplanted into seedling bags containing various types of nutrient matrix viz. garden soil, perlite, vermiculite, and Pindstrup substrate at variable proportions. Before transplantation, the planting substratum was premoistened with tap water and sterilized with 0.3% (w/v) KMnO4 solution. The in vitro regenerated plants in the seedling bags were covered with shading nets and placed in the abovementioned conventional greenhouse for further acclimatization and growth. The plantlets were observed regularly and watered at four-day intervals. In addition, to increase the survival chance of the transplanted plantlets, the transplanted plants were microirrigated with 1/4 MS macronutrients (approximately 0.2 l m−2) every 2 weeks. The experiments were implemented in three replications and each experiment included at least 300 plantlets. Four weeks after transplantation, the sun-shading net was removed, and the survival rate was calculated after 2 weeks of continuous domestication.
To further improve the survival rate of T. sinensis, the effects of three domestication methods on the transplantation of in vitro regenerated plants were examined. Domestication method I: The washed plants were transplanted to seedling bags filled with the abovementioned optimal nutrient matrix, and the other steps of domestication were the same as above. The survival rate was recorded after 6 weeks of domestication. Domestication method II: The washed plants were transplanted into plastic seedling trays filled with the same nutrient matrix used in domestication method I. The trays were covered with transparent plastic lids for initial domestication in a culture room (approximately 80% relative humidity) at 22–25 °C. The transparent plastic lids were progressively opened and removed 2 weeks later. The plantlets were then transplanted into nursery bags and cultured in the conventional greenhouse used in domestication method I. The survival rate was recorded after 4 weeks of continuous domestication. Domestication method III: The washed plantlets were fixed with a sponge and placed in the small holes of the foam board in the hydroponic trays, which contained half-strength Hoagland nutrient solution (Griffin et al. 1976). In the process of transplantation, the root system of the plantlet should be fully extended in the nutrient solution and be placed in directly contact with the nutrient solution to promote the absorption of nutrients. The hydroponic trays were covered with transparent plastic covers for initial domestication in the culture room used in domestication method II. The nutrient solution was supplemented once a week to maintain adequate nutrition and the transparent plastic cover was progressively opened and removed after 2 weeks of domestication. The plants were then transplanted into seedling bags and cultured in the conventional greenhouse used in domestication method I. The survival rate was recorded after 4 weeks of continuous domestication. The experiments were implemented in three replications and each experiment included at least 300 plantlets.
DNA isolation and genetic fidelity analysis
The genetic uniformity of regenerated plantlets was detected by ISSR molecular markers. Total genomic DNA was extracted from young leaves of 10 regenerated plantlets and mother plants using an HP Total Plant DNA Kit (Omega, Shanghai, China). Using the DNA of the mother plant as the template, 40 ISSR primers were preliminarily screened from the 100 ISSR primer sequences designed by the University of British Columbia in Canada. Among these screened primers, 8 primers with clear amplification bands and good polymorphism were selected and used for the final genetic stability analysis.
Touch-down PCR amplification was used for sequence amplification, and the program was set as follows: preheating at 94 °C for 5 min for one cycle; 24 cycles of amplification at 94 °C for 45 s, an annealing step at an initial temperature of 58 °C that was progressively lowered by − 0.5 °C /cycle and 72 °C for 60 s. This step was followed by 72 °C for 2 min, and finally stored in the 4 °C. PCR amplifications were performed in 20 μl reaction mixtures comprising 2.0 µl of 10× Taq Buffer, 1.0 µl of DNA (20 ng), 2 µl of MgCl2, 1.5 µl of Primer, 1.6 µl of dNTP, 11.6 µl of ddH2O and 0.3 µl of EasyTaq® DNA polymerase in a thermocycler. The amplified products were separated with 2.0% (w/v) agarose gels using 1× TAE (Tris–acetate EDTA) buffer at 120 V for 60 min. The molecular weights of the amplified products were estimated according to a DNA molecular marker (TransGen Biotech, Trans2K® DNA Marker), and the gel images were analyzed using a gel imaging system (Bio-Rad, USA). Under the same PCR conditions, at least three reactions were completed to confirm the reliability of the polymorphism results.
Statistical analysis
All treatments in this study were performed in a completely randomized design (CRD) and implemented in three replicate experiments with at least 30 shoots per experiment. Unless mentioned otherwise, each replication included at least 30 explants. Origin 8.5 software (Origin Lab, USA) was used to analyze the data by analysis of variance (ANOVA) with Tukey’s test. The statistical differences among the means value were distinguished at P < 0.05.
Results and discussion
Shoot initiation
The literature on T. sinensis indicates that abnormal phenomena, such as serious callus formation at the base of explants and vitrification of regenerated plants, eventually lead to low regeneration and survival rates (Kang et al. 2002; Liqiong and Bingkun 2007). The selection of an effective explant type is the basic requirement of any plant regeneration scheme. In many woody plant species, micropropagation from different parts of in vitro regenerated plantlets has received great attention. It is well known that shoot stems with bud primordia are the preferred material for successful micropropagation because their pre-existing meristem can easily develop into new buds and maintain the genetic stability of the donor plant. In this study, in vitro shoot stems with axillary buds or terminal buds that regenerated from in vivo nodal stems were used as explants for the micropropagation of T. sinensis.
For shoot initiation, the in vivo sterilized nodal stems were used as primary explants and inoculated in MS medium supplemented with 0.5 mg l−1 6-BA (Fig. 1B). Two weeks later, the axillary buds germinated, and shoots with dark green leaves were obtained after 4 weeks of cultivation (Fig. 1C). Due to the limited number of explants available for shoot initiation, the germinated shoot buds were excised and subcultured to acquire enough materials for further shoot induction and multiplication (Fig. 1D). After 4 weeks of cultivation, the shoot stems with axillary buds or apical buds from these regenerated shoots were used as in vitro explants for the further micropropagation of T. sinensis (Fig. 1E).
