Abstract
Hesperidin is a flavonoid glycoside that is frequently found in citrus fruits. Our group have demonstrated that hesperidin has neuroprotective effect in 6-hydroxydopamine (6-OHDA) model of Parkinson’s disease (PD), mainly by antioxidant mechanisms. Although the pathophysiology of PD remains uncertain, a large body of evidence has demonstrated that mitochondrial dysfunction and apoptosis play a critical role in dopaminergic nigrostriatal degeneration. However, the ability of hesperidin in modulating these mechanisms has not yet been investigated. In the present study, we examined the potential of a 28-day hesperidin treatment (50 mg/kg/day, p.o.) in preventing behavioral alterations induced by 6-OHDA injection via regulating mitochondrial dysfunction, apoptosis and dopaminergic neurons in the substantia nigra pars compacta (SNpc) in C57BL/6 mice. Our results demonstrated that hesperidin treatment improved motor, olfactory and spatial memory impairments elicited by 6-OHDA injection. Moreover, hesperidin treatment attenuated the loss of dopaminergic neurons (TH+ cells) in the SNpc and the depletion of dopamine (DA) and its metabolities 3,4-dihydroxyphenylacetic acid (DOPAC) and homovanillic acid (HVA) in the striatum of 6-OHDA-lesioned mice. Hesperidin also protected against the inhibition of mitochondrial respiratory chain complex-I, -IV and V, the decrease of Na + -K + -ATPase activity and the increase of caspase-3 and -9 activity in the striatum. Taken together, our findings indicate that hesperidin mitigates the degeneration of dopaminergic neurons in the SNpc by preventing mitochondrial dysfunction and modulating apoptotic pathways in the striatum of 6-OHDA-treated mice, thus improving behavioral alterations. These results provide new insights on neuroprotective mechanisms of hesperidin in a relevant preclinical model of PD.
Similar content being viewed by others
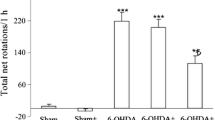
Avoid common mistakes on your manuscript.
Introduction
Parkinson’s disease (PD) is the second most common age-related neurodegenerative disorder, after Alzheimer’s disease. PD is characterized by a progressive degeneration of dopamine (DA) neurons in the substantia nigra pars compacta (SNpc) and attendant projections to striatum (Kalia and Lang 2015). The loss of dopaminergic neurons and consequent DA deficiency within the basal ganglia culminates in a movement disorder characterized by classical parkinsonian motor symptoms, such as resting tremor, bradykinesia, rigidity and postural instability (Balestrino and Martinez-Martin 2017). PD is also associated with non-motor symptoms, some of which occurs many years pior to motor dysfunction (Kalia and Lang 2015). Research has been recently concentrated on these symptoms (e.g. depression, anxiety, memory deficits and olfactory dysfunction), which cause severe negative impact on patient’s quality of life and caregiver’s burden (Chaudhuri and Schapira 2009; Yang et al. 2009).
Although the pathogenesis of PD is still unknown, converging evidence suggest that mitochondrial dysfunction, oxidative stress and apoptosis are important mechanisms involved in dopaminergic neuron degeneration (David Adams Jr 2012; Perier et al. 2007). Injection of catecholamine neurotoxin 6-hydroxydopamine (6-OHDA) into the striatum, a well-recognized experimental model for the study of PD, produces degeneration of nigrostriatal dopamine neuron system. 6-OHDA selectively destroys dopamine neurons in the SNpc by inducing oxidative stress, neuroinflammation and apoptosis (Hernandez-Baltazar et al. 2017).
Since the available pharmachological treatments of PD only provide symptomatic relief, the development of disease-modifying treatments is a challenge for PD therapeutics. In this context, experimental and clinical studies have sought to investigate the effects of new compounds that do not have the side effects of current PD drugs (Carabelas 2016). Natural products, especially plant polyphenols, have attracted progressively more attention as non-pharmacological interventions to modulate neuronal function and reduce the symptomatology of neurodegenerative diseases (Abushouk et al. 2017; Antunes et al. 2014). It has been reported that neuroprotective actions of polyphenols include a number of mechanisms whitin the brain, demonstrating a potential to protect neurons against the injury induced by neurotoxins (Vauzour et al. 2008).
In this context, hesperidin (4′-methoxy-7-O-rutinosyl-3′,5-dihydroxyflavanone), a specific flavonoid glycoside that is frequently found in citrus fruits, has been described to possess significant antioxidant, anti-inflammatory, antiviral, anticancer, and antidepressant-like properties in mice (Garg et al. 2001; Gaur and Kumar 2010). For instance, we have previously shown that hesperidin exerts therapeutic effects in a mouse model of 6-OHDA, mainly by its antioxidant activity. However, there is a paucity of studies regarding the ability of hesperidin in modulating critical mechanisms involved in the neurodegeneration of PD, such as mitochondrial dysfunction and apoptosis.
Thus, the present study was carried out to investigate the potential of hesperidin treatment for 28 days in a mouse model of PD induced by intrastriatal injection of 6-OHDA. In order to elucidate the possible protective mechanisms of hesperidin against the dopaminergic nigrostriatal impairment induced by 6-OHDA, we evaluated the markers of mitochondrial dysfunction and apoptosis in the striatum of mice. In addition, we analyzed whether these neurobiological mechanisms are accompanied by an improvement in cognitive, motor and olfactory impairments evoked by 6-OHDA injection.
Materials and methods
Animals
The experiments were conducted using male C57BL/6 mice (25–35 g, 3–6 months old). Animals were maintained at constant room temperature (21 ± 1 °C) with free access to water and food, under a 12:12 h light:dark cycle (lights on at 07:00 h) and the manipulations were carried out during the light portion of the cycle. The procedures of this study were conducted according to the guidelines of the Committee on Care and Use of Experimental Animals Resources and with the approval of Ethical Committee for Animal Use (CEUA protocol #002/2013) of the Federal University of Pampa, Brazil. All efforts were made to minimize animal suffering and to reduce the number of animals used.
Reagents
All reagents used in this study were purchased from Sigma Chemical Co. (St. Louis, MO, USA).
Experimental design
The mice were submitted to stereotaxic surgical to inject 6-OHDA or vehicle into the striatum. Seven days after the microinjections, treatment with oral hesperidin (50 mg/kg) was initiated and continued for 28 days, as previously described by us (Antunes et al. 2014, 2016). Mice were randomly assigned into four groups (n = 6–8 per group; total number = 32): (I) sham-operated/saline (sham/vehicle) as the control group; (II) sham-operated/hesperidin treated mice (sham/hesperidin); (III) 6-OHDA-mice treated with saline (6-OHDA/vehicle); (IV) 6-OHDA-mice treated with hesperidin. After the finish of treatments, the mice were submitted to behavior tests and the striatum was removed for neurochemical assays. All analyses were performed in a blinded fashion (Fig. 1).
