Abstract
Coal seams with low gas permeability and high gas outburst hazards are becoming more serious as coal mines extend deeper, but there are no appropriate protective coal seams for this kind of coal seam in China. In this paper, mining technology using a protective seam with nearly whole rock (PSNWR) is used to improve gas drainage and ensure safety during production. The characteristics of the distribution and occurrence of PSNWRs and their mechanical properties are analyzed. A theoretical mechanics model and three-dimensional numerical model are established to study the controlling effect of PSNWR mining on pressure-relief gas drainage. In this context, the mining process, system and gas extraction design for PSNWRs are introduced. The results for Pingdingshan No. 12 Coal Mine show that mining with a PSNWR 2.0 m thick can effectively reduce the danger of coal and gas outbursts and improve gas drainage and utilization. The gas drainage rates are >80 %, which significantly increases the social, economic and environmental benefits of Pingdingshan No. 12 Coal Mine.
Similar content being viewed by others
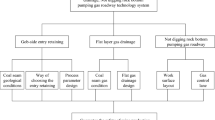
Avoid common mistakes on your manuscript.
1 Introduction
Since the first reported coal and gas outburst occurred in the Issac Colliery, Loire coal field, France, in 1843 (Lama and Bodziony 1996), this hazard has occurred in most coal-producing countries and has posed a challenge to coal mine safety experts (Beamish and Crosdale 1998; Díaz Aguado and González Nicieza 2007a; Yang et al. 2012). In recent years, with the expansion of the scale of coal mining and the increase in mining depth, coal mine gas outbursts have become the main factor restricting high productivity and high efficiency, especially in the exploitation of coal seams with low gas permeability and high gas. Coal and gas outbursts are natural disasters that influence coal mine safety and production. China now has the world’s highest risk of coal and gas outburst disasters (Cheng 2010). By 2012, 800 coal mines in China were in danger of coal and gas outbursts (Li 2014), and the death toll for coal and gas outbursts from 2006 to 2010 was 1199 (Wang et al. 2014).
There are several ways to control and prevent coal and gas outbursts (Flores 1998; Noack 1998; Díaz Aguado and González Nicieza 2007b; Lu et al. 2009; Tian and Zheng 2011), but a comparative analysis indicates that protective seam mining technology is the most economical and effective method (Yu et al. 2004; Brandt and Sdunowski 2007; SAWS 2005, 2008). The main idea of the technique is first to mine a coal seam with a low risk of coal and gas outbursts, which serves to relieve the stress in the protected coal seam and improve the gas permeability of the protected coal seam (Yang et al. 2011). Unfortunately, there are no appropriate protective coal seams for many coal seams are in danger of coal and gas outbursts in China (Li 2014). In the three levels of the Ji15 coal seam in Pingdingshan No. 12 Coal Mine, whose gas outburst hazard is serious, the upper Ji14 coal seam has good coal quality and is without the risk of coal and gas outbursts, but the distribution and occurrence of the Ji14 coal seam is not stable. The average thickness is 0.5 m and in most areas there is no coal seam, so mining such a protective seam with nearly whole rock (PSNWR) will produce a large amount of waste rock. The key technology includes the mining scheme, process, system arrangement and gas extraction design.
This study focused on the geological conditions of the Pingdingshan No. 12 Coal Mine in Henan Province. Based on the characteristics of the distribution and occurrence of a PSNWR and its mechanical properties, the expansive deformation and pressure-relief gas drainage of the protected seam and the depth of PSNWR floor damage are studied using theoretical analysis and numerical modeling. In addition, the mining process, system and gas extraction design of the PSNWR are introduced. The results for Pingdingshan No. 12 Coal Mine show that the technology can effectively reduce the danger of coal and gas outbursts, improve gas drainage and utilization and has great application prospects.
2 Study areas
2.1 Geological setting of Pingdingshan Coal Mine
The Pingdingshan No. 12 Coal Mine, located in eastern Henan Province, Pingdingshan, is about 7.5 km from Pingdingshan city center. The mine, put into production on July 1, 1960, has seen more than 50 years of exploitation history. At present, the main recoverable section is the Ji15 seam. The coal reserves are 32.323 million t and the recoverable reserves are 21.253 million t. The seam is 800 m underground and 3.2 m thick, and it is a typical low-permeable gas-rich seam. Due to its high gas content, low permeability and low gas drainage ratio, the technical difficulty of safe mining has begun to hinder its sustainable development. The drilling and disclose of the Ji15 coal seam indicate that the gas content is about 15.26–19.14 m3/t, the gas pressure is 1.78–2.3 MPa, the porosity is 2.37 % and the permeability coefficient is 0.00112–0.076 m/d. Figure. 1 shows the location of the Pingdingshan Coal Mine and the layout of the mining area.