In vitro morphogenesis of shoot stems
Multiple shoot induction
Cytokinin is the main hormone involved in the initiation of shoot stem cells and the establishment of shoot apical meristem (SAM) (Pernisova et al. 2018; Wybouw and De Rybel 2019), and exogenous cytokinin has been used to induce multiple shoots from shoot stems of many woody plant species. The optimal type and concentration of cytokinin that promote shoot regeneration and multiplication vary among plant species. To effectively induce shoot regeneration, the in vitro stem segments with axillary buds were cultivated in medium containing different cytokinins (Table 1). The results revealed that the cytokinin in the medium had an important effect on multiple shoot induction, but the effects of different kinds and concentrations of cytokinin varied (Table 1). For the explants cultivated in medium with a low concentration (≤ 1.0 mg l−1) of KT, the axillary buds of the explants germinated, and almost no multiple shoots or calli were induced after 4 weeks of cultivation. The results were consistent with the medium devoid of PGRs (Fig. 2A). The difference was that the low concentration of KT could shorten the germination time of the existing bud primordia and improve the germination efficiency (Fig. 2B), and a few explants induced adventitious roots at the base. When the explants were inoculated in medium containing TDZ, the results showed that TDZ could inhibit the germination of the existing bud primordia and the induction of multiple shoots (Table 1). A higher concentration (above 0.5 mg l−1) of TDZ led to abnormal swelling of the explants (Table 1). The inhibition of axillary bud germination and multiple shoot formation by TDZ may be mainly due to the dual effects of auxin and cytokinin (Singh et al. 2003). In contrast, a low concentration of ZT in the medium could promote the germination and elongation of the existing bud primordia, but could not promote the formation of multiple shoots (Table 1). A high concentration (not less than 1.0 mg l−1) of ZT promoted the formation of many green calli at the base of explants, and this effect was accompanied by germination of the existing bud primordia, but these buds were vitrified seriously (Table 1). Of the four types of cytokinin examined, 6-BA was proven to be more beneficial for bud germination and shoot regeneration (Tables 1 and 2). The superiority of 6-BA over other cytokinins in shoot induction from nodal explants also has also been observed in several other woody plants, including Citrus jambhiri Lush (Savita et al. 2012), Sapium sebiferum Roxb (Hou et al. 2020), and Bauhinia racemose Lam (Sharma et al. 2017). In addition, the formation of callus at the base of shoot stems was also observed in medium supplemented with 6-BA, and the callus diameter was positively correlated with the concentration of 6-BA (Tables 1 and 2).
Shoot organogenesis from in vitro shoot stems of T. sinensis. A, B Shoot induction from in vitro 30-day-old shoot stem with axillary bud on MS medium A without PGRs or B with 0.5 mg l−1 KT after 4 weeks of culture. C, D Multiple shoot induction from in vitro 30-day-old shoot stem with C axillary bud or D terminal bud on MS medium containing 3.0 mg l−1 6-BA after 4 weeks of culture. E–I Multiple shoot induction from in vitro E 30-day-old, F 45-day-old, G 60-day-old, H 75-day-old and I 90-day-old shoot stem with axillary bud on medium fortified with 1.0 mg l−1 6-BA and 0.2 mg l−1 IAA after 4 weeks of culture, respectively. J Completed plantlets were directly obtained from a 30-day-old shoot stem with axillary bud on the MS medium containing 1.0 mg l−1 6-BA, 0.2 mg l−1 IAA and 0.5 g l−1 AC after 4 weeks of culture. K Shoot multiplication on medium supplemented with 1.0 mg l−1 6-BA and 0.2 mg l−1 IAA after 3 weeks of culture. L Elongated shoots were obtained on MS medium containing 0.5 mg l−1 ZT after 3 weeks of culture. M–N In vitro rooting of the elongated shoot on 1/4 MS medium containing 0.5 mg l−1 K-IBA and 1.0% sucrose without (M) or with (N) 0.5 g l−1 AC. O The regenerated plantlet was domesticated by the hydroponic system for 2 weeks before transplanting into the nursery bag. P The regenerated plantlets grown in the greenhouse after 6 weeks of domestication (Bars = 1.0 cm)
During the process of shoot induction of T. sinensis, we also found that the explant type affected shoot regeneration. The shoot stems with terminal buds produced more shoots than those with axillary buds on the same medium under the same culture conditions (Tables 1 and 2). In medium containing 3.0 mg l−1 6-BA, the shoot induction effect of the two explants was the best, and the shoot induction rate was 100%. The average number of shoots produced per stem segment with axillary buds was 4.9, and the average number of shoots per stem segment with terminal buds was 5.7, but those shoots exhibited slight vitrification (Tables 1 and 2, Fig. 2C, D). Severely vitrified dwarf shoots were induced from two types of shoot stems cultured in medium containing a concentration of 6-BA (5.0 mg l−1) higher than the optimum level. Similar findings were also been observed in the propagation of Lucas aspera Spreng by Vijendra et al. (2017). Vitrification is a common physiological obstacle in the process of in vitro vegetative propagation, which seriously limits the growth and survival of regenerated plantlets. We speculate that the imbalance of endogenous cytokinins caused by the oversupply of exogenous cytokinins may be the cause of this phenomenon in the process of micropropagation of T. sinensis. In the present study, we found that 6-BA was a crucial growth regulator in the regulation of bud primordium germination and multiple shoot induction from shoot stems of T. sinensis. This result is consistent with that obtained for cytokinin in overcoming apical dominance, stimulating dormant meristems to release multiple buds, and promoting shoot formation (Mali and Chavan 2016; Sharma et al. 2017).
