Abstract
Lectins have potential applications in agriculture, medicine, and biotechnological research and have become an important tool in the identification of different blood groups. Lectins can be obtained from different sources, such as microalgae. Tetradesmus obliquus is a green microalga that produces bioactives with high added value. A novel lectin was purified from microalgae T. obliquus by ion exchange and gel filtration chromatographies and characterized by molecular mass determination; carbohydrate contents; recognition of the rabbit, bovine, horse, and ABO blood type; inhibition by carbohydrate and glycoprotein; optimum pH and temperature; and their stability. Lectin was purified with a purification factor of 4.2-fold and yield of 3.13 in a two-step using ion exchange and gel filtration. It agglutinated human type O and rabbit erythrocytes but not bovine, horse, human erythrocytes types A1, A2, B, and AB. Lectin showed optimum pH at 7.0–7.5 and stability for 90 min, while the optimum temperature of 20–50 °C showed fairly good thermostability. The lectin is a glycoprotein with 5.37% of carbohydrate, an apparent molecular mass of 78 kDa as determined by gel filtration, and the activity was decreasing in the presence of ions and inhibited only by glycoprotein azoalbumin, azocasein, and albumin, characteristics that indicate the lectin from T. obliquus as unique among microalgal lectins.
Similar content being viewed by others
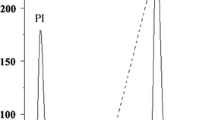
Avoid common mistakes on your manuscript.
Introduction
Lectins or hemagglutinins are carbohydrate-binding proteins/glycoproteins reversibly of non-immune origin with multiple potential applications in agriculture, medicine, and biotechnological research. It has been well documented that lectins play important roles in cellular and molecular recognition and have been exploited as carriers of chemotherapeutic agents, biomarkers of specific microorganisms, potential diagnostic reagents, and anticancer drugs, antiviral, antimicrobial, antiparasitic, anti-inflammatory, and larvicidal activity (Coelho et al. 2017).
In addition, lectins seem comparable to antibodies in their sensitivity and this property is useful in clinical fields. It shows a highly specific reaction to different sugar heads that are present on the erythrocyte surface membrane, bound to either glycoprotein or glycolipids, specifying ABO or MN blood groups. Recently, a limited number of lectins have been used in the recognition of blood groups. In the ABO blood group especially, lectins from Dolichos biflorus, Vicia cracca, Phaseolus limensis, Otala lactea, and Crotalaria striata potentially recognize blood group A substance (Khan et al. 2002), but only lectins from Dolichos biflorus are considered Anti-A1 commercially.
Lectins are widespread in distribution and have been isolated from viruses, bacteria, fungi, insects, plants, algae, and vertebrates. Lectins purified from different species or even the same species may show differences in structure, molecular size, and carbohydrate specificity, resulting in different biological properties.
Plant lectins have been intensively studied. However, lectins extracted from photosynthetic microorganisms are less known. In the literature, there are reports of lectins extracted from microalgae such as Nostoc, Microcystis, Oscillatoria, Scytonema, Chlorella, Scenedesmus, Desmodesmus, and Chlorococcum. Although considerable progress has been made in understanding the distribution and biochemical characteristics of microalgal lectins, much less is known about algal lectins than those derived from plants. This means that the utilization of algal lectins is not as well advanced as lectins from other sources.
Tetradesmus obliquus is a green microalga (previous name = Scenedesmus acutus (Wynne and Hallan 2016)). This group of microalgae (Chlorophyceae, Scenedesmaceae) may be a promising source of lectins as it has been shown to produce a huge variety of bioactive products with potential commercial values, such as lipids, carbohydrates, proteins, vitamins, amino acids, and beta-carotene that are used as feedstock for energy production, feed additives, food, cosmetics, and medicine (Ishaq et al. 2016). In addition, microalgal bioprocesses have been highlighted because they do not compete with food crops as requiring arable land and can use waste as nutrients for their development.
In the present study, a lectin from T. obliquus was purified, characterized, and evaluated for its potential in the agglutination of the difference in blood group.
