Abstract
Chia (Salvia hispanica L.) seed oil is the richest natural source of α-linolenic acid, an n − 3 polyunsaturated fatty acid (ω-3 PUFA), contributing to its use as functional and nutraceutical food in large part of Latin America. However, a food with such fatty acid composition could be highly susceptible to lipid oxidation. Thus, the present study was conducted to determine the thermal and oxidative stability of chia oil by various methods. Rancimat method was used to evaluate the effect of synthetic and natural antioxidants in the oxidative stability. Pressurized differential scanning calorimetry (PDSC), Schaal test and 1H NMR spectroscopy were used to assess the thermal stability. The effect of frying temperature and/or heating time on fatty acid composition was assessed by 1H NMR. The results show that tert-butylhydroquinone (TBHQ) and a mixture of TBHQ and rosemary extract were effective in increasing the oxidative stability of chia oil. Concerning the storage conditions, PDSC, Schaal test and 1H NMR data showed that chia oil is stable at 60 °C; hence, there is no need for special storage conditions. PDSC and 1H NMR results indicate that chia oil cannot be used in cooking and frying, because at high temperatures severe degradation of the unsaturated groups and loss of the nutritional properties of the oil occur.
Similar content being viewed by others
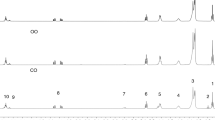
Explore related subjects
Discover the latest articles, news and stories from top researchers in related subjects.Avoid common mistakes on your manuscript.
Introduction
Lipids are essential foods in a healthy diet. They perform numerous biological functions so that a diet low in fat can lead to malfunction of the organism. Vegetable oils, for example, are sources of vitamins, natural antioxidants and essential fatty acids without which the organism would be predisposed to autoimmune diseases, inflammatory diseases, cardiovascular diseases, hypertension, depression, and neurological disorders [1–6].
A large number of studies have shown a relationship between the incidence of cardiovascular diseases and high consumption of saturated fatty acids. Reverse effect occurs with regular intake of foods rich in polyunsaturated acids (PUFAS). Some essential fatty acids are not synthesized by the human body and thus must be included in our regular diet. The linoleic (LA, C18:2, n − 6) and the α-linolenic (LNA, C18:3, n − 3) acids play key roles in human metabolism processes such as inflammation. The n − 6 deficiency, for example, can hinder children growth and lead to accumulation of fat in the liver. A poor diet n − 3, on the other hand, may compromise the development of the brain and the retina and can cause cardiac arrhythmias in patients with ischemic heart disease. Although these acids seem to have different functions, metabolic studies indicate that there is some competition between linoleic and α-linolenic; As a consequence, a proper balance of linoleic and α-linolenic acids should be established regarding their regular intake. Some reports suggest an ideal diet should contain a ratio of α-linolenic to linoleic of 5:1 [7–9].
Chia (Salvia hispanica L.) seed oil is a functional food in further evidence at the moment. Chia is native from the region that extends from west central Mexico to northern Guatemala. It is cultivated mainly in Mexico, Bolivia, Ecuador, Guatemala and Argentina. In Brazil, the commercial production of Chia is not economically important. Although chia has been a plant used by Aztecan tribes, its oil is relatively new in the market with few reports about it. The great appeal of this oil is its high content of LNA between 64.8 and 56.9% and its good n − 6/n − 3 ratio. However, such composition makes the oil highly susceptible to oxidative degradation with loss of nutritional properties and formation of toxic compounds, making it an unsuitable food for human consumption [10–13].
Oxidative stability is an important quality indicator of edible vegetal oils because it gives estimation of shelf-life and of resistance to structural changes caused by intrinsic and extrinsic factors [14]. Different methods are used in determining oil oxidative stability. It can be determined according to the oxygen absorption by the sample and through loss of initial substrates and the formation of products from the decomposition of fatty acids [15]. It can be used for oxidative determination: real-time test that involves high costs of reagents and a large number of analyses; and accelerated tests, which use accelerated aging conditions for the samples that cause oxidation as elevated temperature, increased oxygen pressure, addition of metals and light exposure [16].