It has been reported that in the process of multiple shoot induction, the combination of auxin and cytokinin at an appropriate proportion can better induce the shoot regeneration than cytokinin alone (Su et al. 2011). To improve the number and quality of multiple shoots, we further studied the effects of the combinations of 6-BA and auxins (NAA, IBA, and IAA) on multiple shoot induction from in vitro stems with axillary buds of T. sinensis. The results revealed that the combination of 6-BA and a lower concentration of auxin could promote shoot regeneration, whereas the combination of 6-BA and a higher concentration of auxin could lead to excessive callus formation and inhibit the formation of multiple shoots. Among the combinations tested, 6-BA in combination with IAA was found to be more effective for multiple shoot induction than 6-BA in combination with NAA or IBA. The best result was obtained in the medium with 1.0 mg l−1 6-BA and 0.2 mg l−1 IAA, and the highest frequency of shoot induction (100%) with the maximum average number of shoots (5.4) was acquired in this medium (Table 3, Fig. 2E). Based on the degree of explant callusing and the shoot induction response, the medium fortified with 1.0 mg l−1 6-BA and 0.2 mg l−1 IAA was more suitable for multiple shoot induction from shoot stems of T. sinensis and should be used in subsequent research.
Effect of the explant age and AC on callus reduction and the shoot response
The above findings revealed that the shoot stems of T. sinensis were apt to induce calli at the base of explants when they were cultivated on shoot induction medium. To a certain extent the formation of calli at the base of explants is not what we expected, because it would affect the growth of shoots by limiting the absorption of nutrients. In addition, the callus will reduce the quality of regenerated shoots, increase the cost of treatment and production, and even lead to variation among shoots. It is well reported that the physiological state of explants directly affects the in vitro morphogenesis to a certain extent. To examine the effect of explant age on callus reduction and shoot induction, the nodal stems from the in vitro regenerated plantlets at different ages were cultured in medium supplemented with 1.0 mg l−1 6-BA and 0.2 mg l−1 IAA. The results showed that the explant age had an important influence on the regeneration ability of explants (Table 4, Fig. 2E–I). The callus induction rate and callus diameter were inversely proportional to the age of the explants (Table 4). When the 90-day-old shoot stems were cultured on the medium, there was no callus induction. In contrast, the average number of shoots was positively correlated with the age until it reached 75-day-old, but there was almost no difference in the frequency of shoot induction was observed (Table 4, Fig. 2E–I). The results showed that older explants have lower capacities for both shoot and callus regeneration than younger explants of T. sinensis. It has been shown that the difference in the shoot stem regeneration ability under different physiological conditions might be due to their different responses to PGRs in the induction medium (Zhang et al. 2015). For T. sinensis, this finding may be due to the lower cytokinin response of older explants, which is responsible for reducing shoot regeneration and callus induction. Combining the results of shoot induction and callus induction, the shoot stems of 75 day-old were the most suitable explants for multiple shoot induction. The shoot induction frequency was 97.8%, and each explant produced an average of 7.6 shoots.
As an effective adsorbent, AC is widely used in the process of micropropagation to promote the growth and development of different plant species due to its unique ability to reduce harmful substances in the culture medium (Thomas 2008). In this study, to test the effect of AC on callus reduction and the shoot regeneration response, the in vitro 30-day-old shoot stems of T. sinensis were cultivated in medium fortified with various contents of AC, 1.0 mg l−1 6-BA and 0.2 mg l−1 IAA. The results showed that AC in the medium had a significant effect on the regeneration capacity and morphogenesis of the explants (Table 5). For the explants inoculated in the medium supplemented with AC, AC could markedly suppress callus formation, and the callus induction rate and the callus diameter were inversely proportional to the content of AC. The explants cultured in medium supplemented with at least 0.5 g l−1 AC exhibited no callus induction (Table 5). Similar results were found for the callus induction of rice (Chutipaijit and Sutjaritvorakul, 2018). In addition, we found that the addition of AC was not beneficial for the formation of multiple shoots, and the mean number of shoots decreased with increases in the AC content (Table 5). However, AC in the medium could promote the growth and maturation of shoots. Seedlings with strong white roots could be directly formed in one step when the stems are cultivated on medium supplemented with at least 0.5 g l−1 AC for 4 weeks (Fig. 2J). To a certain extent, this finding can identify a new dirction for the industrial micropropagation of T. sinensis. The addition of AC in cultures has induced different morphogenetic responses in many plant species, such as promoting bud proliferation and seedling growth of foxtail millet (Rathinapriya et al. 2019), improving primary culture survival rates of oil palm (Susanthi et al. 2019), and promoting the adventitious root formation of wheat (Dong et al. 2020). Thus far, the underlying mechanism through which AC stimulates plant development is unclear. A relevant study in wheat showed that AC stimulates plant growth by regulating the expression of genes related to cell differentiation (Dong et al. 2020).
Regenerated shoot multiplication and elongation
After 3 weeks of subculture, the shoots proliferated on fresh shoot induction medium supplemented with 1.0 mg l−1 6-BA and 0.2 mg l−1 IAA (Fig. 2 K). The shoots were then isolated separately and subjected to elongation culture. Among the PGRs tested, a low concentration of ZT was more beneficial to shoot elongation and growth, and the shoots treated with a low concentration of ZT were longer than those treated with GA3. The promoting effect of ZT on shoot elongation of kiwiberry (Abdullah et al. 2021) and olive (Bayraktar et al. 2020) has also been reported. In addition, we also found that the combination of 6-BA and GA3 at low concentrations could also significantly improve shoot length. According to the shoot length and shoot growth, MS medium containing 0.5 mg l−1 ZT was more suitable for the elongation of shoots of T. sinensis, and the average shoot length was approximately 3.8 cm (Table 6, Fig. 2L).