Stereotaxic surgical injection of 6-OHDA
Surgery was performed under anesthesia with 10 ml/kg of 1% ketamine and 0.2% xylazine. 6-OHDA (5 μg in 2 μL of 0.9% NaCl with 0.2 μg/mL ascorbic acid) was injected slowly (0.5 μl/min) into the right striatum (0.9 mm anterior and 1.8 mm lateral from bregma, 3.0 mm ventral from the dura) as described previously (Antunes et al. 2014; Goes et al. 2014, 2018). After the injection, the syringe was maintained in the brain for an additional 3 min before it was slowly retracted. Controls were injected with the vehicle. Following surgery, the scalp was sutured and gel lidocaine (2%) was administered for post-operative analgesia. Subcutaneous sterile saline was given to aid in post-operative recovery. All animals were then placed in clean cages on a heated pad for recovery. Postoperatively and on the following 3 days mice were monitored closely for any signs of pain and discomfort.
Behavioral assessment
Open field test (OFT)
To verify the effects of hesperidin and 6-OHDA administration on locomotor activity, the animals were submitted individually to a 5 min OFT (Insight model EP 154C) 24 h after the last treatment. The apparatus was cleaned with an ethanol solution (10% v/v) and dried with paper towels after each trial in order to prevent odors from carrying-over between trials. The parameter observed was the total distance traveled (unit: mm) (Prut and Belzung 2003).
Rotational behavior
Twenty-eight days after surgery, animals were tested for rotational behavior after receiving an intraperitoneal bolus injection of apomorphine hydrochloride (0.6 mg/kg; from Sigma-Aldrich). Rotational testing was conducted following the methodology initially described by Ungerstedt (1971). Briefly, animals were placed inside a cylindrical container (33 cm diameter and 35 cm height) and contralateral rotations (the number of 360° contralateral turns) were counted for 60 min in a quiet isolated room.
Olfactory discrimination task (ODT)
The olfactory discrimination ability was assessed using a protocol previously described by Prediger et al. (2010). This task is based on the fact that rodents usually disclose preference for places impregnated with their own odor (familiar compartments) than places with other non-familiar odors. In short, each mouse was placed for 5 min in a cage divided in two equal areas separated by an open door, where it could choose between one compartment with fresh sawdust (non-familiar compartment) and another with unchanged sawdust (familiar compartment) that the same mice had occupied for 3 days before the test. Each animal was initially placed in the center of the non-familiar compartment, and the time spent by the mice in both compartments (familiar vs. non-familiar) was recorded. Generally, mature male mice are able to discriminate between the familiar and the non-familiar compartments, spending much more time in the familiar compartment since they significantly prefer their own odor. The results were expressed as the percentage of time the mice remained in the familiar compartment.
Barnes maze (BM)
Spatial learning and working memory were evaluated using the Barnes maze (Walker et al. 2011). The maze consists of a circular platform (92-cm diameter) surrounded by 20 holes (5-cm diameter, equidistant), one providing an escape route. The mice were placed in a dark chamber in the center of the maze and aversive stimuli subsequently triggered (bright light and noise). The animal was then given the opportunity to leave the maze by crawling through the hole allowing the escape. In the spatial acquisition phase, animals were subjected to four trials per day for four consecutive days, with a constant inter-trial interval time of 20 min. The number of holes explored and the time required for the mice to locate the escape hole were quantified. For the reference memory phase or probe trials, animals were tested on the 5th day (short-term retention) and 12th day (long-term retention) following the first trials, omitting training sessions between test trials. During the probe trials, the escape route was removed and replaced with a standard hole through which the animal could not enter. The mice were tested during a 90-s period. The latency to reach the virtual target hole and number or erros were recorded, the outcome measure corresponding to the spatial memory capacity of the animal.
Tissue preparation
After behavioral tests, mice were euthanized with a barbiturate overdose (intraperitoneal pentobarbital sodium 150 mg/kg). The striatum was removed and rapidly homogenized in 50 mM Tris-Cl, pH 7.4. The homogenate was centrifuged at 2400 g for 15 min at 4 °C, and a low-speed supernatant fraction (S1) was used for assays.
Biochemical determinations
Immunohistochemistry of tyrosine hydroxylase (TH)
Design-based stereology was performed for cell counts and volume measurements of TH-positive neurons in substantia nigra pars compacta (SNpc), using a Leicamicroscopy (DMR 6000) coupled with stereological system newCast (Visiopharm version 4.5.6.857), and a video-camera Olympus DP72. The regions of interest (SNpc) were delineated according to Paxinos and Franklin (2001) and Oorschot (1996) by anatomical landmarks (Bregma−4.70 to −6.30 mm) with a 2,5× magnification on live microscopic video images displayed on a monitor.
The SNpc TH-positive neurons were counted by the optical fractionator design (Gundersen et al. 1988; Gundersen 2002; Schmitz and Hof 2005). Briefly, the prefixed brains were embedded in 8% agar solution and cut exhaustively in coronal sections by a vibrotome (Leica VT1000S) in 40-μm-thick sections, cover all region of interest, along the frontal-caudal axis. Every fifth serial section containing SNpc was selected, and then we obtained the section sampling fraction (ssf = 1/5). In this sample sections were applied free-floating immunohistochemical methods to identify the dopaminergic neurons in SNpc. So, the sections were washed with PBS, incubated with a 0.3% Triton X-100 solution, exposed to 0.3% hydrogen peroxide in distilled water to block endogenous peroxidases, placed in a 10% non-immune normal goat serum (Jackson Immuno Research Labs), incubated with a primary antibody (rabbit anti-Tirosinehidroxilase, 1:1000, Abcam) and with a secondary antibody (anti-rabbit IgG peroxidase conjugate, 1:200, KPL) in PBS. Immunoreactivity was visualized with 3,3-diaminobenzidine in PBS containing 0.01% hydrogen peroxide, and then the sections were mounted in a glass slide and cover with a coverslip.
In each sampled section some unbiased counting frames were created by the software and randomly placed over the region of interest. The counting frames were replaced systematically by stepwise movements in x- and y-directions. The area of the unbiased counting frame (a (frame) = 6400 μm2) relative to the area associated with the x and y steps (step length = 70,000 μm) gives the area sampling fraction (asf = 1/11). The optical disector height, along the z-axis, was determined by excluding an up guard region and a bottom guard region. The height of the optical disector relative to the actual thickness of the section results in the height sampling fraction (hsf = 20). Only counting frames for which at least a part of the frame fell within the delineated contour were used for counting. Cells were marked if they were positive and in focus within the counting area. Furthermore, the counting frames have forbidden lines to avoid edge effects.
The estimated total number of positive cells (N) was calculated from the number of TH-neurons counted according to the formula:
Where Q- is the TH-positive neurons selected by the optical disector, ssf is the section sampling fraction; asf is the area sampling faction (a (frame) / step length) and hsf is the high sampling fraction.
Determination of monoamine levels
The measurement of DA and 3,4-dihydroxyphenylacetic acid (DOPAC) in striatal tissue was carried out by HPLC, as previously described (Goes et al. 2014). Tissue homogenate (10% w/v) was sonicated in 0.1 M HClO4 for 30 s, centrifuged at 4 °C for 15 min at 15,000 rpm, and the supernatant was filtered (0.2 μm, Millipore). A 20-μl sample was then injected into the C-18 HPLC column. The mobile phase was 0.163 M citric acid (pH 3.0), containing 0.02 mM NaCl with 0.69 mM sodium octanesulfonic acid as the ion pairing reagent, 4% v/v acetonitrile and 1.7% v/v tetrahydrofuran. DA and DOPAC were electrochemically detected, using an amperometric detector (Shimadzu, Japan). The amount of monoamines was determined by comparison with freshly prepared standards, and their concentrations were expressed as ng/mg of tissue.