Rancimat is a standard method used in the determination of the stability of fats and oils [17]. It measures the change in electrical conductivity of deionized water caused by the presence of volatile products from the secondary oxidation of lipids. An electric conductivity curve (μs cm−1) versus time (h) is generated automatically by the equipment software. The straight projections passing through the baseline and the tangent from the inflection point of the curve intersect at a point in time that corresponds to the oxidative induction period (IP). The method is advantageous in industry because no pretreatment of the sample is required and very less reagents are necessary. However, the method is not reproducible. The rancimat glass tube cleaning is critical [18] and it is not adequate for the analysis of oils with natural volatile constituents or short-chain fatty acids.
The pressurized differential scanning calorimetry (PDSC) is a technique that measures the amount of heat liberated in the oxidation reaction. The analysis is made directly on the sample using a heat flux differential between the sample and the thermocouple reference, with varying pressure and temperature. The PDSC curve registers heat flow as a function of time, determining the oxidation induction time (OIT) or determining the temperature oxidation onset (OOT) as a function of temperature. This technique uses smaller quantities of sample than the Rancimat method. The assay is reproducible and can be applied to any lipid composition [19–21].
Oven test is widely used in assessing the stability of lipids. In such case, the samples are examined at regular time intervals determining acidity index, peroxide index, iodine index, conjugated dienes and trienes dosage, among others. It is the closest evaluation of the real process of vegetable oil oxidation; however, there is no standardization for this test with respect to the storage time and the range of samples collected [16].
The techniques and methods mentioned above are classical procedures for oxidative stability testing of lipids. Each has advantages and disadvantages. They have in common the impossibility of registration of the structures of products from the chemical transformation of the constituents present in the oil. The 1H NMR is a technique that can give in minutes the fingerprint of a sample of lipid with excellent reproducibility, in addition to revealing the identity of oil oxidation products such as aldehydes and peroxides and monitoring the structural changes in the oil throughout the oxidation process. In polyunsaturated oils, for example, it is possible to observe and determine the variation of the molar percentage of PUFAS throughout the oxidation process, determining the n − 3 degradation in oils rich in this kind of acid [19, 21–25].
In the present study, thermal and oxidative stability of chia oil was evaluated by employing different methods with the objective of obtaining the maximum information concerning safe of storage conditions and use as food. The work also focused on the use of natural and synthetic antioxidants additives enhancers of oxidative stability of oil.
Experimental
Materials
Chia seeds (S. hispanica L.) were commercially acquired from Mexico. Rosemary leaves utilized in the preparation of rosemary extract (RE) were acquired in the city of Rio de Janeiro. Ethanol and deuterated chloroform were purchased from Tedia. The antioxidants were acquired from Sigma-Aldrich.
Methods
Oil extraction
Seed samples were ground, weighed and subjected to extraction according to Ixtaina and co-workers [12] with some modifications. In the process, n-hexane was used in a Soxhlet apparatus at reflux for 8 h. The solvent was removed using a rotary evaporator. The oil yield was expressed as extracted oil g/100 g of seed.
Fatty acid composition by 1H NMR
Fatty acid composition of chia oil was determined by 1H nuclear magnetic resonance measuring the areas by integration of the specific peaks according to the method utilized in the literature [26] applying the Eqs. (1)–(4). The result was expressed in molar percentage. 1H NMR spectra were acquired on a DRX-300 spectrometer from Bruker Co. The spectra acquisition time was 47 s with 16 repetitions in intervals of 1 s and pulse width of π/3. The vegetable oil samples were dissolved in CDCl3 at a concentration of 50 mg mL−1. TMS was used as internal standard. The composition was evaluated by integration of the peaks.
Preparation of rosemary extract
The rosemary leaves utilized for preparation of the extracts were first washed out and thus dried in an air circulation stove at the temperature of 60 °C for 24 h. The dried leaves were powdered in a blender, weighted (200 g) and submitted to the extraction with ethanol (300 mL) for 15 days. The extracts were filtered, and the solvent was removed by rotary evaporator. Rosemary extract (RE) was stored in an amber glass container in the refrigerator until use.