In vitro rooting of T. sinensis
Effect of auxins on rooting
As an important organ for water and inorganic salt absorption, the formation of adventitious roots is the key to the success of in vitro micropropagation (Steffens and Rasmussen, 2016; Vidoz et al. 2010). In addition, a healthy and strong root system is helpful to maximize the biomass of regenerated plants. However, for many woody species, the formation of adventitious roots is a limiting factor for in vitro propagation. Therefore, how to make plants produce many strong roots under in vitro conditions is of great significance to the successful establishment of regeneration systems. The formation of adventitious roots is very complex and is strictly regulated by the internal development process of shoots, environmental input, and hormone signals (Gonin et al. 2019). Auxin plays an important role in the initiation of adventitious roots (Shu et al. 2019), and the optimal type and concentration of auxin for adventitious root induction vary with plant species. In this study, elongated shoots were transferred into 1/2 MS medium containing various types of auxins for rooting, and the results are presented in Table 7. For all types of auxins tested in this study, especially for IAA and NAA, we found that low concentrations of these auxins were more beneficial for adventitious root induction (Table 7). The shoots cultured in medium supplemented with a high concentration (> 1.0 mg l−1) of IAA, IBA, or K-IBA produced only short and thickened roots, and compact callus formed at the root base, which led to retardation of the shoots. In medium containing a high concentration (≥ 0.5 mg l−1) of NAA, most explants produced only a large number of calli in the base without adventitious root formation. In the medium fortified with 0.5 mg l−1 K-IBA, the root induction rate was the highest (88.9%), each explant produced 3.6 roots, and the average length of the roots was 2.8 cm (Table 7). In adventitious root induction practice, IBA is usually the most commonly used rooting PGR because of its stability and effectiveness in promoting adventitious roots formation (Lakehal and Bellini, 2019; Pacurar et al. 2014). In the present study, K-IBA showed the best root induction response for T. sinensis, and the superiority of K-IBA in adventitious root induction may be attributed to several factors, including that it increases the solubility of IBA salts, which are more beneficial for transport, absorption, and metabolism to potential rooting origin sites with shoot stems (Stevens and Pijut, 2017).
Effect of sucrose, macronutrient strength, and AC on rooting
Sucrose, as the source of energy and a signaling molecule, can coordinate with auxins to regulate the formation of adventitious roots (Cheng et al. 2020). In addition, in the in vitro rooting process, the macronutrients in the culture medium not only provide sufficient nutrition for rooting, but also affect the development of adventitious roots as a functional penetrant. In our present study, to reduce the production cost, the effects of the sucrose content, the macronutrient strength of the culture media, and AC on the rooting of T. sinensis were further examined. The results showed that the rooting response was directly proportional to the sucrose content in the medium until the sucrose content increased to 10.0 g l−1. In the medium supplemented with 10.0 g l−1 sucrose and 0.5 mg l−1 K-IBA, the rooting rate was as high as 95.6%, each shoot produced an average number of 4.5 roots, and the root length was as long as 3.8 cm (Table 8). In contrast, in the medium without sucrose, only 37.8% of shoots produced thin and short roots, and the plantlets ultimately turned yellow. The results suggested that leaf photosynthesis alone could not provide enough energy for rooting, and the addition of a low concentration of sucrose to the rooting medium could not only promote the formation of adventitious roots but also stimulate root elongation. Similar results were found for the adventitious root formation of S. sebiferum (Hou et al. 2020).
The analysis of the shoots cultivated in rooting medium containing different macronutrient strengths and 0.5 mg l−1 K-IBA revealed that a relatively low strength of macronutrients was more conducive to rooting than a high strength of macronutrients (Table 9). With decreases in the strength of macronutrients in the medium, the rooting response increased gradually until the strength decreased to 1/4-fold (Table 9). The rooting rate and the mean roots produced per explant observed in medium with a macronutrient strength less than 1/4-fold were higher than those found in medium containing macronutrients at full or half-strength, however, the shoots on the former medium suffered from leaf chlorosis, and some leaves remained yellow after domestication. The best rooting response and plant growth were observed in 1/4 MS medium supplemented with 0.5 mg l−1 K-IBA and 10.0 g l−1 sucrose (Table 9, Fig. 2M). The adventitious root induction rate was 100%, each explant produced 5.7 roots, and the average root length was 4.4 cm. In other woody plants, such as cherry trees (Sun et al. 2018) and apple trees (Sun et al. 2016a), the medium with low strength of macronutrients was more favorable for in vitro rooting. The reason may be that the concentration of macronutrients required for rooting is much lower than that required for shoot induction and growth. In addition, the osmotic pressure of the medium with low macronutrient strength was low, which not only promotes the absorption of nutrients but also stimulates the formation of root primordia.
We further found that when the elongated shoots were transferred to 1/4 MS medium supplemented with 0.5 mg l−1 K-IBA, 10 g l−1 sucrose, and 0.5 g l−1 AC, the plantlets grew vigorously with longer and developed roots than those cultured on the medium without AC (Fig. 2N). The results indicated that supplementation of the culture media with AC could significantly improve the growth and maturation of shoots and the development of roots. Similar studies on the effects of AC in promoting plant regeneration and root growth have been performed in many plants (Dong et al. 2020; Navarro et al. 2021; Rathinapriya et al. 2019).
Domestication and transplantation
The success of the regeneration technology ultimately depends on whether the regenerated plantlets can be transplanted from rooting medium with a high frequency of survival to the seedling substrate, and then transferred to the field. During the process of transplantation, the transition period from culture room to field conditions is critical. It is well documented that in the process of domestication or hardening of the in vitro regenerated plantlets, relatively low humidity and high light intensity under greenhouse or field conditions will create an adverse/stressful environment for the directly exposed regenerated plantlets, which will limit the survival rate of plants to a certain extent. In addition, the transplanting matrix also plays a key role in the acclimatization of the regenerated plantlets (Mali and Chavan 2016). Previous studies have indicated that one of the main obstacles to the large-scale propagation of T. sinensis using plant tissue culture technology is the high mortality rate of plantlets during domestication (Kang et al. 2002). Therefore, to obtain a higher survival percentage, the effects of the composition and ratio of the nutrient matrix as well as the acclimation methods on the survival of regenerated plantlets were examined. The results showed that the types and proportions of transplanting nutrient substrates exerted important effects on the survival of regenerated plantlets of T. sinensis. The plantlets transplanted in the nutrient matrix with Pindstrup substrate presented a higher survival rate and better vigor than those acclimatized in the matrix without Pindstrup substrate (Fig. 3). Among the different types and proportions of transplanting substrates tested, the perlite:vermiculite:Pindstrup substrate (1:1:1) mixture was found to be more suitable for the acclimatization of the regenerated plantlets of T. sinensis. After 6 weeks of acclimatization, the highest survival rate (84.7%) and better vigor of the plantlets were obtained on this transplanting substrate. However, considering the transplanting cost, the garden soil:perlite:vermiculite:pindstrup substrate (3:1:1:3) mixture was used in the following large-scale transplantation experiment. The survival rate was as high as 81.7%, and no significant difference was found between the plantlets grown on this nutrient matrix and those grown on the perlite:vermiculite:Pindstrup substrate (1:1:1) mixture.
