Abstract
Spinal cannabinoid receptor 1 (CB1R) and purinergic P2X receptors (P2XR) play a critical role in the process of pathological pain. Both CB1R and P2XR are expressed in spinal dorsal horn (DH) neurons. It is not clear whether CB1 receptor activation modulates the function of P2X receptor channels within dorsal horn. For this reason, we observed the effect of CP55940 (cannabinoid receptor agonist) on ATP-induced Ca2+ mobilization in cultured rat DH neurons. The changes of intracellular calcium concentration ([Ca2+]i) were detected with confocal laser scanning microscopy using fluo-4/AM as a calcium fluorescent indicator. 100 μM ATP caused [Ca2+]i increase in cultured DH neurons. ATP-evoked [Ca2+]i increase in DH neurons was blocked by chelating extracellular Ca2+ and P2 purinoceptor antagonist PPADS. At the same time, ATP-γ-S (a non-hydrolyzable ATP analogue) mimicked the ATP action, while P2Y receptor agonist ADP failed to evoke [Ca2+]i increase in cultured DH neurons. These data suggest that ATP-induced [Ca2+]i elevation in cultured DH neurons is mediated by P2X receptor. Subsequently, we noticed that, in cultured rat DH neurons, ATP-induced Ca2+ mobilization was inhibited after pretreated with CP55940 with a concentration-dependent manner, which implies that the opening of P2X receptor channels are down-regulated by activation of cannabinoid receptor. The inhibitory effect of CP55940 on ATP-induced Ca2+ response was mimicked by ACEA (CB1R agonist), but was not influenced by AM1241 (CB2R agonist). Moreover, the inhibitory effect of CP55940 on ATP-induced Ca2+ mobilization was blocked by AM251 (CB1 receptor antagonist), but was not influenced by AM630 (CB2 receptor antagonist). In addition, we also observed that forskolin (an activator of adenylate cyclase) and 8-Br-cAMP (a cell-permeable cAMP analog) reversed the inhibitory effect of CP55940, respectively. In a summary, our observations raise a possibility that CB1R rather than CB2R can downregulate the opening of P2X receptor channels in DH neurons. The reduction of cAMP/PKA signaling is a key element in the inhibitory effect of CB1R on P2X-channel-induced Ca2+ mobilization.
Similar content being viewed by others
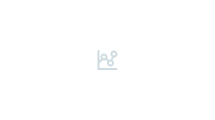
Avoid common mistakes on your manuscript.
Introduction
The endocannabinoid system is composed of two main cannabinoid receptors (CB1 and CB2) and two main classes of endogenous ligands or endocannabinoids. It is reported that CB1 and CB2 receptor are abundantly expressed in the central nervous system, particularly in the cortex, basal ganglia, hippocampus, cerebellum and spinal cord [1, 2]. More and more experimental evidence show that endogenous cannabinoid mechanisms play an important regulatory role in the nociceptive information processing in various areas of the nervous system including the spinal dorsal horn. Farquhar-Smith et al. [3] found that CB1R was strongly expressed in the superficial spinal dorsal horn, including primary afferents and spinal neurons. Immunocytochemical experiments show that more than 30% glutamatergic and approximately 20% GABAergic spinal interneurons is endowed with CB1R, respectively [4]. Intrathecal pretreatment with AM251 (CB1 receptor antagonist) blocked Aβ-fiber stimulation induced- inhibition of the evoked excitatory postsynaptic currents in both excitatory and inhibitory substantia gelatinosa neurons in spinal nerve ligation rats, which implies that activation of CB1R contributes to suppression of spinal nociceptive transmission [5].
On the other hand, more and more evidence suggest that activation of spinal P2X receptors (P2XR) is involved in the induction of nociceptive responses, mechanical hyperalgesia, and the excitation of sensory spinal neurons [6]. It is well known that the fast ionotropic effects are exerted through a family of P2X ATP-gated channels expressed in spinal cord. Presynaptic P2X receptors have been proposed to play a role in modulating glutamate release from the first sensory synapse in the spinal cord [7, 8]. Several ATP receptor subtypes including P2X2, 5, 6 are expressed in spinal dorsal horn neurons [9, 10]. Jo et al. [11] also reported that about 50–60% of dorsal spinal cord neurons possess functional P2X receptors. Increasing evidence suggests that the activation of postsynaptic P2X receptors mediates excitatory transmission within the spinal cord. Patch clamp recording revealed that, in transverse neonatal rat spinal cord slices, ATP-activated excitatory postsynaptic currents are mediated by P2X receptors in a subpopulation of spinal cord lamina II neurons [6]. A further study found that neurons in the superficial layer revealed long-lasting enhancement of depolarization by ATP through P2X receptors during the slow repolarization phase at a single neuron level [10].
