Abstract
Superconducting thick films of Bi2Sr2Ca0.95M0.05Cu2.0O8+δ (Bi-2212) with M = K, Li, and Al were fabricated by a dip coating process. The influences of different doping elements on the lattice structure, electrical transport properties and related superconducting properties were systematically investigated. XRD analyses confirmed that all the doping ions have successfully entered into the Bi-2212 matrix, and led to a systematical change of lattice parameters. Due to the change of thermodynamic properties with doping, the phase evolution process changed during the sintering process. Thus both the content of secondary phases and the size of Bi-2212 crystals changed, which influenced the intergrain connections. The carrier concentrations of thick films were also influenced by doping, which contributed to the changes of critical temperature. It can be deduced that the hole doping with either K+ or Li+ can tune the samples into overdoping region, thus led to the increase of critical current at 77 K with the maximum critical current density of ~5300 A/cm2.
Similar content being viewed by others
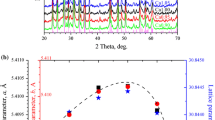
Avoid common mistakes on your manuscript.
1 Introduction
Since the first discovery of Bi2Sr2CaCu2O8+δ (Bi-2212), it has been considered to be one of the most promising high-temperature superconductors (HTS) for practical applications due to its excellent properties including the high irreversibility field beyond 100 T [1] and high critical current density under high magnetic field [2]. Meanwhile, as the only HTS so far, which can be made into round wires with isotropic cross sections, it can greatly simplify the winding process of cables and magnets. Therefore, it shows great potential for the applications as insert coils in high field magnets and Rutherford cables for accelerators [3–9]. Recently, the maximum total magnetic field of 33.8 T was successfully achieved under the background field of 31.2 T with Bi-2212 insert coils [10] even with a defected terminal, which demonstrated the large potential of Bi-2212 conductors in practical applications.
Aiming at the practical applications, many investigations focused on the studies of Bi-2212 tapes (wires) [11–14] and thick films [15–17]. Elementary doping was proved to be an effective way for the optimization of superconducting properties by tuning the lattice structure, microstructure, thermodynamic behaviors, and electrical/magnetical properties of Bi-2212. Pb substitution at Bi site of Bi-2212 was considered to be the most successful example [18–21]. The introduction of Pb could result in the decrease of the lattice parameter c of Bi-2212, which not only reduced the anisotropic behavior of superconducting properties, but also introduced the effective flux pinning centers to enhance the in-field current capacity. Therefore, the superconducting critical parameters were effectively optimized. Rare earth (RE) elements doping on the Sr/Ca site of Bi-2212 have also been studied [22–31]. RE ions, such as Gd3+, Ho3+, Yb3+ and Eu3+ could work as effective pinning centers to enhance the flux pinning properties, and lead to an obvious increase of critical current density.
In our study, aiming at the optimization of superconducting properties of Bi-2212 and researches of the doping effects of different elements, Bi-2212 superconducting thick films with both hole doping by K and Li, and electron doping by Al were fabricated. The influences of all the doping ions on the phase composition, microstructure, electronic properties and related superconducting properties were systematically investigated.
2 Experimental details
Precursor powders Bi2Sr2Ca0.95M0.05Cu2.0O8+δ (Bi-2212) with M = K, Li, and Al were prepared by modified co-precipitation process [32] with the starting materials of Bi2O3, SrCO3, CaCO3, and CuO (>99.9 %). KOH, LiOH, and Al2O3 powders with the atomic ratio of 0.05 were added respectively as dopant before the calcination process. Single phased Bi-2212 precursor powders with different doping elements were obtained after a series of calcination processes in air at 800 °C/12 h, 820 °C/20 h, and 850 °C/20 h with intermediate grinding. The final powders were milled and sieved into 425 meshes. The paste for coating was made by mixing the precursor powders, organic binder (polyvinyl butyral) and solvent (ethanol) with the weight ratio of 10:0.1:9. Meanwhile, Ag substrates with the dimension of 4 × 20 × 0.2 mm were ultrasonically cleaned in acetone for 15 min. Bi-2212 thick films were obtained by dipping the substrate into the paste and lifting up with constant speed of 2 cm/s with self-made lifting device. The thick films were heated at 300 °C for 2 h to get rid of the organics. For the partial melting process, the films were heated to T max of 892 °C with a constant heating rate of 5 °C/h and dwell time of 20 min, then cooled down to 840 °C and annealed for 20 h. Then the final films with the thickness of 40–60 μm were obtained.