Materials and methods
Microorganisms and culture conditions
Tetradesmus obliquus (A5F5402) was isolated from Açude of Apipucos (Recife, Pernambuco, Brazil, coordinates 8° 1′ 13.08″ S; 34° 55′ 56.51″ W) and cultivated in 250-mL Erlenmeyer flasks each containing 50 mL BG-11 medium at 30 ± 1 °C, continuous light of 52 ± 4 μmol photons m−2 s−1, under constant aeration for 15 days, when it reached the end of the logarithmic growth phase. Then, cells were removed by filtration, dried, and used for further analysis.
Lectin extraction
Dried biomass was homogenized at a concentration of 100 mg mL−1 in Tris-HCl-buffered saline (0.2 M, pH 7.2), using a magnetic homogenizer for 9 h at 4 °C (Chu et al. 2006). The homogenate was centrifuged at 10000×g for 8 min at 4 °C. The supernatant was the crude extract of T. obliquus.
Precipitation step
Crude extract was precipitated by adding ammonium sulfate slowly to the clarified cell extract with gentle stirring at 4 °C until the solution was saturated at a concentration of 0–60% and 60–80% (w/v). The protein precipitated by centrifugation (8000×g for 30 min) was dissolved in 10 mM Tris-HCl buffer (pH 7.5) and dialysed against the same buffer for 18 h at 4 °C. After dialysis, hemagglutinating activity and protein content of each fraction were estimated.
Purification and molecular mass determination by size-exclusion chromatography of Tetradesmus obliquus lectin
Tetradesmus obliquus lectin was purified using two-step chromatography using ion exchange and gel filtration. Supernatant (crude extract) of T. obliquus was loaded on to anion-exchange chromatography using DEAE Sephadex A-50 column (1.6 cm × 10 cm) pre-equilibrated with 20 mM Tris-HCl buffer pH 7.2. The unabsorbed fraction was eluted with the equilibration buffer. Elution was carried out at a flow rate of 1 mL min−1, and the absorbance was monitored at 280 nm. The peak was pooled, concentrated, and was used to determinate protein total and hemagglutinating activity.
Fractions exhibiting hemagglutinating activity were pooled, concentrated, and injected into on FPLC-Superdex G-75 HR10/300GL fast flow column (1.0 cm × 80 cm) (AKTA avant 25, Sweden) pre-equilibrated with 10 mM Tris-HCl buffer (pH 7.0). The elution rate was 0.5 mL min−1, and the absorbance was determined at 280 nm. Peaks were used to determinate protein total and hemagglutination activity. Peak with hemagglutination activity was reloaded in the same conditions to obtain lectin purified. Weight determination was achieved using Gel Filtration Markers Kit for protein molecular weights 6500–66,000 Da from Sigma-Aldrich.
Hemagglutinating activity assay
Hemagglutinating activity was measured with erythrocytes treated with glutaraldehyde using the method of Correa and Coelho (1995). Twofold serial dilutions of the sample (50 μL) were mixed with 2.5% (v/v) erythrocyte buffer solution (50 μL) at 25 °C in a 96-well microtiter plate. Saline was used as the blank control, and the results were recorded after incubation at 25 °C for 45 min. Lectin activity was determined by its ability to agglutinate erythrocytes. Hemagglutination was recorded visually, and mat formation indicated the presence of lectin activity, while button formation at the bottom of cavity showed the absence of lectin activity. Serial twofold dilutions of the sample were used to determine the hemagglutinating titer (U).
Protein concentration
The concentration of total protein was measured by the method of Smith et al. (1985), using bovine serum albumin as the standard.
Erythrocytes sample
Erythrocytes were obtained from the blood samples of rabbit, bovine, horse, and human (A subgroup 1, A subgroup 2, B, AB, and O). Blood was mixed with 1/10 volume of 3.7% Na-citrate and centrifuged at 8000×g for 20 min and stored until use.
The recognition of subgroup A1 was performed using the tube technique (Fresenius HemoCare Brasil Ltda, Itapecerica da Serra, São Paulo, Brasil. 2017). A 2–3% suspension of red blood cells (erythrocytes) was washed 3 times in PBS solution and added Anti-A1 Lectin reagent (Dolichos biflorus) (Lorne Laboratories, UK) in a test tube as the positive control.
Carbohydrate analysis
The carbohydrate content of the lectin was analyzed by the phenol–sulfuric acid method in microplate format according to Masuko et al. (2005). The results were expressed as percentage of carbohydrate to protein determination (% of glucose per mg of protein). The standard curve was obtained using 50–450 nM of glucose.