Addition of the antioxidants in the oils samples
The antioxidants were added to chia oil samples as described in Table 1. Butylated hydroxytoluene (BHT), tert-butylhydroquinone (TBHQ), α-tocopherol (α-T), propyl gallate (PG), caffeic acid (CA), ferulic acid (FA), vanillic acid (VA), syringic acid (SA), ellagic acid (EA), trans-cinnamic acid (t-C), rutin (R), quercetin (Q), gallic acid (GA) and rosemary extract (RE) were added to a concentration of 200 mg kg−1. Binary combinations (1:1, w/w) of RE with the other antioxidants were also tested at concentration of 200 mg kg−1.
Rancimat method
The oxidative stability of chia oil with and without antioxidant additives was determined according to AOCS Official Method cd 12b-92 AOCS 1997 [27], in a 873 Biodiesel Rancimat equipment from Metrohm using 2 g of oil at 110 °C and constant air flow of 10 L h−1. The result, expressed as induction period (IP), was automatically determined from the inflection point of the curve by the software that accompanies the equipment.
Pressurized differential scanning calorimetry (PDSC)
PDSC curve of pure chia oil was obtained by means of a pressurized differential scanning calorimeter, model DSC Q1000 equipment from TA Instruments, coupled to a pressure cell. The experiment was performed in the isothermal mode for various temperatures, utilizing an aluminum crucible, 10 mg of oil, under oxygen atmosphere with initial pressure of 1400 kPa and heating rate of 10 °C min−1 [28].
Schaal test
Schaal oven test was undertaken according to the literature [29] with minor modifications. 15 mL of chia oil in glass container without antioxidant additives was maintained within the oven to allow the direct contact with air and stored at 60 ± 2.5 °C for a total of 16 days. Samples were analyzed after 1, 2, 5 and 16 days to perform the following profile fatty acid variation and molar percentage of α-linolenic by 1H NMR [26].
Monitoring the degradation of fatty acids in the oil heated at the frying temperature
The frying temperature stability test was performed at 180 °C frying temperature during 75 min on a heater plate. Sample of 15 mL was placed in a 50-mL uncapped glass flask that allowed direct contact with atmospheric air. The evaluation of the fatty acid profile of oil was made by 1H NMR [26] at time zero and every 15 min after the initiation of heating.
Results and discussion
Oil yield and fatty acid profile
The yield of chia oil obtained in this study was 27 g of oil/100 g of seed. This result is in agreement with previous reports from the literature [11, 12]. Figure 1 shows the 1H NMR spectra of chia oil. Table 2 summarizes the characteristic chemical shift assignments for oil triacylglycerides [30]. The analysis of 1H NMR spectra revealed 11 signals in the region between 0.5 and 5.5 ppm. The signal at 0.68 ppm was attributed to the presence of stigmasterol phytosterols. The other signals were attributed to oil triacylglycerides. The fatty acid profile was expressed in molar percentage of α-linolenic, linoleic, oleic and saturated fatty acids determined using equations Eqs. (1)–(4), and the integrals values are shown in Table 2. The molar percentage of fatty acids obtained for the chia oil was 64.04% for α-linolenic acid, 16.18% for linoleic acid, 4.80% for oleic acid and 14.98% for saturated acids.
There are few studies on chia oil and no reports on the determination of chia oil fatty acid composition by 1H NMR. The comparison between the fatty acids compositions of chia oil determined by GC in previous reports [12, 31, 32] and the results presented herein obtained by 1H NMR shows little difference. However, we must take in mind that to perform a GC analysis of a vegetable oil sample it is necessary to obtain the corresponding methyl esters first and this may produce interference on the analysis result. On the other hand, 1H NMR spectroscopy demands no prior esterification for vegetable oil analysis. Another advantage of 1H NMR is the convenient way to determine the α-linolenic acid contents, which is possible because of a unique feature of its 1H NMR spectrum: the terminal methyl group from this type of fatty acid chain gives a distinct triplet signal at 0.98 ppm that does not appear in any other. So it is a matter of simple calculation to use terminal methyl 1H NMR integrals to determine the α-linolenic acid contents in a vegetable oil sample. By way of 1H NMR analysis, we obtained n − 3/n − 6 fatty acid ratio of 3.96 in accordance with the previous report from the literature [12] that uses GC and HPLC. This value is significantly higher than for any other vegetable oil [33], and so the chia oil may be a good natural source for incorporation into foods for human diet [34].