It is well known that some different kinds of protein kinases play an important role in the regulation of P2X receptor function. The ATP-induced P2X1 and P2X3 currents are potentiated by an activator of protein kinase C (PKC) [12,13,14]. Protein kinase A (PKA) has been suggested to play a role in the prostaglandin E2-induced potentiation of P2X3R currents in DRG neurons [15]. Our previous studies indicate that corticosterone rapidly inhibits P2X receptors-induced Ca2+ elevation and inward currents through activating PKA in DRG neurons [16, 17]. Han et al. [18] also reported that corticosterone modulated P2X receptor-medicated Ca2+ influx through a membrane-initiated, non-genomic and PKA-dependent pathway in HT4 cells. On the other hand, activation of the Gi/Go-protein-coupled CB1 receptor can reduce PKA activity through reducing the activity of adenylate cyclase [19]. Based on these reports, we hypothesized that CB1R activation could negatively regulate cAMP/PKA signaling and downregulate the opening of the P2X receptor channel in dorsal horn neurons. Therefore, in the present study, we examined whether P2X receptor-induced Ca2+ influx in cultured rat spinal dorsal horn neurons could be influenced after CB1 receptor activation by using confocal laser scanning microscopy.
Materials and Method
Purification and Culture of Dorsal Spinal Cord Neurons
The technique for preparing primary cultures of spinal dorsal horn neurons has been described in detail elsewhere [20]. Briefly, the dorsal one-third of the spinal cord was cut into pieces and incubated in 0.125% trypsin (Sigma) for 25 min at 37 °C in a humidified 5% CO2 −95% air atmosphere. The enzymatic digestion was stopped by adding 3 ml DMEM/F12 containing bovine serum albumin (1 mg/ml; Sigma) and DNase (0.01%; Sigma). The mechanical dissociation was performed by passing through a fire-polished glass pipette. After centrifugation (600 g min for 10 min), the supernatant was removed and replaced with culture medium containing Neurobasal medium (without phenol red and glutamate-free), glutamine (0.5 mM) and B27 (Life Technologies Inc., Gaithersburg, MD). The viability of the dissociated cells was determined by trypan blue exclusion (> 95%). The cells were plated onto sterile glass coverslips pre-coated with 10 μg/ml poly-d-lysine (Sigma) followed by 10 μg/ml Laminin-I (Sigma) and maintained in a 95% air and 5% CO2 humidified incubator at 37 °C for 2–3 days. One day after the cells were seeded, cytosine arabinoside (5 μM) was added to the culture medium for 24 h to reduce glial proliferation. All experiments were performed on neurons after 2–3 days in vitro.
[Ca2+]i Measurement
[Ca2+]i measurement was carried out according to methods previously described [20, 21]. Changes of [Ca2+]i in neurons were detected with confocal laser scanning microscopy using fluo-4/AM (Dojindo, Kumamoto, Japan) as a calcium fluorescent indicator that could monitor real-time alterations of [Ca2+]i. All fluorescence measurements were made from subconfluent areas of the dishes so that individual neurons could be readily identified. Prior to recording, neurons were loaded with the Ca2+ -sensitive fluorescent dye fluo-4/AM (2 μM) for 30 min in a 95% air and 5% CO2 humidified incubator at 37 °C. Subsequently, the coverslips were thoroughly rinsed with D-Hanks medium lacking fluo-4/AM to remove extracellular traces of the dye and to complete de-esterification. Finally, the coverslips were mounted cell-side-up in the free bottom of the chamber and placed on the stage of the confocal microscope. The dye in the selected cytoplasmic part of cells was excited at wavelength 494 nm and fluorescence images were captured at 516 nm at 2 s intervals with an intensified charge-coupled device camera controlled by a computer. All fluorescence measurements were made at room temperature (20–22 °C). Image data were analyzed off-line. The change in [Ca2+]i was represented by relative fluorescence intensity [F1/F0, %] (F0, control; F1, administration of drugs).
Drug Application
ATP, adenosine 5′-O-(3-thiotriphosphate) (ATP-γ-S), pyridoxal phosphate-6-azophenyl-2′,4′ -disulfonic acid (PPADS) and 8-Br-cAMP were made with 0.01 M PBS. CP55940, ACEA, AM1241, AM251, AM630, forskolin and Fluo-4/AM was prepared in 100% DMSO and then diluted to various concentrations with 0.01M PBS. Ca2+ -free solution was made up of 0.2 mM calcium with the addition of 1 mM EGTA (NP-ethyle-neglycoltetraacetic acid). All drugs were added directly to bath solutions. The presence of dimethyl sulfoxide (< 0.1%) alone did not affect the Ca2+ fluorescence intensity. All chemicals except Fluo-4/AM were purchased from Sigma. Fluo-4/AM was purchased from Dojindo, Kumamoto, Japan.
Statistical Analysis
All data were presented as mean ± SD. Statistical analysis was performed with one-way analysis of variance (ANOVA) followed by LSD post hoc test when multiple comparisons were made (SPSS13.0, USA). IC50 was obtained by using GraphPad Prism 4 Software (USA). Differences at the P < 0.05 level were considered statistically significant.