Hemagglutination inhibition by sugars and glycoprotein
Saccharides with a high affinity toward the lectin could block the binding site of lectin from red blood cell glycoproteins/glycolipids, inhibiting hemagglutination. The sugar inhibition assay was performed in a manner analogous to the hemagglutination test. Serial twofold dilutions of 25 μL of various sugar (20 mM) or glycoprotein (1.0%) samples were prepared in PBS in a 96-well U-shaped microtiter plate. Afterward, 25 μL of lectin sample with 2048 hemagglutination units was added to the solution mixture. The plate was incubated in room temperature for 40 min followed by the addition of 50 μL of 2.5% erythrocytes rabbit suspension to each well. The number of wells which showed hemagglutination was counted and compared with the control in which PBS was added instead of sugar or glycoprotein solution. Sugars tested included rhamnose, arabinose, fructose, glucose, sucrose, galactose, and raffinose, and glycoproteins were lactoferrin, azoalbumin, azocasein, lysozyme, and albumin. The residual activity was determined under the standard assay conditions and expressed as percentage relative activity compared with control samples.
Effect of ion on hemagglutinating activity
The purified lectin was pre-incubated at room temperature for 30 min with 10 mM of various ions as ZnSO4, ZnCl2, FeCl3, FeCl2, MnSO4, CaCl2, CuSO4, MgSO4, Na2S2O3, and K2S2O5. Then, 2.5% erythrocytes rabbit systems (v/v) were added, and the samples were incubated in a water bath at 37 °C, for 120 min. After incubation, hemagglutinating activity was assayed as described previously.
Effect of pH and temperature on the hemagglutinating activity
The influence of pH on hemagglutinating activity was determined by incubating purified lectin at 25 °C for 30 min in 10 mM buffers of different pH: citrate phosphate (pH 4.0–7.5) and Tris-HCl (pH 7.0–9.0), glycine-NaOH (pH 10.0). To determine pH stability, the purified lectin (50 μL) was incubated with buffers (50 μL) at 25 °C at various pH and then samples were neutralized, and activity was assayed after 30, 60, and 90 min to elucidate the pH stability of lectin at varied pH. The residual activity was determined under the standard assay conditions.
To determine the optimum temperature, hemagglutinating activity was measured at temperatures ranging from 20 to 100 °C. The thermal stability was examined with pre-incubation of purified lectin at determined temperatures after 30, 60, and 90 min of incubation and assayed for hemagglutination under standard assay conditions. Hemagglutination activity at any given temperature was expressed as percentage relative activity compared with control samples.
Results
Tetradesmus obliquus extract agglutinated rabbit erythrocytes obtained titer higher than 224 (Table 1), and it was used for purification steps. A lectin from T. obliquus was purified by two chromatographic steps, namely ion exchange chromatography with a DEAE-Sephadex column and gel filtration with Superdex G-75, which revealed strong agglutination activity (Table 1). The first purification step was ammonium sulfate precipitation. The crude extract obtained from T. obliquus was subjected to precipitation in ammonium sulfate in two fractions of saturation, 0–60% and 60–80%, in order to determine which precipitated fraction has the highest hemagglutinating activity. The 60–80% saturation fraction had high hemagglutinating activity (Table 1) and was selected for purification by chromatography. However, the 0–60% fraction also had significant protein concentration with low hemagglutinating activity.
The precipitate obtained by 60–80% ammonium sulfate saturation was desalted by dialysis and then applied to an anion-exchange chromatography column. The precipitate gave a single active peak in ion exchange chromatography on DEAE Sephadex (Fig. 1) with a titer of 40,960 and specific activity of 49.3 titer g−1. The active peak was further separated into two peaks in molecular exclusion chromatography with a Sephadex G-50 column. The first peak (fraction I) exhibited strong hemagglutination activity (47.6 titer g−1) and molecular mass estimated at 78 kDa, whereas the second peak (fraction II) showed weak activity (Fig. 2a). Only the first peak was reloaded in the same conditions to observe the purity protein. As shown in Fig. 2b, only one peak was observed, showing that the lectin was purified with purification factor of 4.2-fold and overall yield of 3.13%. A summary of the lectin purification process is shown in Table 1. In addition, carbohydrate analysis using the phenol–sulfuric acid assay revealed that lectin was a glycoprotein with carbohydrate content of 5.37%.