Mitochondria isolation
Mitochondria from mouse brain striatum were isolated by following standard protocol (Pedersen et al. 1978). The mitochondrial protein content was estimated using the method of Lowry et al. (1951).
Estimation of NADH dehydrogenase (complex-I) activity
NADH dehydrogenase activity was measured by catalytic oxidation of NADH with potassium ferricyanide as an artificial electron acceptor at excitation (350 nm) and emission (470 nm) wavelengths for NADH (Shapiro et al. 1979). The reaction mixture consisted of 200 μl of 10 mM potassium ferricyanide, 60 μl of 1 mM NADH in 2 mM potassium phosphate buffer, and 2.64 ml of 0.12 M potassium phosphate buffer. Activity of NADH dehydrogenase was expressed as nmol NADH oxidized/min/mg protein.
Estimation of succinate dehydrogenase (complex-II) activity
The mitochondrial succinate dehydrogenase (SDH) was determined by the progressive reduction of nitro blue tetrazolium (NBT) to an insoluble colored compound, diformazan (dfz), which was measured at 570 nm (Storrie and Madden 1990). Briefly, the reaction mixture consisted of 1.5 ml of phosphate buffer (0.2 M, pH 7.8), 0.2 ml of succinic acid (0.6 M, pH 7.8), 0.3 ml of BSA (1%, w/v), and 0.1 ml of 0.03 M potassium ferricyanide and the reaction was started by addition of mitochondrial preparation (30 μl). The SDH activity was expressed as micromole formazan produced/min/mg protein.
Estimation of cytochrome-C oxidase (complex-IV) activity
Complex-IV activity was measured in mitochondrial preparation according by Storrie and Madden (1990). Briefly, first the cytochrome c was reduced by the addition of a few crystals of sodium borohydride and then neutralized to pH 7.0 by 0.1 M HCl. The reduced cytochrome c (0.3 mM) was added to 0.075 M phosphate buffer (pH 7.4) and the reaction was initiated by addition of appropriate amount of mitochondrial suspension (30 μl). The decrease in absorbance was measured at 550 nm for 3 min. Results were expressed as nmol cytochrome-c oxidized/min/mg protein (ε550 = 19.6 mmol−1 cm−1).
Estimation of F1F0 ATP synthase (complex-V) activity
Mitochondrial F1F0 synthase was measured according to Griffiths and Houghton (1974). Briefly, mitochondrial suspension was incubated in 500 ml of ATPase buffer (50 mM Tris HCl and 5 mM MgCl2, pH 7.5) at 37 °C with 5 mM ATP for 10 min. The reaction was stopped by adding 500 ml of 10% (w/v) trichloroacetic acid. The contents were centrifuged at 3000 g for 20 min and then 500 ml of supernatant was mixed with 500 ml of water. After, the inorganic phosphate concentration was measured by the method of Fiske and Subbarow (1925). Results were expressed as nmol ATP hydrolyzed/min/mg protein.
Evaluation of mitochondrial membrane potential (MMP)
MMP is monitored with the help of fluorescent cationic dye tetra-methyl rhodamine methyl ester (TMRM). Briefly, 100 μl of mitochondrial suspension was added to 890 μl of assay buffer (80 mM NaCl, 75 mM KCl, 25 mM d-glucose, and 25 mM HEPES, pH 7.4) and then 10 μl of 15 μM of TMRM solution was added to the above mixture and incubated for 15 min at 37 °C. After incubation 400 μl of PBS was added and centrifuged at 10,000 rpm. The pellet was collected in PBS and the rhodamine dye taken up by mitochondria was measured with a spectrofluorometer (Hitachi, F-2500, Japan) (Huang 2002). The fluorescence emission was read at an excitation λ of 535 ± 10 nm and emission λ of 580 ± 10 nm using slit no. 10. The peak fluorescence intensity recorded was around 570 ± 5 nm. The results were expressed as fluorescence intensity value/mg protein.
Measurement of Na+, K + -ATPase activity
Activity of Na+, K + -ATPase was measured in striatum according to Silva et al. (2013). Briefly, the reaction medium consisted of 30 mM Tris–HCl buffer (pH 7.4), 0.1 mM EDTA, 50 mM NaCl, 5 mM KCl, 6 mM MgCl2, and 50 μg protein in the presence or absence of ouabain (1 mM) to 350 μl final volume. The reaction was started by adding adenosine triphosphate (ATP) to 5 mM final concentration. After 30 min at 37 °C, reaction was stopped by adding 70 μl trichloroacetic acid (50%). Appropriate controls were included in the assays for ATP non-enzymatic hydrolysis. The amount of inorganic phosphate released was quantified by the colorimetric method described by Fiske and Subbarow (1925), and the Na+, K + -ATPase activity was calculated by subtracting the ouabain-insensitive activity from the overall activity (in the absence of ouabain).
Estimation of Caspase-3 (casp-3) and Caspase-9 (casp-9) activities
The striatal tissue homogenates were tested for caspase-3 and caspase-9 activities by the addition of a caspase-specific peptide that is conjugated to the colour reporter molecule p-nitroanaline (pNA). The cleavage of the peptide by the caspase releases the chromophore pNA, which was assessed spectrophotometrically at a wavelength of 405 nm. The enzymatic reaction for caspases activities were carried out using caspase3 and caspase-9 colorimetric kit (R&D Systems, Minneapolis, MN, USA) according to the manufacturer’s instructions.
Statistical analysis
The data distribution was verified by applying the Kolmogorov-Smirnov test. The results are presented as the means ± standard error of the mean (SEM). Comparisons between the experimental and control groups were performed using two-way analysis of variance (ANOVA), followed by Bonferroni post hoc tests when appropriate. A value of p < 0.05 was considered significant. All tests were performed using GraphPad Prism software 6.0 (San Diego, CA, USA).
Results
6-OHDA injection and hesperidin treatment did not affect locomotor activity in the OFT
Two-way ANOVA of total distance travelled in the OFT revealed that locomotor activity was not changed significantly by 6-OHDA (F1,24 = 0.00 p = 0.9843), hesperidin (F1,24 = 0.15 p = 0.7036) nor 6-OHDA × hesperidin interaction (F1,24 = 0.58 p = 0.4565), indicating that treatments did not cause sedative or excitatory effects on the animals (Fig.2a).
Effect of hesperidin treatment and stereotaxic surgery injection of 6-OHDA on total distance travelled in the OFT (a), contralateral rotations induced by apomorphine (0.6 mg/kg) (b) and olfactory discrimination in ODT (c). Values are mean ± S.E.M. (n = 6–8 per group). **: p < 0.01, ***: p < 0.001 when compared 6-OHDA/vehicle with sham/vehicle; #: p < 0.05 when compared 6-OHDA/hesperidin with 6-OHDA/vehicle (two-way ANOVA and Bonferroni post hoc test)
Hesperidin attenuated apomorphine-induced cicling behavior in 6-OHDA-lesioned mice
Statistical analysis of number of contralateral rotations induced by apomorphine yielded a significant effect of 6-OHDA (F1,24 = 94.72 p < 0.001) and hesperidin (F1,24 = 35.65 p < 0.001). Post hoc comparisons demonstrated that 6-OHDA/vehicle group dramatically increased contralateral rotations when compared with sham/vehicle group. In contrast, hesperidin treatment attenuated the increase in the number of rotations induced by 6-OHDA, partially reversing motor dysfunction (Fig. 2b).