Polycrystalline X-ray diffraction (XRD) patterns on both precursor powders and thick films were taken on an XRD (Bruker D8 Advance) with Cu-Kα radiation (λ = 0.15406 nm). Lattice parameters were estimated with Reitveld refinement by Fullprof®. Texture degrees of (00l) peaks were mostly used to evaluate the quality of texture structures, which were calculated as following,
where ∑I 00l represents intensity sum of all (00l) peaks of the Bi-2212 phase, ∑I is the intensity sum of all diffraction peaks of the pattern. Scanning electron microscopy (SEM) images were obtained with JEOL JSM-6700F. Thermopower values were measured on ZEM-2 within the temperature range of room temperature to 150 °C with the temperature difference of 10 °C. Four thermopower values were taken at one temperature to obtain the average value. Temperature dependences of resistance were measured on a computer-aided apparatus using a DC four-probe method with the input current of 1 mA and the temperature ranging from 81 to 120 K in order to obtain the critical temperature, T c. Critical current values, I c, were measured at liquid nitrogen temperature (77 K) on the same equipment with the criterion of 1 μV/cm. And critical current density, J c, as J c = I c/A, was calculated with I c and the cross section area, A, which was obtained with SEM images.
3 Results and discussion
As shown in Fig. 1a, the XRD patterns of precursor powders with different doping elements all exhibit a near single phased composition, which can be indexed into orthorhombic Bi-2212 structures. Shifts of diffraction peaks comparing with that of undoped powders, which can be observed clearly by zooming in the diffraction peaks of (115), as shown in the inset, suggest the changes of lattice parameters. After Reitveld refinements, the lattice parameters are obtained as plotted in Fig. 1b and listed in Table 1. It can be noticed that the lattice parameters a and b are almost the same for each sample, suggesting a near tetragonal lattice structure. After Li and K doping, the lattice parameters change with the ion radii of doping elements correspondingly. It suggests that these ions enter into the Bi-2212 matrix successfully. On the other hand, after Al doping, the lattice parameters increase obviously. Thus it can be deduced that the Al3+ ions enter into Bi-2212 matrix as interstitial ions due to the extra small ion radius of Al3+ = 0.51 Å.
Dip coating process is adopted for the fabrication of Bi-2212 thick films with different doping elements. XRD patterns of thick films after the partial melting process are plotted in Fig. 2. After the heat treatment processes, Bi-2212 is still the major phase in all these films. While, it is noteworthy that the contents of secondary phases including both Bi2Sr2CuO8 (Bi-2201) and alkali earth cuprates (AEC, (Ca, Sr) x Cu y O z ) phase increase after doping. It suggests that the doping elements into Bi-2212 matrix can influence the phase evolution process thus lead to the change of phase composition in final thick films. Meanwhile, (00l) texture is obtained in the films after sintering. After the calculation with Eq. 1, texture degree values are obtained as listed in Table 1. Maximum texture degree of 93.1 % is obtained for the undoped samples. After doping, texture degrees of all the samples decrease, which is attributed to the increase of the content of secondary phases. On the other hand, the full-width at half maximum (FWHM) values are also estimated based on the XRD pattern, as listed in Table 1. Based on the analyses with Scherrer equation, increasing FWHM value of (008) peak from 0.145° to 0.195° suggests a decrease of average particle size of Bi-2212 phase after doping. Both the increase of secondary phase content and the decrease of average Bi-2212 particle size can imply the weakening of intergrain connections.
SEM images of the surfaces of the doped thick films are shown in Fig. 3a–d. Smooth surface is observed after the partial melting process. Most of the plates like Bi-2212 grains align with the ab plane parallel to the surface. However, due to the interferences of secondary phases after doping, some Bi-2212 grains are not perfectly aligned, which leads to the decrease of texture degree. Comparing with the undoped film, more secondary phase particles can be observed as marked on the images with EDX analysis. Thus more misaligned grains are observed on the surfaces of thick films after doping.
One important effect of doping is the changing of carrier concentrations. In cuprates HTS, the main carriers are holes [33]. According to the previous report by Tallon et al. [34], for small hole concentration per Cu ion, p, (p < 0.2), the following equation is used to estimate the hole concentration values with the room temperature thermopower:
where, k B and e are the Boltzmann constant and the electron charge, respectively, S is thermopower with the unit of μV/K, the extra ln2 term comes from the orbital degrees of freedom (assuming two fold orbital degeneracy). This equation is derived using a single-band Hubbard model with the assumption that the bandwidth is much less than k B T. Therefore, by measuring the thermopower values, it is possible to study the carrier concentration change and electrical transport behavior of the normal state qualitatively.