To further improve the transplanting survival rate of T. sinensis, the effects of domestication methods on the transplantation of in vitro regenerated plantlets were studied. After 6 weeks of domestication, the three methods mentioned above succeeded in plantlet acclimatization, but the survival rate differed (Table 10). The survival rate of the regenerated plants acclimatized by method II and method III was relatively higher than that obtained with domestication method I (Table 10). We speculated that the higher survival rate obtained with methods II and III could be attributed to the plant status during the domestication period, in which the plants grew well in the plastic tray or hydroponic tray, and each plantlet had a strong root system before transplantation into the nursery bag. The results showed that the combination of mini-incubators and a greenhouse was suitable for the acclimatization of the regenerated plantlets of T. sinensis. The advantage of the domestication method II or III used in this study was that they could reduce the transplanting pressure of the regenerated plants because the growth environment of the transplanted plants was similar to that during the rooting period of tissue culture. The plantlets grew vigorously and the highest frequency of survival (95.3%) was obtained when the plantlets were initially acclimatized by the hydroponic system for 2 weeks before transplantation into the nursery bag (domestication method III). Most importantly, plantlets domesticated by hydroponics grew faster, had longer and stronger adventitious roots, and formed more fibrous roots (Fig. 2O). Some evidence demonstrates that regenerated plantlets transplanted directly usually have tender roots and lack root hairs, which affects the survival rate of large-scale transplantation. In contrast, the plantlets domesticated by hydroponics developed roots and a strong plant morphology, which are more conducive to their survival under transplantation conditions. Hence, the application of a hydroponic system in the domestication of the plantlets of T. sinensis could reduce the problems caused by poor root architecture. One month after transplantation, the regenerated plantlets grew healthily and had the same appearance as the wild plants (Fig. 2P). Three months later, the domesticated plants were successfully cultivated in the field.
Genetic stability assessment
The genetic integrity of regenerated plantlets is one of the basic requisites for the implementation of any regeneration scheme. In our study, the genetic stability of regenerants of T. sinensis was evaluated by ISSR markers. During the ISSR analysis, 40 primers were screened and only 8 primers yielded clear and reproducible bands. The band numbers ranged from 5 (UBC-840 and UBC-879) to 9 (UBC-815), and the band sizes ranged from approximately 150 bp to 2000 b (Table 11). Among the 8 primers tested, UBC-826 and UBC-879 amplified 6 and 5 monomorphic bands, respectively, and they are shown in Fig. 4A and B. The amplification bands of all the regenerants and mother plants were the same and monomorphic, which confirmed the genetic stability of the regenerated plants of T. sinensis (Fig. 4). In recent years, many researchers have used ISSR markers to detect the homogeneity of many economically important woody plants regenerated from in vitro culture and to confirm whether these plantlets are identical to the mother plant, such as Pterocarpus marsupium (Ahmad et al. 2021), Eucalyptus nitens (Ayala et al. 2019) and Moringa peregrina (Al Khateeb et al. 2013).
Genetic fidelity assessment of the mother plant and in vitro regenerated plantlets of T. sinensis by ISSR molecular markers. A Profiles produced by primer UBC-826. B Profiles produced by primer UBC-879. Lane MP: Mother plant. Lanes 1–10: Randomly selected regenerated plantlets. Lane M: Molecular ruler (2 kb DNA ladder)
Conclusions
In conclusion, the present study established a highly efficient, repeatable, and cost-effective micropropagation procedure for T. sinensis via direct shoot organogenesis using shoot stems with axillary buds or terminal buds as explants. The highest shoot induction frequency (97.8%) with smaller calli was obtained when the explants were cultured in MS medium containing 1.0 mg l−1 6-BA and 0.2 mg l−1 IAA. In addition, 0.5 mg l−1 ZT was proven to be more conducive to shoot elongation. When the elongated shoots were cultured in 1/4 MS medium supplemented with 0.5 mg l−1 K-IBA, 0.5 g l−1 AC, and 10.0 g l−1 sucrose, the highest rooting response (100%) was achieved. In addition, the present study proposes an effective hydroponic domestication method that could significantly improve the survival rate of T. sinensis. The highest transplanting survival rate (95.3%) was obtained in the mixture of garden soil, perlite, vermiculite and Pindstrup substrate (3:1:1:3). Furthermore, the results from the evaluation of ISSR molecular markers showed that the heredity of the regenerated plantlets was consistent with that of the donor plants, and the in vitro regenerated plantlets were “true to type”. The regeneration scheme established in this study has great application potential and can continuously provide plantlets of T. sinensis within a short time. The method is thus of great significance to germplasm preservation of T. sinensis and its sustainable industrialized utilization in food.