Hemagglutination activity of purified lectin was tested with human A1, A2, B, AB, and O-type erythrocytes. Table 2 summarizes the results of the hemagglutination titers. Among the erythrocytes analyzed (human blood group A1, A2, B, AB, and O), lectin from T. obliquus exhibited a preference only for human blood group O and rabbit erythrocytes and no hemagglutinating activity was observed with bovine and horse erythrocytes.
Hemagglutination activity of purified T. obliquus lectin was not inhibited by any of the monosaccharides, sucrose disaccharide, lysozyme, and lactoferrin glycoprotein, whereas it was inhibited by azoalbumin, azocasein, and albumin glycoproteins (Table 3). Tetradesmus obliquus lectin showed optimal activity at pH 7.0–7.5 and temperature between 20 and 50 °C (Figs. 3 and 4). Thermal stability was determined by incubating lectin at 25–100 °C in a water bath for 0–90 min. The lectin was not affected by exposure to a temperature of 50 °C for 90 min but declined rapidly, reaching 6.25% of the control value, when heated at 60 °C for 30 min. The hemagglutinating activity was totally abolished when the lectin was heated at 70 °C for 30 min. Lectin exhibited thermostability and retained 100% activity at 50 °C even after 60 min, and maintained activity of 50% of the control value for 90 min, while activity lectin has been shown to be lost at temperatures higher than 70 °C.
The presence of ions affected hemagglutination activity caused by lectin from T. obliquus. All evaluated ions reduced hemagglutinating activity by more than 90%, and the strongest inhibition was with CaCl2 which completely inhibited hemagglutinating activity (Table 4).
Discussion
Hemagglutination activity from T. obliquus extract was higher than that of other microalgal extracts. Among eighteen species of Chlorella, sixteen species of Chlamydomonas, three species of Spirulina, two species of Scenedesmus, and one species each of Synechococcus, Selenastrum, Monoraphidium, Coelastrum, and Eutetramorus, Scenedesmus species obtained high value of 28 using 20% ethanol and acetone (1:10, w/v) to extract preparation (Chu et al. 2004). This difference of titer may be due to the solvent and/or method of extraction used to obtain the extract with hemagglutinating activity. Singh and Walia (2018) related that Scenedesmus quadricauda, Chlorella vulgaris, Chlorococcum infusiformis, Desmodesmus subspicatus, and Desmodesmus dimorphus have cell surface–bound lectin activity. Thus, extraction using magnetic stirring of the Tris-HCl buffer pH 7.0 may have provided high values of hemagglutinating activity in T. obliquus extracts.
In addition, high hemagglutinating activity in the T. obliquus extract can be too due to the presence of polyphenols which causes false hemagglutination activity. Previous studies have shown that Scenedesmus spp. contain high concentrations of polyphenols which mimic lectin-mediated hemagglutination (Shetty and Sibi 2015; Strejckova et al. 2016; Hamed et al. 2017). In this context, the purification of protein with hemagglutinating activity becomes necessary to indicate the presence of lectin in the T. obliquus extracts.
Isolation of lectins can be achieved by a combination of different purification steps. Acids (e.g., acetic acid; Naeem et al. 2007), organic solvent (e.g., acetone; Medeiros et al. 2010) or salt (e.g., ammonium sulfate) can be used to precipitate lectins. Precipitation with ammonium sulfate at the 60–70% concentration is the most widely used. For example, Raja et al. (2011) used 60% to precipitate Aegle marmelos fruit lectin, Abreu et al. (2018) used 70% to precipitate Solieria filiformis lectin (SfL), and Yi et al. (2003) used 75% to precipitate Chlorella pyrenoidosa lectin. This is in accordance with the present work that had higher hemagglutinating activity in the 60–80% fraction (Table 1). On the other hand, lower fractions of ammonium sulfate (0–60%) can also precipitate protein with weak hemagglutinating activity (Table 1), as observed for the lectin from the cyanobacterium Oscillatoria agardhii that was precipitated with 20–60% of ammonium sulfate (Sato et al. 2000).
Lectin from T. obliquus was purified using anion-exchange DEAE-Sephadex and gel filtration chromatography. Other microalgal lectins have been purified using conventional chromatographic approaches (Sato et al. 2000; Melo et al. 2004). The majority of reports on lectin activity from other microalgae and cyanobacteria used a multistep purification protocol. Two-step purification of the lectin demonstrates the efficiency of the procedure.