Results
Involvement of P2X Receptor in ATP-Induced [Ca2+]i Increase in Cultured Rat DH Neurons
We observed the effect of 100 μM ATP on [Ca2+]i in cultured DH neurons by using confocal laser scanning microscopy. ATP at 100 μM caused [Ca2+]i increase in approximately 50% of tested neurons. However, ATP can be rapidly degraded into ADP, AMP and adenosine by ectonucleotidases in the extracellular space [22]. It is not clear whether P2X or P2Y receptors are involved in Ca2+ mobilization induced by ATP. Then, ATP-γ-S (a non-hydrolyzable ATP analogue) and ADP (P2Y receptor agonist) were used [23]. We found that ATP-induced [Ca2+]i increase was mimicked by exogenous ATP-γ-S. In a given neuron, the ATP and ATP-γ-S increased Ca2+ fluorescence intensity had similar traces (Fig. 1c, d). On the other hand, no detectable [Ca2+]i increase was observed in neurons stimulated with ADP (Fig. 1c, d). Zeng et al. also reported that ADPbetaS (non-hydrolysable ADP analogue) had no effect on cultured DH neuronal Ca2+ fluorescence intensity [24]. In addition, pretreated with PPADS (the selective antagonist for P2X1, 2, 3, 5, 7 receptor) or chelating extracellular Ca2+ with EGTA eliminated ATP-induced [Ca2+]i responses (Fig. 1a, b, d). It seems that P2X receptor activation is required for ATP-induced Ca2+ response.
The influence of Ca2+-free solution and PPADS on ATP-induced Ca2+ response in cultured DH neurons. a The trace is from a single neuron and shows that 100 μM ATP increased [Ca2+]i in DH neurons, with features of a rapid rise after ATP application (arrow) followed by a slower recovery. Pretreatment with PPADS (50 μM for 10 min) significantly blocked the ATP-induced Ca2+ response. b Ca2+-free solution eliminated [Ca2+]i increase response to ATP. c ATP-γ-S (100 μM), a non-hydrolyzable analog of ATP, mimicked the effect of ATP in DH neurons. In contrast, ADP (100 μM) stimulation failed to induce [Ca2+]i elevation in DH neurons. d A statistical analysis of the influence of Ca2+-free solution and PPADS on ATP -induced [Ca2+]i increase. Data represent means obtained from 3 independent experiments, each including 20–30 cells. *P < 0.01, compared with control; # P < 0.01 compared with ATP
The Effect of CP55940 on ATP-Induced [Ca2+]i Increase in Cultured Rat DH Neurons
In order to identify whether the synthesized CB receptor agonist CP55940 regulates ATP-evoked Ca2+ response, cultured DH neurons were pretreated with CP55940 for 10 min prior to ATP application. CP55940 at 1 μM had no effect on basal Ca2+ fluorescence intensity in neurons at quiescence state. However, as shown in Fig. 2a, b, CP55940 at 1 μM notably decreased ATP-induced [Ca2+]i increase in these neurons. We also noticed that the inhibitory action of CP55940 was concentration-dependent. CP55940 inhibited ATP-induced [Ca2+]i increase to 98.20 ± 9.85, 90.17 ± 8.06, 63.37 ± 3.45, 53.40 ± 2.37% at 1, 10, 100 nM and 1 μM, respectively (Fig. 2c). The IC50 of the CP55940 effect was 35.7 nM.
Concentration-dependent inhibitory effect of CP55940 on ATP-induced Ca2+ response in cultured DH neurons. a Fluorescence images of Ca2+ response from cultured DH neurons. (A1) Control: basal Ca2+ fluorescence intensity in neurons; (A2) The effect of 100 μM ATP on neurons; (A3) The effect of 1 μM CP55940 on ATP-induced [Ca2+]i increase in neurons. b The trace is from a single DH neuron and shows that CP55940 pretreatment (1 μM for 10 min) inhibited ATP-induced [Ca2+]i increase. c CP55940 (0.001–1 μM) inhibited 100 μM ATP-stimulatory action on [Ca2+]i in cultured DH neurons with a dose-dependent manner. *P < 0.05, **P < 0.01, compared with ATP
CP55940 Modulates ATP-Induced [Ca2+]i Increase via CB1 Receptor in Cultured DH Neurons
To find out which type of cannabinoid receptor is responsible for the effect of CP55940 on ATP-induced [Ca2+]i increase, AM251 (selective CB1R antagonist) and AM630 (selective CB2R antagonist) was applied, respectively. According to previous studies, some other off-target effects may prevail if the concentration of CB receptor agonist is greater than 1 μM [25]. We noticed that AM251 and AM630 at 1–10 μM are frequently used in order to establish the involvement of cannabinoid receptor signalling in cellular responses [26, 27]. For this reason, in our experiments, cells were exposed to CP55940 (1 μM) with or without AM251 (10 μM) and AM630 (10 μM) pretreatment, respectively. In the presence of AM251 (10 μM for 10 min), the inhibitory effect of CP55940 on ATP-induced [Ca2+]i elevation was abolished (Figs. 3a, 4). In contrast, the inhibition of CP55940 was not affected by pretreatment with AM630 (10 μM for 10 min) (Figs. 3b, 4). Moreover, the inhibitory action of CP55940 on ATP-induced Ca2+ response was mimicked by selective CB1R agonist ACEA (3 μM for 10 min) (Figs. 3c, 4). Pretreatment with selective CB2R agonist AM1241 (1 μM for 10 min) failed to inhibit ATP-induced Ca2+ response (Figs. 3d, 4). These observations suggest that the effect of CP55940 on ATP-induced Ca2+ response in cultured DH neurons is mediated by CB1R rather than by CB2R.