Abbreviations
- AC:
-
Activated charcoal
- ANOVA:
-
Analysis of variance
- MS medium:
-
Murashige and Skoog medium
- 6-BA:
-
N6-benzyladenine
- GA3 :
-
Gibberellic acid
- IBA:
-
Indole-3-butyric acid
- IAA:
-
Indole-3-acetic acid
- ISSR:
-
Inter-simple sequence repeat
- K-IBA:
-
Potassium salt of indole butyric acid
- KT:
-
Kinetin
- NAA:
-
A-naphthaleneacetic acid
- PGRs:
-
Plant growth regulators
- CRD:
-
Completely randomized design
- TDZ:
-
Thidiazuron
- ZT:
-
Zeatin
References
Abdullah M, Sliwinska E, Goralski G, Latocha P, Tuleja M, Widyna P, Popielarska-Konieczna M (2021) Effect of medium composition, genotype and age of explant on the regeneration of hexaploid plants from endosperm culture of tetraploid kiwiberry (Actinidia arguta). Plant Cell Tissue Organ Cult 147:569–582
Ahmad A, Ahmad N, Anis M, Alatar AA, Abdel-Salam EM, Qahtan AA, Faisal M (2021) Gibberellic acid and thidiazuron promote micropropagation of an endangered woody tree (Pterocarpus marsupium Roxb.) using in vitro seedlings. Plant Cell Tissue Organ Cult 144:449–462
Al Khateeb W, Bahar E, Lahham J, Schroeder D, Hussein E (2013) Regeneration and assessment of genetic fidelity of the endangered tree Moringa peregrina (Forsk.) Fiori using Inter Simple Sequence Repeat (ISSR). Physiol Mol Biol Plants 19:157–164
Ayala PG, Brugnoli EA, Luna CV, Gonzalez AM, Pezzutti R, Sansberro PA (2019) Eucalyptus nitens plant regeneration from seedling explants through direct adventitious shoot bud formation. Trees-Struct Funct 33:1667–1678
Bayraktar M, Hayta-Smedley S, Unal S, Varol N, Gurel A (2020) Micropropagation and prevention of hyperhydricity in olive (Olea europaea L.) cultivar “Gemlik.” S Afr J Bot 128:264–273
Bingkun T, Pengcheng W, Yaomei Y (2002) Study on cutting propagation of Toona sinensis. China Veg 1:13–15
Cai W, Duan H, Wang J, Zhu Y (2019) Establishment of in vitro rapid micropropagation of four table grape cultivars. J Nucl Agric Sci 33:248–254
Cao J-J, Lv Q-Q, Zhang B, Chen H-Q (2019) Structural characterization and hepatoprotective activities of polysaccharides from the leaves of Toona sinensis (A. Juss) Roem. Carbohyd Polym 212:89–101
Chen C-J, Michaelis M, Hsu H-K, Tsai C-C, Yang KD, Wu Y-C, Cinatl J Jr, Doerr HW (2008) Toona sinensis Roem tender leaf extract inhibits SARS coronavirus replication. J Ethnopharmacol 120:108–111
Chen GH, Huang FS, Lin YC, Hsu CK, Chung YC (2013) Effects of water extract from anaerobic fermented Toona sinensis Roemor on the expression of antioxidant enzymes in the Sprague-Dawley Rats. J Funct Foods 5:773–780
Chen CH, Li CJ, Tai IC, Lin XH, Hsu HK, Ho MLJICT (2017) The fractionated Toona sinensis leaf extract induces apoptosis of human osteosarcoma cells and inhibits tumor growth in a murine xenograft model. Integr Cancer Ther 16:397–405
Cheng L, Zhao M, Hu Z, Liu H, Li S (2020) Comparative transcriptome analysis revealed the cooperative regulation of sucrose and IAA on adventitious root formation in lotus (Nelumbo nucifera Gaertn). BMC Genomics 21:1–15
Chih-Yao H, Pung-Ling H, Chih-Ming C, Chi-Tang M, Shu-Miaw CJCPB (2012) Tangy scent in Toona sinensis (Meliaceae) leaflets: isolation, functional characterization, and regulation of TsTPS1 and TsTPS2, two key terpene synthase genes in the biosynthesis of the scent compound. Curr Pharm Biotechnol 13:2721–2732
Chutipaijit S, Sutjaritvorakul T (2018) Application of activated charcoal and nanocarbon to callus induction and plant regeneration in aromatic rice (Oryza sativa L.). Chem Speciat Bioavailab 30:1–8
Dong J, Yang W, Wang M, Ma Y (2013) Analysis of characteristic aroma components of Toona sinensis fruits grown in Yuxi, Yunnan Province. Food Sci 34:217–220
Dong F-S, Lv M-Y, Wang J-P, Shi X-P, Liang X-X, Liu Y-W, Yang F, Zhao H, Chai J-F, Zhou S (2020) Transcriptome analysis of activated charcoal-induced growth promotion of wheat seedlings in tissue culture. BMC Genet 21:1–11
Gonin M, Bergougnoux V, Nguyen TD, Gantet P, Champion A (2019) What makes adventitious roots? Plants-Basel 8(240):1–24
Griffin GJ, Hale MG, Shay FJ (1976) Nature and quantity of sloughed organic-matter produced by roots of axenic peanut plants. Soil Biol Biochem 8:29–32
Hou JY, Mao YJ, Su PF, Wang DC, Chen X, Huang SW, Ni J, Zhao WW, Wu LF (2020) A high throughput plant regeneration system from shoot stems of Sapium sebiferum Roxb., a potential multipurpose bioenergy tree. Ind Crops Prod 154:112–653
Hsiang CY, Hseu YC, Chang YC, Kumar KJS, Ho TY, Yang HL (2013) Toona sinensis and its major bioactive compound gallic acid inhibit LPS-induced inflammation in nuclear factor-kappa B transgenic mice as evaluated by in vivo bioluminescence imaging. Food Chem 136:426–434
Jiang X, Zhang B, Lei M, Zhang J, Zhang J (2019) Analysis of nutrient composition and antioxidant characteristics in the tender shoots of Chinese toon picked under different conditions. LWT-Food Sci Technol 109:137–144
Kaeppler SM, Kaeppler HF, Rhee Y (2000) Epigenetic aspects of somaclonal variation in plants. Plant Mol Biol 43:179–188
Kakumu A, Ninomiya M, Efdi M, Adfa M, Hayashi M, Tanaka K, Koketsu M (2014) Phytochemical analysis and antileukemic activity of polyphenolic constituents of Toona sinensis. Bioorg Med Chem Lett 24:4286–4290