2.2 Characteristics of distribution and occurrence of the Ji14 coal seam
Based on the drilling and disclose of the Ji14 coal seam, we analyze and forecast its distribution and occurrence. A three-dimensional distribution of the Ji14 coal seam is shown in Fig. 2.
Based on the distribution and occurrence of the Ji14 coal seam, combined with field practice, the thickness of the coal seam along the directions of the Ji14-31010 coalface and the Ji14-31030 coalface when advancing is shown in Fig. 3.
According to Figs. 2 and 3, we can see that the thickness of the coal seam along the directions of the Ji14-31010 coalface and the Ji14-31030 coalface when advancing is rather changeable. The thickness ranged from 0 to 0.8 m, the average coal thickness was 0.5 m and in most areas there is no coal seam. In actual production, part of the floor rock also needs to be mined to improve the pressure-relief gas drainage. Mining such a rock-coal seam as a PSNWR produces a large amount of waste rock (>80 %), and the technology has to be more advanced.
3 Key parameters of PSNWR
To optimize the design of the PSNWR mining scheme and improve the effects of gas drainage, the tensile strength, compressive strength and shear strength of rock samples from the Ji14 coal seam were tested (Wang et al. 2013; Zhang et al. 2014). The key parameters are shown in Tables 1, 2 and 3.
The test results show that the average tensile strength of the rock in the Ji14 coal seam is 2.455 MPa, the average compressive strength is 18.84 MPa, and when the shear angle is 45°, 55° or 65°, the average shear strength is 1.585, 1.475 or 0.875 MPa, respectively. According to the Mohr–Coulomb rule, the cohesion c is 0.611 MPa, and the internal friction angle \(\varphi\) is 34.84°. In addition, the Protodyakonov coefficient f is 7–9.
4 Mining scheme for PSNWR
4.1 Theoretical analysis
When the PSNWR is extracted, the overburden stress will transfer, resulting in high stress in front of the face. A mechanical model was established in the strike direction (Meng et al. 2010; Wang 2008), as shown in Fig. 4. M(x,y) are the coordinates of a point on the floor, S 1 and S 2 are the zone of influence of the front abutment pressure, γH is the in situ stress and k is the stress concentration factor of the front abutment pressure.
The stress on the unit length in front of the face is:
The stress at M(x, y) caused by \(q(\eta )\) is:
The stress at M(x, y) caused by the whole of the loading is:
According to the Mohr–Coulomb rule, the maximum shear stress at M(x, y) is:
The damage criterion for M(x, y) is:
where \(\sigma_{x}\) is the vertical stress, \(\sigma_{y}\) is the horizontal stress, \(\tau_{xy}\) is the shear stress, \(\varphi\) is the internal friction angle and \(c\) is the cohesion, based on the mining geological conditions and the key parameters of PSNWR tested in Sect. 3. Finally, we obtain that when the mining thickness is 0.5, 1.0, 1.5, 2.0 or 2.5 m, the maximum damage depth for PSNWR is 5.5, 6.1, 7.8, 14 and 17.5 m, respectively.
4.2 Numerical modeling and simulation scheme
FLAC3D (Itasca Consulting Group Inc., 2006), developed by Itasca Consulting Group, Inc., is widely used in mining engineering, and the Mohr–Coulomb model is the conventional model used to represent the problems of coal and rock damage. The gas pressure and seepage laws can be studied using the FLAC3D stress and seepage coupling simulation (Yang et al. 2014; Hou et al. 2012; Ma et al. 2016; Wei et al. 2016), which is beneficial to guiding the mining scheme for PSNWR. The Mohr–Coulomb model was used in our model, and the mechanical and seepage parameters applied in this simulation were mainly determined according to experiments in the Pingdingshan Coal Mine, and these are shown in Table 4. The numerical model assumes a length of 200 m in the dip direction, a width of 300 m in the strike direction and a height of 80 m, as shown in Fig. 5. The horizontal displacements of the four vertical planes of the model are restricted in the normal direction, and the vertical displacement at the base of the model is set to zero. At the top of the model, a vertical load (p = 2.5 MPa) is applied. At the bottom of the model, the gas pore pressure in the Ji15 coal seam is defined as 1.78 MPa.