Hesperidin reversed olfactory dysfunction induced by 6-OHDA injection
Two-way ANOVA of olfactory discrimination in the ODT showed a significant 6-OHDA (F1,16 = 14.04 p < 0.01), hesperidin (F1,20 = 23.59 p < 0.001) and 6-OHDA × hesperidin interaction (F1,20 = 19.10 p < 0.001). Post hoc comparisons demonstrated that 6-OHDA-lesioned mice spent less time in familiar compartment when compared with control group, inducing an impairment in olfactory ability. Oral treatment with hesperidin fully protected against the decrease of olfactory function caused by 6-OHDA injection (Fig. 2c).
6-OHDA-induced spatial memory deficit in the Barnes maze is prevented by hesperidin
Two-way ANOVA of spatial memory in the BM at day 5 revealed a significant 6-OHDA × hesperidin interaction in time to reach target (F1,24 = 12.77 p < 0.01) and number of errors (F1,24 = 5.81 p < 0.05). Post hoc comparisons found that 6-OHDA-lesioned mice took significantly longer than sham/vehicle to reach target and had an increase in the number of erros in the 5th day, indicating a deficit in spatial memory. On the other hand, hesperidin protected against the spatial learning deficits induced by 6-OHDA injection, preserving the cognitive performance at the levels of control group (Fig. 3a-b).
Effect of hesperidin treatment and intrastriatal injection of 6-OHDA on time to target virtual scape hole and number of errors at 5th day (a and b) and 12th day (c and d) in the Barnes Maze test. Values are mean ± S.E.M. (n = 8 per group). **: p < 0.01, ***: p < 0.001 when compared 6-OHDA/vehicle with sham/vehicle; #: p < 0.05 when compared 6-OHDA/hesperidin with 6-OHDA/vehicle (two-way ANOVA and Bonferroni post hoc test)
In the BM at day 12, statistical analysis of time to reach target and number of erros yielded a significant 6-OHDA × hesperidin interaction (F1,24 = 37.63 p < 0.001 and F1,24 = 6.59 p < 0.05, respectively). Once again, hesperidin treatment protected against spatial memory impairment induced by 6-OHDA, as shown by the decrease of time to reach target and normalization of number of erros (Fig. 3c-d).
In the acquisition phase (four trials per day for four consecutive days), no significant differences were found between groups for both time to target and number of errors (data not shown).
Hesperidin attenuates the loss of dopaminergic neurons in the substantia nigra pars compacta (SNpc) of 6-OHDA-lesioned mice
Dopaminergic cell death in the SNpc was measured 28 days after 6-OHDA microinjection through the estimation of the total number of TH+ neurons using stereological method (Fig. 4). This method is designed to describe quantitative analysis of the mammalian brain and provide the estimation of the total number of cells from thick sections samples using 3-D optical sectioning (Gundersen et al. 1988; Gundersen 2002).
Effect of hesperidin treatment and intrastriatal injection of 6-OHDA on tyrosine hydroxylase (TH)-positive cells in the susbtantia nigra pars compact (SNpc) evaluated through immunohistochemistry in different scale bar: 5x, 20x and 63x (a). The figure (b) shows the effect of hesperidin and 6-OHDA microinjection on the total number estimated of TH+ cells in the SNpc. Values are mean ± S.E.M. (n = 6 per group). ***: p < 0.001 when compared 6-OHDA/vehicle with sham/vehicle; #: p < 0.05 when compared 6-OHDA/hesperidin with 6-OHDA/vehicle (two-way ANOVA and Bonferroni post hoc test)
Two-way ANOVA of TH+ cells revealed a significant 6-OHDA (F1,20 = 49.38 p < 0.001), hesperidin (F1,20 = 4.80 p < 0.05) and 6-OHDA × hesperidin interaction (F1,20 = 8.63 p < 0.01). Post hoc comparisons showed that 6-OHDA significantly decreased TH+ cells in SNpc of mice when compared with control group. Hesperidin treatment partially restored the decrease of TH+ cells caused by 6-OHDA. Although TH+ immunoreactivity was decreased in sham hesperidin mice, stereological cell counts of SNpc TH+ neurons were not significantly altered by hesperidin compared to sham control (Fig. 4a-b).
Effect of 6-OHDA and hesperidin on DA, DOPAC and HVA levels
Two-way ANOVA of DA levels in the striatum demonstrated a main effect of 6-OHDA (F1,20 = 40.58 p < 0.001) and 6-OHDA × hesperidin interaction (F1,20 = 12.31 p < 0.01). In addition, a significant 6-OHDA × hesperidin interaction was found to the levels of DOPAC (F1,20 = 5.56 p < 0.05) and HVA (F1,20 = 6.10 p < 0.05).
Post hoc comparisons demonstrated that marked decrease of DA, DOPAC and HVA levels in the striatum was mitigated by hesperidin treatment (Fig. 5a-c).
Effect of hesperidin treatment and stereotaxic surgery injection of 6-OHDA on the levels of DA (a), DOPAC (b) and HVA (c) in the striatum. Values are mean ± S.E.M. (n = 6 per group). **: p < 0.01, *** p < 0.001 when compared 6-OHDA/vehicle with sham/vehicle; #: p < 0.05 when compared 6-OHDA/hesperidin with 6-OHDA/vehicle (two-way ANOVA and Bonferroni post hoc test)
Hesperidin protected against mitochondrial dysfunction caused by 6-OHDA injection
The activity of the mitochondrial respiratory chain complex-I, II, IV and V was analyzed in order to evaluate mitochondrial function in the striatum of mice.
Statistical analysis of mitochondrial complex-I activity in the striatum revealed a significant effect of 6-OHDA (F1,20 = 60.28 p < 0.001) and 6-OHDA × hesperidin interaction (F1,20 = 0.835 p < 0.05). Post hoc comparisons showed that hesperidin treatment protected against the inhibition of complex I induced by 6-OHDA. Moreover, complex-I activity in sham/hesperidin mice were significantly increased when compared to control counterparts, indicating a per se effect of hesperidin (Fig. 6a).
Effect of hesperidin treatment and intrastriatal injection of 6-OHDA on the activity of complex I (a), complex II (b), complex IV (c) and complex V (d) of the mitochondrial respiratory chain in the striatum of mice. Values are mean ± S.E.M. (n = 6 per group). *: p < 0.05, *** p < 0.001 when compared 6-OHDA/vehicle with sham/vehicle; #: p < 0.05 when compared 6-OHDA/hesperidin with 6-OHDA/vehicle; @: p < 0.05 when compared hesperidin/vehicle with sham/vehicle (two-way ANOVA and Bonferroni post hoc test)
Two-way ANOVA of mitochondrial complex-II activity in the striatum yielded a main effect of 6-OHDA (F1,20 = 7.82 p < 0.05). Post hoc comparisons demonstrated that 6-OHDA caused an inhibition of complex II-activity when compared with the sham/vehicle mice, without a significant protection of hesperidin treatment (Fig. 6b).