Oxidative stability
The oxidative stability of chia oil and the protective effect of the individual antioxidants by Rancimat method are shown in Table 3 (first and second columns). The induction period (IP) of antioxidant-free sample was 1.25 h which is compatible with oil rich in PUFAS, especially n − 3. Among the antioxidants tested, BHT and mainly TBHQ proved to be the most efficient. The natural antioxidants produced no relevant effects. In a previous publication about chia oil was evaluated the effectiveness of some natural antioxidants such as tocopherols, rosemary extract, green tea extract and ascorbyl palmitate. According to the authors, with the exception of tocopherols, the antioxidants were effective but when applied in high concentrations (5000 ppm) [32]. The aforementioned study did not examine the effect of synthetic antioxidants commonly used in the industry of vegetable oils such as BHT and TBHQ. In fact, there is a controversy among researchers in the case of natural antioxidants because some believe there is no need to limit the use in food, while others believe that there should be a limitation in the same way that there is for substances of synthetic origin [33, 34]. In 2004, the European Food Safety Authority (EFSA) reported no scientific support for the non-use of synthetic antioxidant TBHQ, and the same happened in 2011 and in 2012 to the synthetic antioxidants BHA and BHT. Usually, antioxidant additives are added to food products in small amounts so as not to interfere in the organoleptic properties [35]. Thus, in our study the natural or synthetic antioxidants were added in low concentrations in order to find those that are effective for chia oil.
Some antioxidants are potentiated when used in combination with another in a synergistic effect [32]. In this study, we verified the antioxidant effect of RE combined with the other antioxidants (Table 3, third and fourth columns). The results presented herein indicate that only the binary combination of RE with TBHQ considerably improves the oil oxidative stability compared to RE alone, probably more due to the effectiveness of the synthetic substance than due to synergistic effect. It must be noted that in the binary mixture was added 100 mg kg−1 of each antioxidant, while 200 mg kg−1 was added in the individual test. Usually, TBHQ is the most efficient antioxidant for vegetable oils including chia as shown in the present work, but its use is limited by regulatory laws of public health. In Brazil, according to the Ministry of Health, the maximum permitted concentration of TBHQ is 200 mg kg−1 [36].
In a recently published study, the authors applied PDSC technique for predicting the oxidative stability of foods rich in unsaturated fatty acids during storage [37]. In the present study, we use the PDSC for predicting the oxidative stability of an oil rich in PUFAs n − 3 during storage. According to the result, in the study of oxidative stability of chia oil at 60 °C, isothermal mode, Fig. 2, it was found that the chia oil does not undergo oxidative degradation in the oxidizing conditions of the experiment, even when exposed for long periods such as 600 min. This result is very important when compared with indexes of iodine, acidity, peroxide, diene and anisidine, physicochemical parameters evaluated in Schaal test, that are not reproducible.
Schaal test and gas chromatography (GC) can also be used in the evaluation of oxidative stability of vegetable oils by monitoring the decomposition of fatty acids, but it requires pretreatment of the sample (preparation of fatty acid esters, extraction and drying). In both, the errors arising from the various stages of manipulation of the sample can compromise the result. In the present study, the composition of fatty acids of chia oil submitted to the oven test was determined by 1H NMR spectroscopy. No pretreatment is needed, but it is required only to dissolve the sample in deuterated solvent and obtain the spectrum. Figure 3 shows the 1H NMR spectra obtained during 16 days of storage.
Through the 1H NMR analyses, various types of hydrogen atoms in the triglyceride molecules can be determined. The 1H NMR spectrum of chia oil at room temperature (spectrum A of Fig. 3) revealed 10 signals in the region between 0.0 and 5.5 ppm. The intensity of b signals (α-linolenic terminal methyl group) shows the high proportion of n − 3 present in the oil. 1H NMR spectra B, C, D and E were obtained from chia oil stored in open containers in an oven at 60 °C with air circulation for 24, 48, 120 and 384 h, respectively. Qualitative analysis of these spectra does not show differences in relation to the signals of the olefin, allyl and bis-allyl hydrogens. The relationship between the hydrogen signals a and b also seems unchanged. The spectra did not reveal the presence of products derived from the degradation of fatty acids, which agrees with the result of the analysis PDSC isotherm at 60 °C (Fig. 2) in which the oil remains unchanged for 600 min in an oxidizing atmosphere and elevated pressure.