4.3 Analysis results
According to the simulation scheme for PSNWR in Sect. 4.2, when the mining height of the PSNWR is 0.5, 1.0, 1.5, 2.0 and 2.5 m (i.e., the mining height of the rock is 0, 0.5, 1.0, 1.5 and 2.0 m), and the mining advance is 120 m in the strike direction, then the maximum deformation of the Ji15 coal seam is 4.52, 6.73, 8.65, 12.18 and 16.48 mm, respectively. The deformation of the Ji15 coal seam is shown in Fig. 6.
According to the coal mine safety rules, the critical value for the gas pressure is 0.74 MPa, the critical value for the gas content is 8 m3/t and the critical value for the relative expansion deformation is 3 ‰ (State Administration of Work Safety of China 2010), so the mining height of the PSNWR should be >1.5 m. Through the stress and seepage coupling simulation, when the mining height of PSNWR is 0.5, 1.0, 1.5, 2.0 and 2.5, and the mining advance is 120 m in the strike direction, then the gas pressure and release rate are as shown in Fig. 7.
As shown in Fig. 7, when the mining height of PSNWR is 0.5, 1.0, 1.5, 2.0 and 2.5 (i.e., the mining height of the rock is 0, 0.5, 1.0, 1.5 and 2.0 m), and the mining advance is 120 m in the strike direction, then the gas pressure is 1.05, 0.80, 0.52, 0.26 and 0.12 MPa, and the gas pressure release rate is about 41, 55, 71, 85 and 93 %, respectively. Here, the depth of floor failure is 5.8, 6.4, 8.3, 14.5 and 16.5 m, respectively, as shown in Fig. 8.
As the layer spacing of the Ji15 coal seam and the Ji14 coal seam is 13.0 m, so the depth of floor failure should be close to or greater than 13.0 m, which is beneficial to gas diffusion and drainage. Through the above analysis, to reduce the danger of coal and gas outbursts effectively and improve gas drainage and utilization, the final mining height of the PSNWR is determined to be 2.0 m, namely mining the Ji14 coal seam with a 1.5-m rock layer as a protective seam.
5 Engineering application
5.1 The PSNWR mining process
Combining the mining design from the PSNWR analysis with the characteristics of the distribution and occurrence of the PSNWR and its Protodyakonov coefficient (f ≈ 7–9), the mining process for PSNWR is as follows. When the height of the Ji14 coal seam is >1.4 m, the MG500/1130-WD shearer with ZY6800-12/25D hydraulic supports will be used to mine the PSNWR directly. When the height of the Ji14 coal seam is 1.2–1.4 m, a single row of blasting holes will be used in the advance. The distance between two boreholes is 0.5–0.7 m along the middle of the rock layer and the angle of elevation is 10°. When the height of the Ji14 coal seam is <1.2 m, a double row of blasting holes will be used in the advance. The distance between two boreholes is 0.5–0.7 m, the upper angle of elevation is 15° and the lower angle of depression is 15°–20°, as shown in Fig. 9. After blasting, the MG500/1130-WD shearer with ZY6800-12/25D hydraulic supports will be used to mine.
After mining the PSNWR, the raw coal of the Ji14 coalface will be transported to the coal–gangue separation system underground in the mine using a belt conveyor. The separated gangue will be stored in a gangue bunker. When the Ji15 coal seam is extracted, the separated gangue will be backfilled in the goaf, thus dealing with the gangue (Sun et al. 2015; Gao et al. 2016).
5.2 Gas extraction in PSNWR
During the mining of the PSNWR in the Ji14-31010 coal face, a gob-side entry retaining will be carried out in the lower entry as a return airway. Then by drilling a borehole down and up the PSNWR, buried pipes in the Ji14-31010 gob will be used for gas extraction (Yuan 2015; Lin and Shen 2015). The gas extraction scheme for the PSNWR is shown in Fig. 10.
5.3 Results and discussion
To understand the controlling effect of PSNWR mining on pressure-relief gas drainage, the gas pressure in the Ji15-31010 coal seam and the extracted content of gas were measured during the mining of the PSNWR (from February 20 to March 20, 2013). The results for the gas pressure in the Ji15-31010 coal seam are shown in Fig. 11. The results for the gas content in the Ji15-31010 entries are shown in Fig. 12.
According to Fig. 11, the gas pressure curve can be divided into four stages during the mining advance of 216 m from February 20 to June 20, 2013. In the early stage of PSNWR mining, the influence of mining is slight, the gas pressure is about 1.8 MPa and there is no obvious change compared with the initial state. When the PSNWR coal face is close to the gas pressure monitoring station, the gas pressure increases to 2.08 MPa. Then, the coal seam fractures close, the gas permeability coefficient is low and the gas extraction effect is poorer. As the PSNWR coalface advances, the gas pressure is relieved, the gas drainage rates increase and the residual gas pressure is about 0.22–0.37 MPa, which has dropped by 79.2–87.6 % compared with the initial gas pressure of 1.78 MPa.