Statistical analysis of complex-IV and complex-V in the striatum revealed a significant 6-OHDA × hesperidin interaction (F1,20 = 5.60 p < 0.05 and F1,20 = 9.61 p < 0.01 respectively). Post hoc comparisons demonstrated that 6-OHDA-lesioned mice showed decreased complex-IV and complex-V activities when compared with the control group. In contrast, hesperidin treatment mitigates the inhibition of complex-IV and abrogated the reduction in complex-V, acting as a protective compound against 6-OHDA-mediated mitochondrial dysfunction (Fig. 6c-d).
Effect of 6-OHDA and hesperidin on mitochondrial membrane potential (MMP) and Na+, K + -ATPase activity
The MMP was assessed in the striatum of mice in order to analyse mitochondrial integrity. Two-way ANOVA of MMP in the striatum yielded a significant effect of 6-OHDA (F1,20 = 14.73 p < 0.01) and 6-OHDA × hesperidin interaction (F1,20 = 13.37 p < 0.01). Post hoc comparisons revealed that 6-OHDA caused a marked decrease in the function of mitochondrial integrity, as shown by reduced MMP when compared to control group. In contrast, hesperidin treatment abrogated the disruption of mitochondrial integrity elicited by 6-OHDA (Fig. 7a).
Effect of hesperidin treatment and intrastriatal injection of 6-OHDA on the mitochondrial membrane potential (a) and Na+, K+-ATPase activity in the striatum of mice (n = 6 per group). *: p < 0.05, ***p < 0.001 when compared 6-OHDA/vehicle with sham/vehicle; #: p < 0.05 when compared 6-OHDA/hesperidin with 6-OHDA/vehicle; (two-way ANOVA and Bonferroni post hoc test)
Statistical analysis of Na+, K + -ATPase activity in the striatum showed a significant effect of 6-OHDA (F1,20 = 13.38 p < 0.01) and hesperidin (F1,20 = 4.90 p < 0.05). Post hoc comparisons demonstrated that 6-OHDA injection significantly decreased Na+, K + -ATPase activity in the striatum of mice. Hesperidin attenuated the reduction of Na+, K + -ATPase activity evoked by 6-OHDA (Fig. 7b).
Hesperidin treatment mitigated the increase in apoptosis markers evoked by 6-OHDA
The activities of caspase-3 and caspase-9 were assessed as marker for apoptosis in the striatum. Two-way ANOVA of casp-3 and casp-9 activity in the striatum revealed a significant 6-OHDA × hesperidin interaction (casp-3: F1,20 = 14.84 p < 0.01; casp-9: F1,20 = 19.19 p < 0.001). Post hoc comparisons showed that 6-OHDA caused an increase of Casp-3 and -9 activities when compared to control counterparts. Hesperidin treatment protected against the increase in caspase activities mediated by 6-OHDA, suggesting a hesperidin-induced antiapoptotic effect (Fig. 8a-b).
Effect of hesperidin treatment and intrastriatal injection of 6-OHDA on the activity of caspase-3 (a) and caspase-9 (b) (n = 6 per group). ***p < 0.001 when compared 6-OHDA/vehicle with sham/vehicle; #: p < 0.05 when compared 6-OHDA/hesperidin with 6-OHDA/vehicle; (two-way ANOVA and Bonferroni post hoc test)
Discussion
PD is a devastating neurodegenerative disease whose knowledge about the mechanisms involved in neurodegeneration and cell death remains not fully understood. Current medications act just on a single target and alleviate symptoms via enhancing DA levels directly or indirectly. Thus, the discovery of compounds that act preventing or reversing the dopaminergic neurodegenerative process remains a big challenge (Kim et al. 2012; Xie et al. 2014).
Naturally occurring flavonoids which are widely present in vegetables, fruits and seeds, are potential candidates for the therapy of neurodegenerative diseases (Solanki et al. 2016). In the present study, we found that oral treatment with flavonoid hesperidin for 28 days promoted neuroprotective effects against motor, cognitive and olfactive dysfunctions induced by 6-OHDA. These results are accompanied by a restorative effect on DA neurons and DA levels by the attenuation of mitochondrial dysfunction and markers of apoptosis in the striatum of mice
Hesperidin improves behavioral alterations and attenuates 6-OHDA-induced damage to dopaminergic neurons
The intrastriatal injection of 6-OHDA is a valid experimental model for studying PD in rodents. Several neurochemical alterations caused by 6-OHDA neurotoxicity are similar to those observed in PD patients (Hernandez-Baltazar et al. 2017). Among these deleterious effects, the reduction in DA levels and dopaminergic cell death was observed (Vianello et al. 2016). The function of dopaminergic neurons can be evaluated by determining the levels of DA, DOPAC, HVA. Moreover, the amount of dopaminergic neurons in SNpc can be measured by TH+ cells content, the rate-limiting enzyme of DA synthesis (Kozina et al. 2014; Soto-Otero et al. 2000). In the current study, we found that 6-OHDA injection caused a disruption in dopaminergic dynamics, as reflected by a marked decrease in TH+ content in the SNpc and a depletion of DA, DOPAC and HVA levels in the striatum, reinforcing our previous studies (Del Fabbro et al. 2019; Goes et al. 2018). In addition, these DA system changes are linked with prominent motor asymmetry, as obserded by the increase in contralateral rotations in 6-OHDA-lesioned mice.
On the other hand, hesperidin treatment protected against 6-OHDA-induced DA impairments, thereby mitigating the decrease of TH+ cells in the SNpc and the reduction of DA, DOPAC and HVA levels in the striatum. Hesperidin also ameliorated the increased circling behavior caused by 6-OHDA, likely due to its ability to preserve nigrostriatal dopaminergic neurons and DA levels in the striatum. Thus, we extend our previous observations indicating that hesperidin is able to prevent the loss of dopaminergic neurons in the SNpc, then contributing to the improvement of motor dysfunction elicited by 6-OHDA neurotoxicity.
It is noteworthy to emphasize that, although TH+ imunoreactivity in the SNpc was decreased in the hesperidin group as compared to control, TH-positive cells were not affected by hesperidin treatment. In the present study, we used stereological method with optical fractionator design for estimating the total number of TH+ neurons in the SNpc. In the technique used in our study, all cells that presented the minimum marking to the developer, regardless of their intensity, were considered positive. Thus, qualitative observation regarding the decrease in intensity does not interfere with the quantification method used for stereological analysis. The biological repercussion of this quantification is that treatment with hesperidin was able to mitigate the loss of TH+ neurons in the SNpc caused by 6-OHDA and did not cause DA neuron impairment in control animals (sham hesperidin).
Olfactory dysfunction is a common non-motor symptom among PD patients and about 75%–90% of patients have hyposmia (Nishi et al. 1999; Ross et al. 2008). In our study, we observed that 6-OHDA-lesioned mice exhibited olfactory dysfunction, as reflected by the reduction of time spent in the familiar compartment in ODT, when compared to control counterparts. Corroborating our findings, it has reported that 6-OHDA contributes to clinical symptoms of PD by depleting striatal DA content (Kumar et al. 2012) and dopaminergic denervation was directly related to olfactory dysfunction after 6-OHDA injection (Valle-Leija and Drucker-Colín 2014). Here, 6-OHDA/hesperidin mice spent more time in familiar compartment, indicating a hesperidin-induced protection of olfactory function.