CP55940 inhibited ATP-induced [Ca2+]i increase via CB1 receptor in cultured DH neurons. a The trace is from a single DH neuron and shows that CB1R antagonist AM251 (10 μM for 10 min) treatment blocked the inhibitory effect of CP55940. b The trace from another DH neuron and shows that CB2R antagonist AM630 (10 μM for 10 min) treatment fails to block the inhibitory effect of CP55940. c The trace shows that CB1R agonist ACEA pretreatment (3 μM for 10 min) inhibited ATP-induced [Ca2+]i increase. d The trace shows that CB2R agonist AM1241 pretreatment (1 μM for 10 min) failed to inhibit ATP-induced [Ca2+]i increase. e The trace shows that forskolin (10 μM, co-incubated with CP55940) completely eliminated the inhibitory effect of CP55940. f The trace shows that 8-Br-cAMP (100 μM, co-incubated with CP55940) markedly suppressed the inhibitory effect of CP55940
The pharmacological profile of the effect of CP55940 on ATP-induced Ca2+ response in cultured DH neurons. CP55940 (1 μM) inhibited ATP-induced Ca2+ elevation in cultured DH neurons. The effect of CP55940 on ATP-induced Ca2+ mobilization was mimicked by CB1R agonist ACEA, but not by CB2R agonist AM1241. In addition, the inhibitory effect of CP55940 on ATP-induced Ca2+ mobilization was blocked by AM251 (CB1 receptor antagonist), but was not influenced by AM630 (CB2 receptor antagonist). Forskolin (an activator of adenylate cyclase) and 8-Br-cAMP (a cell-permeable cAMP analog) reversed the inhibitory effect of CP55940, respectively. Data represent means obtained from 3 independent experiments, each including 20–30 cells. *P < 0.01, compare with ATP; # P < 0.01, compare with CP55940 +ATP
Involvement of cAMP/PKA in the Inhibitory Effect of CP55940 on ATP-Activated [Ca2+]i Elevation
It is reported that binding of cannabinoids to the CB1R suppressed the activity of adenylate cyclase through the intermediacy of the inhibitory Gi/o protein [19]. Subsequently, the fall of intracellular concentration of cAMP may then contribute to decreased PKA protein phosphorylation in many different types of cells [28,29,30]. To investigate a possible role of cAMP/PKA pathway in the effect of CP55940 on the ATP-activated [Ca2+]i increase, forskolin (an activator of adenylate cyclase, 10 μM) and 8-Br-cAMP (cell-permeable cAMP analog, 100 μM) were used. 10 μM forskolin completely eliminated the inhibitory effect of CP55940 on the ATP-evoked Ca2+ responses (Figs. 3e, 4). At the same time, 8-Br-cAMP also reversed the inhibitory effect of CP55940 (Figs. 3f, 4). These results largely confirm that the down-regulation of cAMP/PKA signaling may be involved in the inhibitory effect of CP55940 on ATP-evoked Ca2+ responses in cultured DH neurons.
Discussion
The extracellular mediator ATP, by activating ionotropic P2X and metabotropic P2Y receptors, participates in the generation and modulation of various forms of pain [31,32,33]. It has been postulated that ATP is released from the sensory neurons themselves or from damaged cells and activates various subtypes of P2X and P2Y receptors expressed along the nociceptive pathways [10, 34]. Extracellular ATP increases [Ca2+]i in many different types of cells through binding to purinergic P2X receptor ion channel or G protein-coupled P2Y receptor. P2X activation by ATP always results in rapid influx of extracellular Ca2+ [34]. P2Y receptor-induced [Ca2+]i increase is not dependent upon extracellular Ca2+ and may result from Ca2+ release from an internal store [35]. Agreement with these suggestions, we also found that ATP caused [Ca2+]i increase in approximately 50% of cultured rat DH neurons. Non-hydrolyzable ATP analogue ATP-γ-S mimicked the ATP action, while P2Y receptor agonist ADP had no effect on cultured DH neuronal [Ca2+]i. ATP-evoked Ca2+ response in these neurons was also blocked by chelating extracellular Ca2+. It appears that ATP-induced [Ca2+]i elevation in cultured DH neurons is mediated by P2X receptor. In addition, previous studies suggest that P2Y receptor activation can evoke [Ca2+]i increase in dorsal spinal cord astrocytes and microglia cells in vitro [36, 37]. We also noticed that nearly all cells exhibited Ca2+ mobilization was not stimulated by ADP, which also implies that dorsal spinal cord neurons can be isolated and cultured with high purity in the experiment. It was reported that P2X2, P2X5 and P2X6 subunits are expressed in superficial dorsal spinal cord neurons. Among them, P2X2 subunit is the most abundant in the dorsal horn neurons [9, 10]. Bardoni’s study also shows that the P2X receptors on dorsal horn neurons are nondesensitizing, insensitive to α,β-methylene ATP and sensitivity to the antagonists suramin and PPADS (the selective antagonist for P2X1, 2, 3, 5, 7), which also means that the composition of P2X receptors [6]. In our experiments, PPADS largely blocked the ATP-evoked Ca2+ responses, which presumes that ATP-induced [Ca2+]i elevation in cultured DH neurons is possibly mediated by P2X receptors. Agreement with our suggestion, some previous studies also reported that ATP excites a subset of DH neurons in culture [6, 38] and in spinal cord slices [6, 10, 39] through activation P2X receptors.