According to Fig. 12, in the early stage of PSNWR mining, the gas content of the drained gas is lower in the Ji15-31010 entries, and in the later stage the gas content of the drained gas has obviously increased. The rate of gas drainage can reach about 78 % in the Ji15-31010 head entry, and the borehole residual gas content is 1.91–4.35 m3/t. The rate of gas drainage can reach about 80 % in the Ji15-31010 tail entry, and the borehole residual gas content is 2.26–4.37 m3/t. The results show that mining the PSNWR can effectively eliminate the danger of coal and gas outbursts.
5.4 Gas drainage and utilization
From 2013 to now, the total coal production of PSNWR has been 0.18 million t, and the monthly PSNWR face advance can reach 120 m. During mining of the Ji15-31010 coalface, the separated gangue of PSNWR backfilled in the goaf is about 0.32 million t, and 120 million m3 of gas drainage has been realized. The rate of gas drainage can reach more than 82 %. The Pingdingshan No. 12 Coal Mine has four gas drainage pumping stations and two gas-fired power stations. The generated energy in 2015 was 16.41 million t degrees, saving 10.67 million CNY from the cost of power consumption. In addition, the drained gas can be used as household fuel, for household heating and hot water, as shown in Fig. 13.
6 Conclusions
PSNWR mining as a new technology can effectively eliminate the danger of coal and gas outbursts. It has been successfully applied in Pingdingshan No. 12 Coal Mine, solving the problems of the simultaneous extraction of coal and gas, and there are significant social and economic benefits. The technology has broad potential applicability. The technology and results can be summarized as follows:
-
1.
The distribution and occurrence of the Ji14 coal seam is rather changeable in Pingdingshan No. 12 Coal Mine. The average thickness is 0.5 m, and in most areas there is no coal seam. Mining such a rock–coal seam produces a large amount of waste rock (>80 %). The key parameters of the PSNWR were obtained by experimental tests.
-
2.
A theoretical mechanical model and a three-dimensional numerical model were established to study the controlling effect of PSNWR mining on pressure-relief gas drainage. The mining height of the PSNWR was determined to be 2.0 m, namely mining the Ji14 coal seam with a 1.5-m rock layer as a protective seam.
-
3.
Together with the field application, the mining process and gas drainage design for PSNWR are introduced. The results for Pingdingshan No. 12 Coal Mine show that mining a PSNWR of 2.0 m thickness can effectively reduce the danger of coal and gas outbursts and improve gas drainage and utilization. The gas drainage rates are >80 %, which significantly increases the social, economic and environmental benefits of Pingdingshan No. 12 Coal Mine.
-
4.
There is no appropriate protective coal seam for many coal seams in danger of coal and gas outbursts in China. PSNWR mining is a new technology that can effectively eliminate the danger of coal and gas outbursts, and it has broad potential applicability.
References
Beamish BB, Crosdale JP (1998) Instantaneous outbursts in underground coal mines: an overview and association with coal type. Int J Coal Geol 35:27–55
Brandt J, Sdunowski R (2007) Gas drainage in high efficiency workings in German coal mines. In: Proceeding of 2007’ China (Huainan) international symposium on coal gas control technology. China University of Mining and Technology Press, Xuzhou, pp 22–29
Cheng YP (2010) Mine gas hazard control theory and engineering application. China University of Mining and Technology Press, Xuzhou
Díaz Aguado MB, González Nicieza C (2007) Control and prevention of gas outbursts in coal mines, Riosa–Olloniego coalfield, Spain. Int J Coal Geol 69:236–266
Díaz Aguado María B, González Nicieza C (2007) Control and prevention of gas outbursts in coal mines, Riosa–Olloniego coalfield. Int J Coal Geol 69:253–266
Flores RM (1998) Coalbed methane: from hazard to resource. Int J Coal Geol 35:3–26
Gao R, Zhang JX, Spearing AJS, Li M, An BF, Hao DY (2016) Research into stope roof control of compound roof by solid backfilling mining. Int J Min Sci Technol 26(4):609–614
Hou ZM, Gou Y, Taron J, Gorke UJ, Kolditz O (2012) Thermo-hydro-mechanical modeling of carbon dioxide injection for enhanced gas-recovery (CO2-EGR): a benchmarking study for code comparison. Environ Earth Sci 67:549–561