The present study also demonstrated that 6-OHDA caused a marked impairment of spatial memory of mice. For instance, 6-OHDA-lesioned mice showed a reduction in the time to target and increased number of errors in 5th day (short-term retention) and 12th day (long-term retention) in the Barnes maze. By contrast, hesperidin treatment blocked short and long-term spatial memory deficits induced by 6-OHDA injection. These results are in line with a previou study of our laboratory, in which we demonstrated that hesperidin protected against 6-OHDA-mediated memory impairment in Morris water maze test, a finding that are related to the ability of hesperidin in increasing striatal DA levels (Antunes et al. 2014).
Altogether, our results suggest that restorative effect on DA levels and dopaminergic neurons may be important neurobiological mechanisms of hesperidin against behavioral disturbances induced by 6-OHDA injection.
Hesperidin mitigates mitochondrial dysfunction and increased apoptosis markers induced by 6-OHDA injection
Altough the exact pathophysiological mechanisms of PD remain unknown, the role of mitochondrial dysfunction is recognized (Bose and Beal 2016; Rocha et al. 2018). The present study showed that 6-OHDA injection reduced the activity of mitochondrial respiratory chain enzymes, including complex I, II, IV and V in the striatum, causing a mitochondrial dysfunction. Corroborating our results, it has been shown that the respiratory control ratio, the main parameter of mitochondrial function, was impaired by 6-OHDA administration (Kupsch et al. 2014). It has been reported that mitochondrial damage is closely related to degenerative processes of dopaminergic neurons in the SNpc (Rocha et al. 2018). Moreover, impaired mitochondrial function is also associated with an increase in reactive oxygen species generation, which ultimately leads to DA neuron death (Lin and Beal 2006).
In our study, the treatment with hesperidin protected against the inhibition of mitochondrial complex I, IV and V in the striatum, suggesting that this flavonoid may play a role in preserving mitochondrial function. It is an important finding, indicating that hesperidin, due to its antioxidant mechanisms (Antunes et al. 2014) and by preventing mitochondrial defects, could act in different process that contribute to striatal damage and loss of DA neurons in 6-OHDA model of PD. Another important finding of the present study is that 6-OHDA injection decreased MMP in the striatum, indicating a loss of mitochondrial integrity and disrupting effect of cellular bioenergetics. Conversely, hesperidin treatment attenuated the 6-OHDA-induced decrease in MMP in the striatum, showing a stabilizing effect on physiological function of the respiratory chain to generate ATP.
The enzyme Na + K + -ATPase plays a critical role in cell function, regulating the exchange of Na + and K+ between the cell and the intercellular space. The loss of function of this enzyme can activate neuronal apoptosis by altering K+ homeostasis (Wang et al. 2003). In the present study, Na + K+ ATPase activitiy was significantly decreased in the striatum of 6-OHDA-lesioned mice. The decrease in this membrane ATPase could be due to the mitochondrial defects induced by 6-OHDA, contributing to neuronal damage and death. The hesperidin treatment mitigated the inhibition of Na + K+ ATPase evoked by 6-OHDA, acting as a membrane stabilizer compound in the neurons of striatum.
We also found that 6-OHDA-neurotoxicity is associated with an activation of apoptotic patways, as revealed by the increase of caspase-3 and caspase-9 activities in the striatum. In addition to its crucial role in cellular energy metabolism, mitochondria have long been recognized to modulate apoptosis by activation of caspase cascades (Hauser and Hastings 2013). In agreement with our results, it has been demonstrated that 6-OHDA-induced apoptosis is mediated by mitochondrial dysfunction in mice (Zhang et al. 2016). Indeed, the release of cytochrome C after mitochondrial damage is involved in caspase-3 activation (Li et al. 1997). In the present study, hesperidin treatment blocked the exacerbated activation of caspase-3 and caspase-9 in the striatum, exhibiting an anti-apoptotic effect in a neurotoxin experimental model of PD. Therefore, we demonstrated that hesperin modulates the dopaminergic neurons in SNpc through mechanisms involved in apoptotic cell death. These results represent another relevant therapeutic strategy for PD using hesperidin administration.
The present findings together support and extend the potential of oral treatment with hesperidin for 4 weeks as a therapeutic approach to alleviate behavioral abnormalities and neurodegeneration induced by instrastriatal injection of 6-OHDA. This study highlighs novel evidences about the neuroprotective mechanisms of hesperidin. A synopsis of our major findings can be seen in Fig. 9.
Synoptic diagram showing the effect of oral treatment with hesperidin in 6-OHDA-treated mice. In this study, intrastriatal injection of 6-OHDA caused mitochondrial dysfunction, reflected by the inhibition of mitochondrial enzymatic complex-I, II, III and IV, which resulted in increased caspase-3 and -9 activities in the striatum. These 6-OHDA-neurotoxic mechanisms culminated in reduced striatal DA, DOPAC and HVA levels and loss of TH+ cells in the SNpc, which contributes to the development of motor, cognitive and olfactory function abnormalities. The oral treatment of hesperidin abrogated neurochemical deficits and ameliorated behavioral disturbances induced by 6-OHDA
Conclusion
In conclusion, our results show that hesperidin exerts neuroprotection against 6-OHDA-induced impairment of dopaminergic neurons in the SNpc. We indicate that this protection was related to attenuation of mitochondrial dysfunction and mitochondrial-mediated apoptotic pathways. The findings of the present study represent a relevant therapeutic strategy to counteract motor, cognitive and olfactive impairments in a relevant mouse model of PD. Further studies on human subjects are needed to explore the potential of treatment with hesperidin as a part of neuroprotective strategies for PD patients.