The PDSC exothermic analysis performed at 80, 100, 110, 120, 160 and 180 °C temperatures (Fig. 4) showed different behaviors when compared to the analysis at 60 °C.
At 80 °C temperature, the oil starts the oxidation process 303.86 min after exposure to oxidizing and high-pressure atmosphere. This experiment oxidation induction time (OIT) value is 359.95 min. The PDSC curves at 100 °C, as expected, showed a decreased value compared with analysis at 80 °C, and a shorter time interval between the stages of initiation and termination of the oxidation. Using a temperature of 110 °C, the same temperature at which the Rancimat experiment was performed, result in OIT of 49.40 min and the time interval between the initiation and termination steps of the experiment was equivalent to the experiment performed at a temperature of 100 ºC, In a previous study and under the same conditions, a sample of sunflower oil containing 56.9% linoleic acid without the presence of linolenic acid results in OIT of 65.0 min [38]. The difference of about 15 min suggested a poorer oxidative stability of the chia oil. To reinforce this, we can use Eq. (5) that is developed based on the kinetic study of the oxidation of vegetable oils to predict the tendency to oxidation (OX) of oils containing unsaturated fatty acids [39]. In the present, the oxidative value of chia oil results in 1.35, while according to a previous report for sunflower oil it results in 0.57 [38]. These two values indicate that chia oil has almost three times greater tendency to oxidation than sunflower oil, based on fatty acid composition. The OIT values at 120 and 160 °C result in 17.95 and 10.07 min, respectively. According to the graph, at 160 °C the decomposition of the primary oxidation products was very fast, practically with no interval between the initial and final phases of oxidation. The PDSC analysis performed at 180 °C showed no difference with the isotherm at 160 °C.
PDSC and Rancimat are rapid techniques for assessing the oxidative stability of fatty acids. Both techniques involve drastic conditions of sample exposure and differ from the usual conditions to which the samples are submitted. In the PDSC, exothermic events are measured, and in the Rancimat method the conductivity of the volatiles formed during oxidation is measured [28]. The spectroscopic methods have been developed for the purpose of detecting the profile of degradation in the food composition. The 1H NMR is capable of detecting difference in the profile of the fatty acids before and after the lipid oxidation. In the present study, we employed the 1H NMR to determine the profile of degradation of chia oil submitted to frying temperature, a drastic condition for an oil rich in n − 3. Figure 5 shows spectrum of unheated chia oil (A) and chia oil heated at 180 °C and sampled at 15-min intervals (B–F). Chia oil begins degradation after 15 min of heating. In the sequence, this degradation leads to an inversion between the intensities of signal a and b indicating α-linolenic degradation. The figure also shows that the g signal, attributed to bis-allylic hydrogens, gradually decreases from spectrum A to F. Heating at 180 °C for 75 min (spectrum F of Fig. 5) caused the loss of about 75% molar percentage of α-linolenic acid. This result is in agreement with the oxidative behavior observed in PDSC analysis at 180 °C in which the oil degrades rapidly, and suggests that chia oil is not suitable to frying conditions.
Although 1H NMR spectra of the heated oil, shown in Fig. 5, only shown in the degradation of the polyunsaturated fatty acids; however, extreme changes occur in the composition of the heated oil in the frying temperature, such as the formation of free fatty acids, di-and monoacylglycerols, glycerol and a number of toxic products inappropriate for human consumption.
Conclusions
In this work, the 1H NMR spectroscopy proved to be very useful and simple to evaluate the composition and thermal stability of chia oil. This vegetable oil is regarded as the richest natural source of α-linolenic acid. It was shown that it is stable at 60 °C by Schaal tests and PDSC analysis. The evaluation of oxidative stability by Rancimat method showed that TBHQ is an excellent antioxidant additive with excellent protection for chia oil. In relation to binary mixtures with rosemary extract, a natural antioxidant, this work showed that only with TBHQ we have slight improvement in the oil oxidative stability, but probably it is not a synergistic effect. The evaluation of the oil heated at frying temperature showed that it is unstable at 180 °C and its consumption in these conditions poses a risk to consumer health.