Kang B, Zhang X, Chen Y, Zhang J, Li J, Cao J (2002) Effect of PP_(333) and CCC on growth multiplications and rooting of Toona sinensis in tissue culture. Acta Bot Boreali-Occidentalla Sinica 22:411–415
Kuo C-EA, Wu S-Y, Lee C-H, Lai Y-R, Lu C-H, Chen P-C, Cheng J-H, Tsai L-Y, Yen K-T, Tsao Y, Tsai S-M (2020) Toona sinensis modulates autophagy and cytokines in lipopolysaccharide- induced RAW 264.7 macrophages. Biomed Pharmacother 129:110–386
Lakehal A, Bellini C (2019) Control of adventitious root formation: insights into synergistic and antagonistic hormonal interactions. Physiol Plant 165:90–100
Li P, Miao X, Wu J, Hu J (2011) Physiological effects of chitosan on seeds germintion and seedling growth of Toona sinensis. J Northw For Univ 26:44–47
Li F, Gao Y, Xiao X, Chen Q, Xiao H (2017) Effects of Toona sinensis leaf alcohol extract on lifespan and antioxidant activity of Drosophila melanogaster. J Fujian Normal Univ Nat Sci Ed 33:59–64
Liao J-W, Chung Y-C, Yeh J-Y, Lin Y-C, Lin Y-G, Wu S-M, Chan Y-C (2007) Safety evaluation of water extracts of Toona sinensis Roemor leaf. Food Chem Toxicol 45:1393–1399
Liao JW, Yeh JY, Lin YC, Wei MM, Chung YC (2009) Mutagenicity and safety evaluation of water extract of fermented Toona sinensis roemor leaves. J Food Sci 74:T7–T13
Liqiong XU, Bingkun TU (2007) Studies on leaf tissue culture and rapid propagation of Toona sinensis. Hubei Agric Sci 46:24–26
Lin S, Chen C, Luo H, Xu W, Zhang H, Tian J, Ju R, Wang L (2019) The combined effect of ozone treatment and polyethylene packaging on postharvest quality and biodiversity of Toona sinensis (A.Juss.) M.Roem. Postharvest Biol Technol 154:1–10
Luo XD, Wu SH, Ma YB, Wu DG (2000) Limonoids and phytol derivatives from Cedrela sinensis. Fitoterapia 71:492–496
Mali AM, Chavan NS (2016) In vitro rapid regeneration through direct organogenesis and ex-vitro establishment of Cucumis trigonus Roxb.-an underutilized pharmaceutically important cucurbit. Ind Crops Prod 83:48–54
Mu R, Wang X, Liu S, Yuan X, Wang S, Fan Z (2007) Rapid determination of volatile compounds in Toona sinensis (A. Juss.) Roem. by MAE-HS-SPME followed by GC-MS. Chromatographia 65:463–467
Murashige T, Skoog F (1962) A revised medium for rapid growth and bio assays with tobacco tissue cultures. Physiol Plant 15:473–497
Navarro QR, Correa DO, Behling A, Noseda MD, Amano E, Suzuki RM, Fortes Ribas LL (2021) Efficient use of biomass and extract of the microalga Desmodesmus subspicatus (Scenedesmaceae) in asymbiotic seed germination and seedling development of the orchid Cattleya warneri. J Appl Phycol 33:2189–2207
Oh TJ, Cullis MA, Kunert K, Engelborghs I, Swennen R, Cullis CA (2007) Genomic changes associated with somaclonal variation in banana (Musa spp.). Physiol Plant 129:766–774
Pacurar DI, Perrone I, Bellini C (2014) Auxin is a central player in the hormone cross-talks that control adventitious rooting. Physiol Plant 151:83–96
Pernisova M, Grochova M, Konecny T, Plackova L, Harustiakova D, Kakimoto T, Heisler MG, Novak O, Hejatko J (2018) Cytokinin signalling regulates organ identity via the AHK4 receptor in Arabidopsis. Development 145(14):163907
Qin MA, Kanren DAI, Lifen D, Tianru WEI (2006) Propagation of Toona sinensis by tissue culture technology. J Northw For Coll 21:106–108
Rathinapriya P, Satish L, Rameshkumar R, Pandian S, Rency AS, Ramesh M (2019) Role of activated charcoal and amino acids in developing an efficient regeneration system for foxtail millet (Setaria italica (L.) Beauv.) using leaf base segments. Physiol Mol Biol Plants 25:533–548
Ren L, Wan W, Xie Y, Chen B, Wang G, Yin D, Su X, Cao X (2021) Identification of volatile aroma compounds of Toona sinensis (A. Juss) Roem buds and investigation of genes expression profiles confering aroma production. Pak J Bot 53:1459–1464
Savita, Bhagat A, Pati PK, Virk GS, Nagpal A (2012) An efficient micropropagation protocol for Citrus jambhiri Lush. and assessment of clonal fidelity employing anatomical studies and RAPD markers. In Vitro Cell Dev Biol-Plant 48:512–520
Sharma U, Kataria V, Shekhawat NS (2017) In vitro propagation, ex vitro rooting and leaf micromorphology of Bauhinia racemosa Lam.: a leguminous tree with medicinal values. Physiol Mol Biol Plants 23:969–977
Shi Y, Liu T, Han Y, Zhu X, Zhao X, Ma X, Jiang D, Zhang QJFC (2017) An efficient method for decoloration of polysaccharides from the sprouts of Toona sinensis (A. Juss.) Roem by anion exchange macroporous resins. Food Chem 217:461–468
Shu W, Zhou H, Jiang C, Zhao S, Wang L, Li Q, Yang Z, Groover A, Lu M-Z (2019) The auxin receptor TIR1 homolog (PagFBL 1) regulates adventitious rooting through interactions with Aux/IAA28 in Populus. Plant Biotechnol J 17:338–349
Singh ND, Sahoo L, Sarin NB, Jaiwal PK (2003) The effect of TDZ on organogenesis and somatic embryogenesis in pigeonpea (Cajanus cajan L. Millsp). Plant Sci 164:341–347
Steffens B, Rasmussen A (2016) The physiology of adventitious roots. Plant Physiol 170:603–617
Stevens ME, Pijut PM (2017) Origin of adventitious roots in black walnut (Juglans nigra) softwood cuttings rooted under optimized conditions in a fog chamber. New Forest 48:685–697
Su Y-H, Liu Y-B, Zhang X-S (2011) Auxin-cytokinin interaction regulates meristem development. Mol Plant 4:616–625