Recently, increasing evidence suggests that the dorsal spinal cord is an important site contributing to CB1 and CB2 receptor-mediated analgesia [5, 40, 41, 43]. We noticed that ATP-induced Ca2+ mobilization in cultured DH neurons was inhibited after pretreated with CP55940 with a concentration-dependent manner, which implies that the opening of P2X-channels is down-regulated by the activation of cannabinoid receptors. It is clear that CB1 receptors are expressed in spinal dorsal horn neurons [3,4,5]. In addition, Burston et al. found that the CB2 receptor expression was increased in spinal dorsal horn neurons and microglia cells in a rat osteoarthritis model [40]. However, Romero-Sandoval et al. reported that The CB2R was localized to microglia and perivascular cells at the rat spinal dorsal horn [41]. In this experiment, the inhibitory action of CP55940 was mediated by CB1 receptors since pretreatment with the CB1 receptor antagonist AM251 blocked this effect, while the CB2 receptor antagonist AM630 was ineffective. Earlier data indicate that activation of the CB1 receptors inhibit the slow response to ATP mediated by P2X2 and P2X2/3 receptors in primary sensory neurons [42]. To the further study, CB2R in rat spinal dorsal horn have inhibitory effects in neuropathic, but not sham-operated rats [43]. Moreover, in cultured spinal neurons, CB2 receptor is not involved in AEA (endocannabinoid anandamide)-induced potentiation of Gly receptor-mediated responses [44]. WIN55212-2 suppressed heat-evoked activity and decreased the receptive field areas of isolated rat dorsal horn neurons. Pretreatment with the cannabinoid CB1 receptor antagonists SR141716A or AM251, but not the CB2 antagonist SR144528, blocked the effects [45]. These observations support the notion that, at the spinal cord level, CB2 receptor does not participate in regulation of neuronal function under physiological conditions. In agreement with these suggestions, we also found that, in cultured rat DH neurons, CP55940’s inhibition on ATP-induced Ca2+ response was mimicked by CB1R agonist ACEA, but not by CB2R agonist AM1241. It appears that the inhibitory effect mainly mediated through CB1R rather than CB2R.
As a member of the seven transmembrane domain G-protein-coupled receptors family, CB1R can mediate its effects via activation or inhibition of adenylate cyclase [19, 46]. Inhibition of adenylate cyclase plays an important role in several aspects of cannabinoid function. For example, activation of CB1R can reduce the activity of transient receptor potential vanilloid type 1 in rat cultured primary sensory neurons through inhibiting cAMP/PKA activity [47]. Cannabinoid receptor agonist WIN55212-2, which binds to the CB1R, inhibits ATP-activated currents in rat trigeminal ganglionic neurons via inhibition of the AC-cAMP-PKA signaling pathway [48]. In the present study, the addition of adenylate cyclase activator and cAMP analog reversed the inhibition of CP55940 on ATP-induced Ca2+ mobilization in cultured DH neurons. It is reasonable to deduce that the corresponding intracellular signal transduction pathway is as follows: CP55940, after binding to the CB1 receptors, activates Gi which inhibits the activity of AC and results in the reduction of cAMP levels and PKA activity. The inhibition of CP55940 on ATP-induced Ca2+ mobilization may result from decreased phosphorylation of P2X receptor by CB1R-induced reduction of PKA activity.
P2X receptors have been shown to be regulated by both PKA and PKC activation, which suggests that they might be substrates for phosphorylation [49]. Chow et al. report that the intracellular carboxyl terminus of P2X2 receptor contains several consensus phosphorylation sites for cAMP-dependent PKA, suggesting that the function of the P2X2 purinoceptor could be regulated by the protein phosphorylation [50]. It was reported that CB1R activation inhibited forskolin-stimulated cAMP accumulation in spinal locomotor networks and hippocampal slices [51, 52]. In the present study, in cultured dorsal spinal cord neurons, CB1R activation significantly suppressed P2X receptor-evoked Ca2+ mobilization through down-regulating cAMP/PKA signaling pathway, which also strongly supports the notion that cAMP/PKA is an important downstream targets for the CB1 receptor signaling. In addition, we suspect that those neurotransmitter receptors contain several consensus phosphorylation sites for cAMP-dependent PKA can be modulated by CB1 receptor through the tuning down of PKA activity. For example, Roscioni et al. found that the PKA activator 6-Bnz-cAMP and the Epac activator 8-pCPT-2′-O-Me-cAMP significantly increased bradykinin-induced IL-8 release, which means that bradykinin receptor may have phosphorylation sites for cAMP-dependent PKA [53]. Moreover, ACEA (selective CB1 receptor agonist) reduced the mechanical hyperalgesia induced by bradykinin [54]. In the present study, CB1 receptor activation can effectively suppress ATP-induced [Ca2+]i increase mediated by P2X receptor in cultured dorsal spinal cord neurons. The inhibition of CP55940 on ATP-induced Ca2+ mobilization may result from decreased phosphorylation of P2X receptor by CB1R-induced reduction of PKA activity. In additon, Walter et al. report that ATP-induced 2-Arachidonoylglycerol (2-AG, an endogenous agonist of the cannabinoid receptor) production is mainly regulated by P2X7 receptor in cultured mouse astrocytes [55]. Witting et al. suggest that ionotropic, and not metabotropic, purinergic receptors control 2-AG production in cultured microglial cells [56]. In the present study, activation of CB1 receptor can effectively suppress ionotropic P2X receptor-induced [Ca2+]i increase in cultured dorsal spinal cord neurons. Furthermore, in the primary afferent neurons, the analgesic effect of CB1 receptor activation is mediated by a negative modulation of the P2X3 receptor [54]. Based on these reports, we hypothesized that whether CB1R-mediated reduction in P2XR function is a feed-back mechanism to down-regulate 2-AG release in the CNS. It looks likely that P2XR signaling may lead to 2-AG production, which in turn may decrease the neuronal excitability and synaptic strength under pathological circumstances. However, the next important question, whether glial-derived 2-AG decreases neuronal excitability and synaptic strength under pathological circumstances in situ, awaits resolution.