References
Theriault A, Chao J, Wang Q, Gapor A, Adellik K. Tocotrienol: a review of its therapeutic potential. Clin Biochem. 1999;32:309–19.
Saldeen K, Saldeen T. Importance of tocopherol beyond α-tocopherol: evidence from animal and human studies. Nutr Res. 2005;25:877–89.
Shahidi F, Wanasundara UN. Omega-3 fatty acid concentrates: nutritional aspects and production of technologies. Trends Food Sci Technol. 1998;9:230–40.
Boran G, Karacam H, Boran M. Changes in the quality of fish oils due to storage temperature and time. Food Chem. 2006;98:693–8.
Kolanowski W. Seasonal variability in intake of fish oil dietary supplements among inhabitants of Warsaw. Nutr Res. 2008;28:245–50.
Baum SJ, Kris-Etherton PM, Willett WC, Lichtenstein AH, Rudel L, Maki KC, et al. Fatty acids in cardiovascular health and disease: a comprehensive update. J Clin Lipid. 2012;6:216–34.
Burr M, Gilbert I, Rogers S, Holliday RM, Sweemam PM, Elwood PC, Deadman NM. Effects of changes in fat, fish and fibre intake on death and myocardial reinfarction. Diet and Reinfarctional trial (DART). Lancet. 1989;334:757–61.
Connor W. α-Linolenic acid in health and disease. Am J Clin Nutr. 1999;69(5):827–8.
WHO/FAO. Fats and oils in human nutrition. FAO. 1994.
Ayerza R, Coates W. Composition of chia (Salvia hispanica) grown in six tropical and subtropical ecosystems of South America. Trop Sci. 2004;44:131–5.
Ayerza R. Oil content and fat acid composition of chia (Salvia hispanica L.), from five northeastern locations in northwestern Argentina. J Am Oil Chem Soc. 1995;72:1079–81.
Ixtaina VY, Martínez ML, Spotorno V, Mateo CM, Maestri DM, Diehl BWK, Nolasco SM, Tomas MC. Characterization of chia seed oils obtained by pressing and solvent extraction. J Food Comp Anal. 2011;24(2):166–74.
Shahidi F, Zhong Y. Lipid oxidation and improving the oxidative stability. Chem Soc Rev. 2010;39:4067–79.
Smouse TH. Factors affecting oil quality and stability. In: Warner K, Eskin NAM. Methods to assess quality and stability of oils and fat—containing foods. Champaign, IL, AOCS, 1995.
Dobarganes MC, Velasco J. Analysis of lipid hydroperoxides. Eur J Lipid Sci Technol. 2002;104:420–8.
Frankel EN. In search of better methods to evaluate natural antioxidants and oxidative stability in food lipids. Trends Food Sci Technol. 1993;4(7):220–5.
AOCS, Official Methods and Recommended Practices of the American Oil Chemists’ Society: fats, oils and lipid related analytical methods. Champaign, USA, 6ed. AOCS Press, 2009.
Akoh C. Oxidative stability of fat substitutes and vegetable oils by the oxidative stability index method. J Am Oil Chem Soc. 1994;71(2):211–6.
Levy P. Pressure differential scanning calorimetry. Thermochim Acta. 1970;1:429–39.
Kodali DR. Oxidative stability measurement of high-stability oils by pressure differential scanning calorimeter (PDSC). J Agric Food Chem. 2005;53(20):7649–53.
Dunn RO. Oxidative stability of biodiesel by dynamic mode pressurized-differential scanning calorimetry (PDSC). Am Soc Agric Biol Eng. 2006;49(5):1633–41.
Sacchi R, Falcigno L, Paduano A, Ambrosino ML, De Giulio B, Addeo F, Paolillo L. Quantitative evaluation of the aldehydes formed in heated vegetable oils using high resolution proton-NMR spectroscopy. Riv Ital Sostanze Gr. 2006;83:257–63.