Thermopower values of the thick films are measured and the values at 25 °C are adopted for the calculation of carrier concentration. As listed in Table 1, the carrier concentration increases with K and Li doping, and decreases with Al doping. It is well-known that the critical temperature is very sensitive to the carrier concentration. Thus the change of carrier concentration discussed above can lead to a corresponding change of critical temperature. As shown in Fig. 4, the resistance of Bi-2212 thick films are measured as a function of temperature with transport method. And the critical temperture can be deduced. It can be observed that the critical temperatures decrease slightly with K and Li doping from 86.0 K to 85.2 and 85.5, respectively. And the critical temperature increases with Al doping to 90.7 K. This change of critical temperature suggests that the undoped film is in overdoping region after partial melting process. The increase of carrier concentration with K and Li doping tune the samples into strong overdoping region thus leads to the decrease of critical temperature, and the decrease of carrier concentration with Al doping tune the films into optimal doping region.
Critical current values of Bi-2212 thick films are measured with transport method and the V–I curves are plotted in Fig. 5. The critical current values are obtained with the criteria of 1 μV/cm as listed in Table 1. By measuring the area of cross section, critical current density values are also obtained and listed in Table 1. It is noticed that K and Li doping can enhance the current capacity of Bi-2212 films obviously. Considering the weakened intergrain connections after sintering, the increasing critical current density should be attributed to the doping effect on Bi-2212 phase. It is reported that the fabrication of overdoping Bi-2212 superconductors with oxygenation treatments could enhance the current capacity [35]. Thus, in this study, hole doping Li and K both can tune superconductors into overdoping region, thus lead to the enhancement of current capacity.
4 Conclusions
Bi-2212 superconducting thick films with different doping elements of K, Li, and Al were fabricated with a dip coating process. The influences of doping elements on lattice structure, microstructure and related superconducting properties were investigated systematically. The results showed that all the element doping affected the phase evolution process of Bi-2212 films during the partial melting process. The content of secondary phases increased and the average particle size of Bi-2212 phase decreased after doping, both of which weakened the intergrain connections. The other effect of doping was tuning the carrier concentrations, thus the critical temperature changed correspondingly. The critical current density increased after Li and K doping. Considering the weaker intergrain connections after doping, the enhanced critical current density from ~3800 to ~5300 and 4700 A/cm2 after K and Li doping should be attributed to the element doping into Bi-2212 matrix. The results of this study proved the positive doping effect of Li and K to obtain overdoping Bi-2212 with higher current capacity. With further optimization of the fabrication process, especially the partial melting process for Li or K doped samples, it is possible to obtain Bi-2212 thick films or even Bi-2212 multifilament wires with higher current capacity for practical applications.
References
A.S. Alexandrov, V.N. Zavaritsky, W.Y. Liang, P.L. Nevsky, Phys. Rev. Lett. 76, 983 (1996)
H. Miao, K.R. Marken, M. Meinesz, B. Czabaj, S. Hong, IEEE Trans. Appl. Supercond 15, 2554–2557 (2005)
D.C. Larbalestier, J. Jiang, U.P. Trociewitz, F. Kametani, C. Scheuerlein, M. Dalban-Canassy, M. Matras, P. Chen, N.C. Craig, P.J. Lee, E.E. Hellstrom, Nat. Mater. 13, 375–381 (2014)
H.M. Weijers, U.P. Trociewitz, W.D. Markiewicz, J. Jiang, D. Myers, E.E. Hellstrom, A. Xu, J. Jaroszynski, P. Noyes, Y. Viouchkov, D.C. Larbalestier, I.E.E.E. Trans, Appl. Supercond. 20, 576–582 (2010)