References
Woodhams SG, Sagar DR, Burston JJ, Chapman V (2015) The role of the endocannabinoid system in pain. Handb Exp Pharmacol 227:119–143
Ulugöl A (2014) The endocannabinoid system as a potential therapeutic target for pain modulation. Balkan Med J 31:115–120
Farquhar-Smith WP, Egertová M, Bradbury EJ, McMahon SB, Rice AS, Elphick MR (2000) Cannabinoid CB(1) receptor expression in rat spinal cord. Mol Cell Neurosci 15:510–521
Hegyi Z, Kis G, Holló K, Ledent C, Antal M (2009) Neuronal and glial localization of the cannabinoid-1 receptor in the superficial spinal dorsal horn of the rodent spinal cord. Eur J Neurosci 30: 251–262
Yang F, Xu Q, Shu B, Tiwari V, He SQ, Vera-Portocarrero LP, Dong X, Linderoth B, Raja SN, Wang Y, Guan Y (2016) Activation of cannabinoid CB1 receptor contributes to suppression of spinal nociceptive transmission and inhibition of mechanical hypersensitivity by Aβ-fiber stimulation. Pain 157(11):2582–2593
Bardoni R, Goldstein PA, Lee CJ, Gu JG, MacDermott AB (1997) ATP P2Xreceptors mediate fast synaptic transmission in the dorsal horn of the rat spinal cord. J Neurosci 17:5297–5304
Gu JG, MacDermott AB (1997) Activation of ATP P2X receptors elicits glutamate release from sensory neuron synapses. Nature 389:749–753
Nakatsuka T, Gu JG (2001) ATP P2X receptor-mediated enhancement of glutamate release and evoked EPSCs in dorsal horn neurons of the rat spinal cord. J Neurosci 21(17):6522–6531
Collo G, North RA, Kawashima E, Merlo-Pich E, Neidhart S, Surprenant A, Buell G (1996) Cloning of P2X5 and P2X6 receptors and the distribution and properties of an extended family of ATP-gated ion channels. J Neurosci 16:2495–2507
Aoyama R, Okada Y, Yokota S, Yasui Y, Fukuda K, Shinozaki Y, Yoshida H, Nakamura M, Chiba K, Yasui Y, Kato F, Toyama Y (2011) Spatiotemporal and anatomical analyses of P2X receptor-mediated neuronal and glial processing of sensory signals in the rat dorsal horn. Pain 152:2085–2097
Jo YH, Schlichter R (1999) Synaptic corelease of ATP and GABA in cultured spinal neurons. Nat Neurosci 2:241–245
Paukert M, Osteroth R, Geisler HS, Brandle U, Glowatzki E, Ruppersberg JP, Gründer S (2001) Inflammatory mediators potentiate ATP-gated channels through the P2X(3) subunit. J Biol Chem 276(24):21077–21082
Vial C, Tobin AB, Evans RJ (2004) G-protein-coupled receptor regulation of P2X1 receptors does not involve direct channel phosphorylation. Biochem J 382(Pt 1):101–110
Brown DA, Yule DI (2007) Protein kinase C regulation of P2X3 receptors is unlikely to involve direct receptor phosphorylation. Biochim Biophys Acta 1773(2):166–175
Wang C, Gu Y, Li GW, Huang LY (2007) A critical role of the cAMP sensor Epac in switching protein kinase signalling in prostaglandin E2-induced potentiation of P2X3 receptor currents in inflamed rats. J Physiol 584(Pt 1):191–203
Liu XH, Zeng JW, Zhao YD, Chen PH, Xiao Z, Ruan HZ (2008) Rapid inhibition of ATP-induced currents by corticosterone in rat dorsal root ganglion neurons. Pharmacology 82(2):164–170
Liu XH, Zeng JW, Zhao YD, Xiao Z, Fang CQ, Ruan HZ (2010) Inhibition of ATP-induced Ca2+ influx by corticosterone in dorsal root ganglion neurons. Neurochem Res 35(5):804–810
Han JZ, Lin W, Chen YZ (2005) Inhibition of ATP-induced calcium influx in HT4 cells by glucocorticoids: involvement of protein kinase A. Acta Pharmacol Sin 26(2):199–204
Dalton GD, Bass CE, Van Horn C, Howlett AC (2009) Signal transduction via cannabinoid receptors. CNS Neurol Disord Drug Targets 8:422–431
Jo YH, Stoeckel ME, Freund-Mercier MJ, Schlichter R (1998) Oxytocin modulates glutamatergic synaptic transmission between cultured neonatal spinal cord dorsal horn neurons. J Neurosci 18:2377–2386
Gee KR, Brown KA, Chen WN, Bishop-Stewart J, Gray D, Johnson I (2000) Chemical and physiological characterization of fluo-4 Ca2+-indicator dyes. Cell Calcium 27:97–106