Su S, Wang L, Ni J, Geng Y, Xu X (2020) Diversity of red, green and black cultivars of Chinese Toon Toona sinensis (A. Juss.) Roem: anthocyanins, flavonols and antioxidant activity. J Food Meas Charact 14:3206–3215
Sun Q, Sun M, Sun H, Bell RL, Li L, Zhang W, Tao J (2016a) Comparative organogenic response of six clonal apple rootstock cultivars. HortScience 51:272–278
Sun XX, Zhang LT, Cao YQ, Gu QY, Yang H, Tam JP (2016b) Quantitative analysis and comparison of four major flavonol glycosides in the leaves of Toona sinensis (A. Juss.) Roemer (Chinese Toon) from various origins by high-performance liquid chromatography-diode array detector and hierarchical clustering analysis. Pharmacogn Mag 12:S270–S276
Sun Q, Sun H, Bell RL, Guan Q (2018) Plant regeneration from in vitro leaf explants of Prunus pseudocerasus Lindl. Prop Ornam Plants 18:3–11
Susanthi, Sparjan B, Ramajayam D, Biology M (2019) Effect of activated charcoal, culture media and plant growth regulators on in vitro germination and development of elite dura oil palm (Elaeis guineensis Jacq.) Zygotic embryos. J Plant Cell Biotechnology 20:314–323
Thomas TD (2008) The role of activated charcoal in plant tissue culture. Biotechnol Adv 26:618–631
Vidoz ML, Loreti E, Mensuali A, Alpi A, Perata P (2010) Hormonal interplay during adventitious root formation in flooded tomato plants. Plant J 63:551–562
Vijendra PD, Jayanna SG, Kumar V, Gajula H, Rajashekar J, Sannabommaji T, Basappa G, Anuradha CM (2017) Rapid in vitro propagation of Lucas aspera Spreng. A potential multipurpose Indian medicinal herb. Ind Crops Prod 107:281–287
Wang R, Liu D, Liu X, Liu F, Xuan L, Tang Y, Li W (2021) Cytotoxicity and polyol pathway inhibitory activities of chemical constituents isolated from the pericarp of Toona sinensis. Nat Prod Res 36:1593–1598
Wei Z, Chao L, You LJ, Xiong F, Chen YS, Luo YQJJoFF (2014) Structural identification of compounds from Toona sinensis leaves with antioxidant and anticancer activities. J Funct Foods 10:427–435
Wybouw B, De Rybel B (2019) Cytokinin: a developing story. Trends Plant Sci 24:177–185
Xie X, Xu K, Xie M, Zhu Y (2015) Optimization of rapid micropropagation system of apple meristem-tip culture. Plant Physiol J 51:2152–2156
Yang W, Cadwallader KR, Liu Y, Huang M, Sun B (2019) Characterization of typical potent odorantsin raw and cooked Toona sinensis (A. Juss.) M. Roem. by instrumental-sensory analysis techniques. Food Chem 282:153–163
Yang H-L, Kuo Y-T, Gowrisankar YV, Lin K-Y, Hsu L-S, Huang P-J, Lin H-C, Hseu Y-C (2020) The leaf extracts of Toona sinensis and fermented culture broths of Antrodia camphorata synergistically cause apoptotic cell death in promyelocytic leukemia cells. Integr Cancer Ther 19:1–13
Yousheng C, Sziklai O (1985) Preliminary-study on the germination of Toona-sinenesus (Juss, A.) Roem seed from 11 Chinese provenances. For Ecol Manage 10:269–281
Yu WJ, Chang CC, Kuo TF, Tsai TC, Chang SJ (2012) Toona sinensis Roem leaf extracts improve antioxidant activity in the liver of rats under oxidative stress. Food Chem Toxicol 50:1860–1865
Zhang W, Li C, You LJ, Fu X, Chen YS, Luo YQ (2014) Structural identification of compounds from Toona sinensis leaves with antioxidant and anticancer activities. J Funct Foods 10:427–435
Zhang T-Q, Lian H, Tang H, Dolezal K, Zhou C-M, Yu S, Chen J-H, Chen Q, Liu H, Ljung K, Wang J-W (2015) An intrinsic microRNA timer regulates progressive decline in shoot regenerative capacity in plants. Plant Cell 27:349–360
Acknowledgements
The study was financially supported by the Youth Innovation Promotion Association CAS (Grant No. 2021447), Chinese Academy of Sciences-Henan Province Achievement transfer and Transformation Project (2020205 and 2021P02), the Grant of the President Foundation of Hefei Institutes of Physical Science of Chinese Academy of Sciences (YZJJZX202013), and the Strategic Biological Resources Planning Project of the Chinese Academy of Sciences (KFJ-BRP-007-014). The authors are thankful to Dr. Xiaoping Xu for his kind help in manuscript revision.
Author information
Authors and Affiliations
Contributions
HJY Methodology, Data curation, Formal analysis, Writing-original draft, Writing—review & editing. WDC. Visualization, Formal analysis. SPF Investigation, Supervision. DSS Resources. WLF Project administration, Writing—review & editing, Conceptualization.
Corresponding author
Ethics declarations
Conflict of interest
Authors do not have any conflict of interests.
Additional information
Communicated by Paloma Moncaleán.
Publisher's Note
Springer Nature remains neutral with regard to jurisdictional claims in published maps and institutional affiliations.
Rights and permissions
Springer Nature or its licensor holds exclusive rights to this article under a publishing agreement with the author(s) or other rightsholder(s); author self-archiving of the accepted manuscript version of this article is solely governed by the terms of such publishing agreement and applicable law.
About this article
Cite this article
Hou, J., Wang, D., Su, P. et al. In vitro large-scale propagation and genetic fidelity of Toona sinensis (Juss.) M.Roem., an economically important vegetable tree. Plant Cell Tiss Organ Cult 151, 275–291 (2022). https://doi.org/10.1007/s11240-022-02351-z
Received:
Accepted:
Published:
Issue Date:
DOI: https://doi.org/10.1007/s11240-022-02351-z