Tyl EC, Brecker L, Wagner KH. 1H NMR spectroscopy as tool to follow changes in the fatty acids of fish oils. Eur J Lipid Sci Technol. 2008;110:141–8.
Guillén DM, Ruiz A. Monitoring of heat—induced degradation of edibles oils by proton NMR. Eur J Lipid Sci Technol. 2008;110:52–60.
Guillén MD, Uriarte PS. Monitoring by 1H nuclear magnetic resonance of the changes in the composition of virgin linseed oil heated at frying temperature. Comparison with the evolution of other edible oils. Food Control. 2012;28:59–68.
Kirby CW, McCallum JL, Fofana BA. 1H NMR study of the fatty acid distribution in developing flax bolls before and after a cooking treatment. Can J Chem. 2011;89:1138–42.
American Oil Chemist’s Society Official Method Cd-12b-92. Am oil Chem Soc Champaign IL, 1993.
Nascimento JA, Araujo KLGV, Epaminondas PS, Pontes ALS, Souza AL, Queiroz N, Souza AG. Ethanolics extracts of Moringa: antioxidant effect in soybean oil by PDSC and Rancimat. J Therm Anal Calorim. 2014;117:265–8.
Fennema OR. Principles of food science, part i, food chemistry. New York: Marcel Dekker Inc; 1976.
Andrade DF, Mazzei JL, Kaiser CR, d’Avila LA. Assessment of different measurement methods using 1H-NMR data for the analysis of the transesterification of vegetable oils. J Am Oil Chem Soc. 2012;89:619–30.
Ayerza R, Coates W. Protein content, oil content and fatty acid profiles as potential criteria to determine the origin of commercially grown chia (Salvia hispanica L.). Ind Crop Prod. 2011;34:1366–71.
Ixtaina VY, Nolasco SM, Tomás MC. Oxidative Stability of Chia (Salvia hispanica L.) Seed Oil: effect of Antioxidants and Storage Conditions. J Am Oil Chem Soc. 2012;89:1077–90.
Tuberoso C, Kowalczyk A, Sarritzu E, Cabras P. Determination of antioxidant compounds and antioxidant activity in commercial oilseed for food use. Food Chem. 2007;103:1494–501.
Ganesan B, Brothersen C, McMahon DJ. Fortification of foods with omega-3 polyunsaturated fatty acids. Crit Rev Food Sci Nutr. 2014;54:98–114.
Halliwell B, Aeschbach R, Löliger J, Aruoma OI. The characterization of antioxidants. Food Chem Toxicol. 1995;33(7):601–17.
Ramalho VC, Jorge N. Antioxidants used in oils, fats and fats food. Quim Nova. 2006;29(4):755–60.
Holda K, Glogowski R. Selected quality properties of lipid fraction and oxidative stability of dry dog foods under typical storage conditions. J Therm Anal Calorim. 2016;126:91–6.
Cordeiro AMTM, Medeiros ML, Santos NA, Soledade LEB, Pontes LFBL, Souza AL, Queiroz N, Souza AG. Rosemary (Rosmarinus officinalis L.) extract: thermal study and evaluation of the antioxidant effect on vegetable oils. J Therm Anal Calorim. 2013;113:889–95.
We N, Selke E, Mounts TL, Rinsch W, Frankel EN, Zeiton M. Effect of triacylglycerol composition and structures on oxidative stability of oils from selected soybean germplasm. J Am Oil Chem Soc. 1992;69:111–8.
Acknowledgements
The authors acknowledge The Brazilian agencies Conselho Nacional de Desenvolvimento Científico e Tecnológico (CNPq) for the financial support.
Author information
Authors and Affiliations
Corresponding authors
Rights and permissions
About this article
Cite this article
Souza, A.L., Martínez, F.P., Ferreira, S.B. et al. A complete evaluation of thermal and oxidative stability of chia oil. J Therm Anal Calorim 130, 1307–1315 (2017). https://doi.org/10.1007/s10973-017-6106-x
Received:
Accepted:
Published:
Issue Date:
DOI: https://doi.org/10.1007/s10973-017-6106-x