Lai KM, Wong PC (1991) Metabolism of extracellular adenine nucleotides by cultured rat brain astrocytes. J Neurochem 57(5):1510–1515
Prendergast J, Umanah GK, Yoo SW, Lagerlöf O, Motari MG, Cole RN, Huganir RL, Dawson TM, Dawson VL, Schnaar RL (2014) Ganglioside regulation of AMPA receptor trafficking. J Neurosci 34(39):13246–13258
Zeng JW, Liu XH, Zhao YD, Xiao Z, He WJ, Hu ZA, Ruan HZ (2009) Role of P2Y1 receptor in neuron-to-glia signaling at dorsal spinal cord. J Neurosci Res 87(12):2667–2676
Solymosi K, Köfalvi A (2017) Cannabis: a treasure trove or pandora’s box? Mini Rev Med Chem 17(13):1223–1291
Tomiyama K, Funada M (2014) Cytotoxicity of synthetic cannabinoids on primary neuronal cells of the forebrain: the involvement ofcannabinoid CB1 receptors and apoptotic cell death. Toxicol Appl Pharmacol 274(1):17–23
Grabiec U, Koch M, Kallendrusch S, Kraft R, Hill K, Merkwitz C, Ghadban C, Lutz B, Straiker A, Dehghani F (2012) The endocannabinoid N-arachidonoyldopamine (NADA) exerts neuroprotective effects after excitotoxic neuronal damage via cannabinoid receptor 1 (CB(1)). Neuropharmacology 62(4):1797–1807
Felder CC, Joyce KE, Briley EM, Glass M, Mackie KP, Fahey KJ, Cullinan GJ, Hunden DC, Johnson DW, Chaney MO, Koppel GA, Brownstein M (1998) LY320135, a novel cannabinoid CB1 receptor antagonist, unmasks coupling of the CB1 receptor to stimulation of cAMP accumulation. J Pharmacol Exp Ther 284:291–297
Glass M, Felder C (1997) Concurrent stimulation of cannabinoid CB1 and dopamine D2 receptors augments cAMP accumulation in striatal neurons: evidence for a G s linkage to the CB1 receptor. J Neurosci 17:5327–5333
McAllister SD, Glass M (2002) CB(1) and CB(2) receptor-mediated signalling: a focus on endocannabinoids. Prostaglandins Leukot Essent Fat Acids 66:161–171
Burnstock G (2006) Purinergic P2 receptors as targets for novel analgesics. Pharmacol Ther 110(3):433–454
Donnelly-Roberts DL, Jarvis MF (2007) Discovery of P2X7 receptor selective antagonists offers new insights into P2X7 receptor function and indicates a role in chronic pain states. Br J Pharmacol 151(5):571–579
Andó RD, Méhész B, Gyires K, Illes P, Sperlágh B (2010) A comparative analysis of the activity of ligands acting at P2X and P2Y receptor subtypes in models of neuropathic, acute and inflammatory pain. Br J Pharmacol 159(5):1106–1117
Dunn PM, Zhong Y, Burnstock G (2001) P2X receptors in peripheral neurons. Prog Neurobiol 65:107–134
Ralevic V, Burnstock G (1998) Receptors for purines and pyrimidines. Pharmacol Rev 50:413–492
Zeng JW, Liu XH, Zhang JH, Wu XG, Ruan HZ (2008) P2Y1 receptor-mediated glutamate release from cultured dorsal spinal cord astrocytes. J Neurochem 106(5):2106–2118
Zeng J, Wang G, Liu X, Wang C, Tian H, Liu A, Jin H, Luo X, Chen Y (2014) P2Y13 receptor-mediated rapid increase in intracellular calcium induced by ADP in cultured dorsal spinal cord microglia. Neurochem Res 39(11):2240–2250
Jahr CE, Jessell TM (1983) ATP excites a subpopulation of rat dorsal horn neurones. Nature 304(5928):730–733
Li J, Perl ER (1995) ATP modulation of synaptic transmission in the spinal substantia gelatinosa. J Neurosci 15:3357–3365
Burston JJ, Sagar DR, Shao P, Bai M, King E, Brailsford L, Turner JM, Hathway GJ, Bennett AJ, Walsh DA, Kendall DA, Lichtman A, Chapman V (2013) Cannabinoid CB2 receptors regulate central sensitization and pain responses associated with osteoarthritis of the knee joint. PLoS ONE 8(11):e80440
Romero-Sandoval A, Nutile-McMenemy N, DeLeo JA (2008) Spinal microglial and perivascular cell cannabinoid receptor type 2 activation reduces behavioralhypersensitivity without tolerance after peripheral nerve injury. Anesthesiology 108(4):722–734