References
Abushouk AI, Negida A, Ahmed H, Abdel-Daim MM (2017) Neuroprotective mechanisms of plant extracts against MPTP induced neurotoxicity: future applications in Parkinson’s disease. Biomed Pharmacother 85:635–645. https://doi.org/10.1016/j.biopha.2016.11.074
Antunes MS, Goes ATR, Boeira SP, Prigol M, Jesse CR (2014) Protective effect of hesperidin in a model of Parkinson’s disease induced by 6-hydroxydopamine in aged mice. Nutrition 30:1415–1422. https://doi.org/10.1016/j.nut.2014.03.024
Antunes MS, Jesse CR, Ruff JR, de Oliveira Espinosa D, Gomes NS, Altvater EET, Donato F, Giacomeli R, Boeira SP (2016) Hesperidin reverses cognitive and depressive disturbances induced by olfactory bulbectomy in mice by modulating hippocampal neurotrophins and cytokine levels and acetylcholinesterase activity. Eur J Pharmacol 789:411–420. https://doi.org/10.1016/j.ejphar.2016.07.042
Balestrino R, Martinez-Martin P (2017) Neuropsychiatric symptoms, behavioural disorders, and quality of life in Parkinson’s disease. J Neurol Sci 373:173–178. https://doi.org/10.1016/j.jns.2016.12.060
Bose A, Beal MF (2016) Mitochondrial dysfunction in Parkinson’s disease. J Neurochem 139:216–231. https://doi.org/10.1111/jnc.13731
Carabelas R (2016) Parkinson’s disease: old concepts and new challenges. Alzheimers Dis Dement 1. https://doi.org/10.36959/734/36
Chaudhuri KR, Schapira AH (2009) Non-motor symptoms of Parkinson’s disease: dopaminergic pathophysiology and treatment. Lancet Neurol 8:464–474. https://doi.org/10.1016/S1474-4422(09)70068-7
David Adams Jr J (2012) Parkinson’s Disease-Apoptosis and Dopamine Oxidation Open J Apoptosis 1:1–8. https://doi.org/10.4236/ojapo.2012.11.001
Del Fabbro L et al (2019) Chrysin protects against behavioral, cognitive and neurochemical alterations in a 6-hydroxydopamine model of Parkinson’s disease. Neurosci Lett 706:158–163. https://doi.org/10.1016/j.neulet.2019.05.036
Fiske CH, Subbarow Y (1925) The colorimetric determination of phosphorus. J Biol Chem 66:375–400
Garg A, Garg S, Zaneveld LJD, Singla AK (2001) Chemistry and pharmacology of the Citrus bioflavonoid hesperidin. Phyther Res 15:655–669. https://doi.org/10.1002/ptr.1074
Gaur V, Kumar A (2010) Hesperidin pre-treatment attenuates NO-mediated cerebral ischemic reperfusion injury and memory dysfunction. Pharmacol Reports 62:635–648. https://doi.org/10.1016/S1734-1140(10)70321-2
Goes ATR, Souza LC, Filho CB, del Fabbro L, de Gomes MG, Boeira SP, Jesse CR (2014) Neuroprotective effects of swimming training in a mouse model of Parkinson’s disease induced by 6-hydroxydopamine. Neuroscience 256:61–71. https://doi.org/10.1016/j.neuroscience.2013.09.042
Goes ATR, Jesse CR, Antunes MS, Lobo Ladd FV, Lobo Ladd AAB, Luchese C, Paroul N, Boeira SP (2018) Protective role of chrysin on 6-hydroxydopamine-induced neurodegeneration a mouse model of Parkinson’s disease: involvement of neuroinflammation and neurotrophins. Chem Biol Interact 279:111–120. https://doi.org/10.1016/j.cbi.2017.10.019
Griffiths DE, Houghton RL (1974) Studies on energy-linked reactions: modified mitochondrial ATPase of Oligomycin-resistant mutants of Saccharomyces cerevisiae. Eur J Biochem 46:157–167. https://doi.org/10.1111/j.1432-1033.1974.tb03608.x
Gundersen HJG (2002) The smooth fractionator. J Microsc 207:191–210. https://doi.org/10.1046/j.1365-2818.2002.01054.x
Gundersen HJG, Bagger P, Bendtsen TF, Evans SM, Korbo L, Marcussen N, MØLler A, Nielsen K, Nyengaard JR, Pakkenberg B, SØRensen FB, Vesterby A, West MJ (1988) The new stereological tools: Disector, fractionator, nucleator and point sampled intercepts and their use in pathological research and diagnosis. Apmis 96:857–881. https://doi.org/10.1111/j.1699-0463.1988.tb00954.x
Hauser DN, Hastings TG (2013) Mitochondrial dysfunction and oxidative stress in Parkinson’s disease and monogenic parkinsonism. Neurobiol Dis 51:35–42. https://doi.org/10.1016/j.nbd.2012.10.011
Hernandez-Baltazar D, Zavala-Flores LM, Villanueva-Olivo A (2017) The 6-hydroxydopamine model and parkinsonian pathophysiology: novel findings in an older model. Neurol 32:533–539. https://doi.org/10.1016/j.nrleng.2015.06.019
Huang SG (2002) Development of a high throughput screening assay for mitochondrial membrane potential in living cells. J Biomol Screen 7:383–389. https://doi.org/10.1177/108705710200700411
Kalia LV, Lang AE (2015) Parkinson’s disease. Lancet 386:896–912. https://doi.org/10.1016/S0140-6736(14)61393-3
Kim SR, Kareva T, Yarygina O, Kholodilov N, Burke RE (2012) AAV transduction of dopamine neurons with constitutively active rheb protects from neurodegeneration and mediates axon regrowth. Mol Ther 20:275–286. https://doi.org/10.1038/mt.2011.213
Kozina EA, Khakimova GR, Khaindrava VG, Kucheryanu VG, Vorobyeva NE, Krasnov AN, Georgieva SG, Kerkerian-le Goff L, Ugrumov MV (2014) Tyrosine hydroxylase expression and activity in nigrostriatal dopaminergic neurons of MPTP-treated mice at the presymptomatic and symptomatic stages of parkinsonism. J Neurol Sci 340:198–207. https://doi.org/10.1016/j.jns.2014.03.028
Kumar A, Sharma N, Gupta A, Kalonia H, Mishra J (2012) Neuroprotective potential of atorvastatin and simvastatin (HMG-CoA reductase inhibitors) against 6-hydroxydopamine (6-OHDA) induced Parkinson-like symptoms. Brain Res 1471:13–22. https://doi.org/10.1016/j.brainres.2012.06.050
Kupsch A, Schmidt W, Gizatullina Z, Debska-Vielhaber G, Voges J, Striggow F, Panther P, Schwegler H, Heinze HJ, Vielhaber S, Gellerich FN (2014) 6-Hydroxydopamine impairs mitochondrial function in the rat model of Parkinson’s disease: Respirometric, histological, and behavioral analyses. J Neural Transm 121:1245–1257. https://doi.org/10.1007/s00702-014-1185-3
Li P, Nijhawan D, Budihardjo I, Srinivasula SM, Ahmad M, Alnemri ES, Wang X (1997) Cytochrome c and dATP-dependent formation of Apaf-1/caspase-9 complex initiates an apoptotic protease cascade. Cell 91:479–489. https://doi.org/10.1016/s0092-8674(00)80434-1
Lin MT, Beal MF (2006) Mitochondrial dysfunction and oxidative stress in neurodegenerative diseases. Nature 443:787–795. https://doi.org/10.1038/nature05292
Lowry OH, Rosenborough NJ, Farr AL, Randall RJ (1951) Protein measurement with folin phenol reagente. J Biol Chem 193:265–275.