Itasca Consulting Group Inc (2006) FLAC3D (Fast Lagrangian Analysis of Continua in 3 Dimensions), version 3.1. Itasca Consulting Group Inc, Minneapolis
Lama RD, Bodziony J (1996) Outbursts of Gas, Coal and Rock in Underground Coal Mines. R.D. Lama and Associates, Wollongong, p 499
Li DQ (2014) Mining thin sub-layer as self-protective coal seam to reduce the danger of coal and gas outburst. Nat Hazards 71(1):41–52
Lin BQ, Shen CM (2015) Coal permeability-improving mechanism of multilevel slotting by water jet and application in coal mine gas extraction. Environ Earth Sci 73(10):5975–5986
Lu TK, Yu H, Zhou TY, Mao JS, Guo BH (2009) Improvement of methane drainage in high gassy coal seam using water jet technique. Int J Coal Geol 79:40–48
Ma D, Miao XX, Bai HB, Huang JH, Pu H, Wu Y, Zhang GM, Li JW (2016) Effect of mining on shear sidewall groundwater inrush hazard caused by seepage instability of the penetrated karst collapse pillar. Nat Hazards 82(1):73–93
Meng XR, Xu CH, Gao ZN, Wang XQ (2010) Stress distribution and damage mechanism of mining floor. J China Coal Soc 11:1832–1836
Noack K (1998) Control of gas emissions in underground coal mines. Int J Coal Geol 35:57–82
State Administration of Work Safety (2005) 50 gas treatment experiences. China Coal Industry Publishing House, Beijing
State Administration of Work Safety (2008) Protective mining technical specifications, AQ 1050–2008. China Coal Industry Publishing House, Beijing
State Administration of Work Safety of China (2010) Specification of coal and gas outburst prevention. China Coal Industry, Beijing
Sun Q, Zhang JX, Ju F, Li LY, Zhao X (2015) Research and application of schemes for constructing concrete pillars in large section finishing cut in backfill coal mining. Int J Min Sci Technol 25(6):915–920
Tian KY, Zheng JY (2011) The application of hydraulic fracturing outburst prevention measures. Procedia Eng 26:495–500
Wang HF (2008) Pressure relief functional principle of stope underlying coal–rock mass and application in gas extraction of protected coal seam. D, China University of Mining and Technology
Wang L, Cheng YP, Xu C, An FH, Jin K, Zhang XL (2013) The controlling effect of thick-hard igneous rock on pressure relief gas drainage and dynamic disasters in outburst coal seams. Nat Hazards 66(2):1221–1241
Wang L, Cheng YP, Liu HY (2014) An analysis of fatal gas accidents in Chinese coal mines. Saf Sci 62:107–113
Wei JP, Li B, Wang K, Sun DH (2016) 3D numerical simulation of boreholes for gas drainage based on the pore–fracture dual media. Int J Min Sci Technol 26(4):739–744
Yang W, Lin BQ, Qu YA, Zhao S, Zhai C, Jia LL, Zhao WQ (2011) Mechanism of strata deformation under protective seam and its application for relieved methane control. Int J Coal Geol 85:300–306
Yang HM, Huo XY, Zhang SJ (2012) Study on difference of outburst elimination effect between sub-layers of soft coal and hard coal under the condition of gas per-drainage. Saf Sci 50:768–772
Yang W, Lin BQ, Xu JT (2014) Gas outburst affected by original rock stress direction. Nat Hazards 72:1063–1074
Yu QX, Cheng YP, Jiang CL, Zhou SN (2004) Principles and applications of exploitation of coal and pressure relief gas in thick and high-gas seams. J China Univ Min Technol 33:127–131
Yuan L (2015) Theory and practice of integrated coal production and gas extraction. Int J Coal Sci Technol 2(1):3–11
Zhang Y, Shao JF, Xu WY, Sun HK (2014) Stability analysis of a large landslide in hydropower engineering. Nat Hazards 70(1):527–548
Acknowledgments
This work is supported by State Key Laboratory of Coal Resources and Safe Mining, CUMT (SKLCRSM13X01), Qing Lan Project Foundation of Jiangsu Province (2014) and National Key Basic Research Program of China (2013CB227905). The authors gratefully acknowledge the financial support from the organizations mentioned above.
Author information
Authors and Affiliations
Corresponding authors
Rights and permissions
About this article
Cite this article
Sun, Q., Zhang, J., Zhang, Q. et al. A protective seam with nearly whole rock mining technology for controlling coal and gas outburst hazards: a case study. Nat Hazards 84, 1793–1806 (2016). https://doi.org/10.1007/s11069-016-2512-9
Received:
Accepted:
Published:
Issue Date:
DOI: https://doi.org/10.1007/s11069-016-2512-9