Krishtal O, Lozovaya N, Fedorenko A, Savelyev I, Chizhmakov I (2006) The agonists for nociceptors are ubiquitous, but the modulators are specific: P2X receptors in the sensory neurons are modulated by cannabinoids. Pflugers Arch 453:353–360
Sagar DR, Kelly S, Millns PJ, O’Shaughnessey CT, Kendall DA, Chapman V (2005) Inhibitory effects of CB1 and CB2 receptor agonists on responses of DRG neurons and dorsal horn neurons in neuropathic rats. Eur J Neurosci 22(2):371–379
Xiong W, Wu X, Li F, Cheng K, Rice KC, Lovinger DM, Zhang L (2012) A common molecular basis for exogenous and endogenous cannabinoid potentiation of glycinereceptors. J Neurosci 32(15):5200–5208
Liu C, Walker JM (2006) Effects of a cannabinoid agonist on spinal nociceptive neurons in a rodent model of neuropathic pain. J Neurophysiol 96(6):2984–2994
Chen XP, Yang W, Fan Y, Luo JS, Hong K, Wang Z, Yan JF, Chen X, Lu JX, Benovic JL, Zhou NM (2010) Structural determinants in the second intracellular loop of the human cannabinoid CB1 receptor mediate selective coupling to G(s) and G(i). Br J Pharmacol 161:1817–1834
Sántha P, Jenes A, Somogyi C, Nagy I (2010) The endogenous cannabinoid anandamide inhibits transient receptor potential vanilloid type 1 receptor-mediated currents in rat cultured primary sensory neurons. Acta Physiol Hung 97:149–158
Shen JJ, Liu CJ, Li A, Hu XW, Lu YL, Chen L, Zhou Y, Liu LJ (2007) Cannabinoids inhibit ATP-activated currents in rat trigeminal ganglionic neurons. Sheng Li Xue Bao 59(6):745–752
Brown David A., David I (2010) Yule protein kinase A regulation of P2X4 receptors: requirement for a specific motif in the C-terminus. Biochim Biophys Acta 1803(2):275–287
Chow YW, Wang HL (1998) Functional modulation of P2X2 receptors by cyclic AMP-dependent protein kinase. J Neurochem 70(6):2606–2612
Serpa A, Correia S, Ribeiro JA, Sebastião AM, Cascalheira JF (2015) The combined inhibitory effect of the adenosine A1 and cannabinoid CB1 receptors on cAMP accumulation in the hippocampus is additive and independent of A1 receptor desensitization. Biomed Res Int. doi:10.1155/2015/872684
Veeraraghavan P, Nistri A (2015) Modulatory effects by CB1 receptors on rat spinal locomotor networks after sustained application of agonists or antagonists. Neuroscience 303:16–33
Roscioni SS, Kistemaker LE, Menzen MH, Elzinga CR, Gosens R, Halayko AJ, Meurs H, Schmidt M (2009) PKA and Epac cooperate to augment bradykinin-induced interleukin-8 release from human airway smooth muscle cells. Respir Res 10:88
Oliveira-Fusaro MC, Zanoni CI, Dos Santos GG, Manzo LP, Araldi D, Bonet IJ, Tambeli CH, Dias EV, Parada CA (2017) Antihyperalgesic effect of CB1 receptor activation involves the modulation of P2X3 receptor in the primary afferent neuron. Eur J Pharmacol 798:113–121
Walter L, Dinh T, Stella N (2004) ATP induces a rapid and pronounced increase in 2-arachidonoylglycerol production by astrocytes, a response limited by monoacylglycerol lipase. J Neurosci 24(37):8068–8074
Witting A, Walter L, Wacker J, Möller T, Stella N (2004) P2X7 receptors control 2-arachidonoylglycerol production by microglial cells. Proc Natl Acad Sci USA 101(9):3214–3219
Acknowledgements
This work is supported by the National Natural Science Foundation of China (No. 31360253). We are very grateful to the other staff of Department of Physiology. We also thank Guo Luo (Central Laboratory, Zunyi Medical College) for his technique assistance in laser scanning confocal microscopy.
Author information
Authors and Affiliations
Corresponding author
Rights and permissions
About this article
Cite this article
Long, J., Lei, X., Chen, M. et al. CB1 Receptors Mediated Inhibition of ATP-Induced [Ca2+]i Increase in Cultured Rat Spinal Dorsal Horn Neurons. Neurochem Res 43, 267–275 (2018). https://doi.org/10.1007/s11064-017-2414-6
Received:
Revised:
Accepted:
Published:
Issue Date:
DOI: https://doi.org/10.1007/s11064-017-2414-6