Nishi A, Fisone G, Snyder GL, Dulubova I, Aperia A, Nairn AC, Greengard P (1999) Regulation of Na+, K+-ATPase isoforms in rat neostriatum by dopamine and protein kinase C. J Neurochem 73:1492–1501
Oorschot DE (1996) Total number of neurons in the neostriatal, pallidal, subthalamic, and substantia nigral nuclei of the rat basal ganglia: a stereological study using the cavalieri and optical disector methods. J Comp Neurol 366:580–599
Paxinos G, Franklin, KBJ (2001). The mouse brain in stereotaxic coordinates: second edition (deluxe) by academic press, New York, ISBN 0-12-547637-X
Pedersen PL, Greenawalt JW, Reynafarje B, Hullihen J, Decker GL, Soper JW, Bustamente E (1978) Preparation and characterization of mitochondria and submitochondrial particles of rat liver and liver-derived tissues. Methods Cell Biol 20:411–481. https://doi.org/10.1016/s0091-679x(08)62030-0
Perier C, Bové J, Wu DC, Dehay B, Choi DK, Jackson-Lewis V, Rathke-Hartlieb S, Bouillet P, Strasser A, Schulz JB, Przedborski S, Vila M (2007) Two molecular pathways initiate mitochondria-dependent dopaminergic neurodegeneration in experimental Parkinson’s disease. Proc Natl Acad Sci U S A 104:8161–8166. https://doi.org/10.1073/pnas.0609874104
Prediger RDS, Aguiar AS Jr, Rojas-Mayorquin AE, Figueiredo CP, Matheus FC, Ginestet L, Chevarin C, Bel ED, Mongeau R, Hamon M, Lanfumey L, Raisman-Vozari R (2010) Single intranasal administration of 1-methyl-4-phenyl-1,2,3,6- tetrahydropyridine in C57BL/6 mice models early preclinical phase of Parkinson’s disease. Neurotox Res 17:114–129. https://doi.org/10.1007/s12640-009-9087-0
Prut L, Belzung C (2003) The open field as a paradigm to measure the effects of drugs on anxiety-like behaviors: a review. Eur J Pharmacol 463:3–33. https://doi.org/10.1016/S0014-2999(03)01272-X
Rocha EM, De Miranda B, Sanders LH (2018) Alpha-synuclein: pathology, mitochondrial dysfunction and neuroinflammation in Parkinson’s disease. Neurobiol Dis 109:249–257. https://doi.org/10.1016/j.nbd.2017.04.004
Ross GW, Petrovitch H, Abbott RD, Tanner CM, Popper J, Masaki K, Launer L, White LR (2008) Association of olfactory dysfunction with risk for future Parkinson’s disease. Ann Neurol 63:167–173. https://doi.org/10.1002/ana.21291
Schmitz C, Hof PR (2005) Design-based stereology in neuroscience. Neuroscience 130:813–831. https://doi.org/10.1016/j.neuroscience.2004.08.050
Shapiro BL, Feigal RJ, Lam LFH (1979) Mitochondrial NADH dehydrogenase in cystic fibrosis. Proc Natl Acad Sci U S A 76:2979–2983. https://doi.org/10.1073/pnas.76.6.2979
Silva LFA, Hoffmann MS, Gerbatin RR, Fiorin FS, Dobrachinski F, Mota BC, Wouters ATB, Pavarini SP, Soares FAA, Fighera MR, Royes LFF (2013) Treadmill exercise protects against pentylenetetrazol-induced seizures and oxidative stress after traumatic brain injury. J Neurotrauma 30:1278–1287. https://doi.org/10.1089/neu.2012.2577
Solanki I, Parihar P, Parihar MS (2016) Neurodegenerative diseases: From available treatments to prospective herbal therapy. Neurochem Int 95:100–108. https://doi.org/10.1016/j.neuint.2015.11.001
Soto-Otero R, Méndez-Álvarez E, Hermida-Ameijeiras Á, Muñoz-Patiño AM, Labandeira-Garcia JL (2000) Autoxidation and neurotoxicity of 6-hydroxydopamine in the presence of some antioxidants: potential implication in relation to the pathogenesis of Parkinson’s disease. J Neurochem 74:1605–1612. https://doi.org/10.1046/j.1471-4159.2000.0741605.x
Storrie B, Madden EA (1990) Isolation of subcellular organelles. Methods Enzymol 182:203–225. https://doi.org/10.1016/0076-6879(90)82018-W
Ungerstedt U (1971) Postsynaptic Supersensitivity after 6-Hydroxy-dopamine induced degeneration of the Nigrostriatal dopamine system. Acta Physiol Scand 82:69–93. https://doi.org/10.1111/j.1365-201X.1971.tb11000.x
Valle-Leija P, Drucker-Colín R (2014) Unilateral olfactory deficit in a hemiparkinson’s disease mouse model. Neuroreport 25:948–953. https://doi.org/10.1097/WNR.0000000000000218
Vauzour D, Vafeiadou K, Rodriguez-Mateos A, Rendeiro C, Spencer JPE (2008) The neuroprotective potential of flavonoids: a multiplicity of effects. Genes Nutr 3:115–126. https://doi.org/10.1007/s12263-008-0091-4
Vianello R, Domene C, Mavri J (2016) The use of multiscale molecular simulations in understanding a relationship between the structure and function of biological systems of the brain: the application to monoamine oxidase enzymes. Front Neurosci 10:327. https://doi.org/10.3389/fnins.2016.00327
Walker JM, Fowler SW, Miller DK, Sun AY, Weisman GA, Wood WG, Sun GY, Simonyi A, Schachtman TR (2011) Spatial learning and memory impairment and increased locomotion in a transgenic amyloid precursor protein mouse model of Alzheimer’s disease. Behav Brain Res 222:169–175. https://doi.org/10.1016/j.bbr.2011.03.049
Wang XQ et al (2003) Apoptotic insults impair Na+, K+-ATPase activity as a mechanism of neuronal death mediated by concurrent ATP deficiency and oxidant stress. J Cell Sci 116:2099–2110. https://doi.org/10.1242/jcs.00420
Xie A, Gao J, Xu L, Meng D (2014) Shared mechanisms of neurodegeneration in alzheimer’s disease and parkinson’s disease. Biomed Res Int 2014:648740–648748. https://doi.org/10.1155/2014/648740
Yang C, Garrett-Mayer E, Schneider JS, Gollomp SM, Tilley BC (2009) Repeatable battery for assessment of neuropsychological status in early Parkinson’s disease. Mov Disord 24:1453–1460. https://doi.org/10.1002/mds.22552
Zhang Z, Hou L, Li X, Ju C, Zhang J, Li X, Wang X, Liu C, Lv Y, Wang Y (2016) Neuroprotection of inositol hexaphosphate and changes of mitochondrion mediated apoptotic pathway and α-synuclein aggregation in 6-OHDA induced Parkinson’s disease cell model. Brain Res 1633:87–95. https://doi.org/10.1016/j.brainres.2015.12.035
Acknowledgments
The authors are grateful for financial support by FAPERGS (#16/2551-0000526-5 (PRONUPEQ) and CNPq (#430841/2016-7 (Universal)) Research Grants. LCS is recipient by CNPq fellowship [150560/2019–2]. We would like to thank professor Cristiano Ricardo Jesse for the study design, interpretation of the data and for provided their thoughts and his contribution to the development of the experiments.
Author information
Authors and Affiliations
Corresponding author
Ethics declarations
Conflict of interest
The authors have no conflicts of interest to report.
Additional information
Publisher’s note
Springer Nature remains neutral with regard to jurisdictional claims in published maps and institutional affiliations.
Rights and permissions
About this article
Cite this article
Antunes, M.S., Ladd, F.V.L., Ladd, A.A.B.L. et al. Hesperidin protects against behavioral alterations and loss of dopaminergic neurons in 6-OHDA-lesioned mice: the role of mitochondrial dysfunction and apoptosis. Metab Brain Dis 36, 153–167 (2021). https://doi.org/10.1007/s11011-020-00618-y
Received:
Accepted:
Published:
Issue Date:
DOI: https://doi.org/10.1007/s11011-020-00618-y