T. Kiyoshi, A. Sato, H. Wada, S. Hatashi, M. Shimada, Y. Kawate, I.E.E.E. Trans, Appl. Supercond. 10, 472–477 (1999)
Z. Melhem, S. Ball, S. Chappell, Phys. Procedia 36, 805–811 (2012)
M. Dalban-Canassy, D.A. Myers, U.P. Trociewitz, J. Jiang, E.E. Hellstrom, Y. Viouchkov, D.C. Larbalestier, Supercond. Sci. Technol. 25, 115015 (2012)
J. Bock, F. Breuer, C.E. Bruzek, N. Lallouet, M.O. Rikel, H. Walter, in WAMS 2004, ed. by L. Bottura, M. Buzio, T. Taylor (Archamps, 2004), pp. 149–155
K. Ohsemochi, K. Koyanagi, T. Kurusu, T. Tosaka, K. Tasaki, M. Ono, Y. Ishii, K. Shimada, S. Nomura, K. Kidoguchi, H. Onoda, N. Hirano, S. Nagaya, J. Phys. Conf. Ser. 43, 825–828 (2006)
P. Chen, U.P. Trociewitz, M. Dalban-Canassy, J. Jiang, E.E. Hellstrom, D.C. Larbalestier, Supercond. Sci. Technol. 26, 075009 (2013)
D. Bourgault, E. Flahaut, A. Antonevici, C.E. Bruzek, N. Lallouet, J.L. Soubeyroux, R. Tournier, Supercond. Sci. Technol. 17, 463 (2004)
D. Bourgault, E. Flahaut, A. Antonevici, R. Tournier, J.L. Soubeyroux, N. Lallouet, C.E. Bruzek, Cryogenics 44, 681–686 (2004)
S. Pavard, D. Bourgault, C. Villard, R. Tournier, Phys. C 316, 198–204 (1999)
J. Kase, K. Togano, H. Kumakura, D.R. Dietderich, N. Irisawa, T. Morimoto, H. Maeda, Jpn. J. Appl. Phys. 29, L1096 (1990)
D. Sager, L.J. Gauckler, M. Chen, Phys. C 420, 69–77 (2005)
J.A. Alarco, A. Ilushechkin, T. Yamashita, A. Bhargava, J. Barry, I.D.R. Machinnon, Mater. Sci. Eng. B 45, 102–107 (1997)
W. Zhang, E.E. Hellstrom, Phys. C 218, 141–152 (1993)
X.L. Wang, H.K. Liu, S.X. Dou, J. Horvat, D. Millikon, G. Heine, W. Lang, H.M. Luo, S.Y. Ding, J. Appl. Phys. 89, 7669 (2001)
A.L. Crossley, Y.H. Li, A.D. Caplin, J.L. MacManus-Driscoll, Phys. C 314, 12–18 (1999)
A.B. Kulakov, I.K. Bdikin, S.A. Zver’kov, G.A. Emel’chenko, G. Yang, J.S. Abell, Phys. C 371, 45–51 (2002)
H.L. Su, F. Vasiliu, P. Majewski, F. Aldinger, Phys. C 256, 345–352 (1996)
A. Amira, Y. Boudjadja, A. Saoudel, A. Varilci, M. Akdogan, C. Terzioglu, M.F. Mosbah, Phys. B 406, 1022–1027 (2011)
S. Vinu, P.M. Sarun, R. Shabna, P.M. Aswathy, J.B. Anooja, U. Syamaprasad, Phys. B 405, 4355–4359 (2010)
R. Shabna, P.M. Sarun, S. Vinu, U. Syamaprasad, J. Alloys Compd. 493, 11–16 (2010)
R. Shabna, P.M. Sarun, S. Vinu, U. Syamaprasad, J. Alloys Compd. 481, 797–801 (2010)
P.M. Saruna, S. Vinua, R. Shabnaa, A. Bijub, U. Syamaprasad, J. Alloys Compd. 472, 13–17 (2009)
S. Vinu, P.M. Sarun, R. Shabna, A. Biju, U. Syamaprasad, Mater. Lett. 62, 4421–4424 (2008)
S. Vinu, P.M. Sarun, A. Biju, R. Shabna, P. Guruswamy, U. Syamaprasad, Supercond. Sci. Technol. 21, 045001 (2008)
P.M. Sarun, S. Vinu, R. Shabna, A. Biju, U. Syamaprasad, Mater. Lett. 62, 2725–2728 (2008)
A. Biju, U. Syamaprasad, A. Rao, J.G. Xu, K.M. Sivakumar, Y.K. Kuo, Phys. C 466, 69–75 (2007)
X. Sun, X. Zhao, W. Wu, X. Fan, X.-G. Li, Phys. C 305, 227–232 (1998)
C.B. Mao, L. Zhou, X.Y. Sun, X.Z. Wu, Phys. C 281, 35–44 (1997)
T.D. Hien, N.K. Man, K.B. Garg, J. Magn. Magn. Mater. 262, 508–513 (2003)
J.L. Tallon, J.R. Cooper, P.S.I.P.N.D. Silva, G.V.M. Williams, J.W. Loram, Phys. Rev. Lett. 75, 4114–4117 (1995)
T. Shen, J. Jiang, A. Yamamoto, U.P. Trociewitz, J. Schwartz, E.E. Hellstrom, D.C. Larbalestier, Appl. Phys. Lett. 95, 152516 (2009)
Acknowledgments
This research was financially supported by National “973” Project, under contract No. 2011CBA00104, National Natural Science Foundation of China under contract No. 51472206,the international scientific and technological cooperation projects of China No. S2010GR0518, the national ITER program of China No. 2013GB110001, and the Innovative Research Team of Shaanxi province No. 2013KCT-07.
Author information
Authors and Affiliations
Corresponding author
Rights and permissions
About this article
Cite this article
Zhang, S., Li, C., Hao, Q. et al. Doping effects on Bi-2212 high temperature superconducting thick films. J Mater Sci: Mater Electron 26, 6380–6385 (2015). https://doi.org/10.1007/s10854-015-3226-6
Received:
Accepted:
Published:
Issue Date:
DOI: https://doi.org/10.1007/s10854-015-3226-6