Most of the lectins isolated from algae, microalgae, and cyanobacteria are low molecular weight proteins, with values among 3.5 to 57 kDa. Therefore, the native molecular mass estimated for T. obliquus lectin by gel filtration of 78 kDa was considered high when compared with lectins isolated from most species of microalgae such as the green algae Spirogyra spp. (56 kDa; Oliveira et al. 2017) and Chlorella pyrenoidosa (15 kDa; Yi et al. 2003) and the cyanobacteria Microcystis aeruginosa (57 kDa; Yamaguchi et al. 1998), Microcystis viridis (12.2 kDa; Yamaguchi et al. 1999), Nostoc (11 kDa); Oscillatoria agardhii (13 kDa; Sato et al. 2000), and Scytonema varium (9.7 kDa; Bokesch et al. 2003).
Tetradesmus obliquus lectin is a glycoprotein with sugar contents (5.37%) lower than other isolated from some other algae and cyanobacteria, e.g., Oscillatoria agardhii (16%; Sato et al. 2000), Tichocarpus crinitus (6.9%; Molchanova et al. 2009), and Eucheuma sp. (8–10%; Kawakubo et al. 1999), and higher than Meristiella echinocarpa lectin (2.0%; Chaves et al. 2018), Gracilaria ornata lectin (2.9%; Leite et al. 2005), Ulva pertusa lectin (1.2%; Wang et al. 2004).
Lectin from T. obliquus was specific for human O-type erythrocytes. Human blood O type possesses a lower amount of sialic acids when compared with other human blood types (Bulai et al. 2003), and rabbit erythrocytes have previously been shown to have a low content of sialic acid on the surface when compared with horse and bovine erythrocytes (Ish et al. 1993, Angel and Burness 1977; Munksgaard et al. 2014). So, lectin from T. obliquus recognizes sialic acid–poor erythrocytes. Similar results have been observed by hemagglutinins from the Chlorophyceae Ankistrodesmus sp. (titer = 225) and Chlorella ellipsoidea (titer = 23) (Liao and Huang 2000). Chu et al. (2004) reported that Scenedesmus quadricauda and Scenedesmus ecornis extract agglutinated O more strongly that A or B human blood type and lectin from C. pyrenoidosa agglutinated erythrocytes from rabbit, sheep, and pigeon but not from duck, chicken, and human A, B, or O blood cell groups with the highest activity in rabbit erythrocytes (Hung et al. 2012). This study is consistent with other reports on the activity of microalgae hemagglutinins in O human and rabbit erythrocytes (Liao and Huang 2000; Chu et al. 2004; Hung et al. 2012).
Lectin from T. obliquus did not agglutinate subtypes A1 or A2 erythrocytes. This can be justified by the presence of some glycoproteins present on the surface of erythrocytes that promote inhibition of the binding of lectin to carbohydrates on the surface of the blood cell. In the literature, few lectins differentiate subgroups A1 and A2. Dolichos biflorus lectin is the best known in routine blood group serology by recognized only A1 erythrocytes. This lectin is widely used in blood banks for subgrouping. Lectin from Vicia cracca, Phaseolus limensi, and Crotalaria striata has also been shown to be potent in anti-A activity (Renkonen 1948; Khang et al. 1990; Khan et al. 2002).
Only azoalbumin, azocasein, and albumin glycoproteins inhibited hemagglutination activity of purified T. obliquus lectin. This suggests that T. obliquus lectin probably has complex carbohydrate specificity. These properties are also characteristic of many marine macroalgal lectins, such as the Chlorophyta (Hung et al. 2012) and of lectin from the cyanobacterium Oscillatoria (Sato et al. 2000).
Lectin purified from T. obliquus showed optimal activity at pH 7.0–7.5 and was stable after 90 min, losing activity outside of this range. The change in ionization state of a molecule affected by the pH determines the binding forces between the lectin and erythrocyte membrane, leading to a loss of activity. Extremes of pH can alter the lectin native structure due to the changes in the ionization state of amino acid residues, which can lead to the denaturation of the lectin. Previously, a lectin from the red algas Acrocystis nana and Tichocarpus crinitus has also been reported to retain its maximum hemagglutinating activity within a pH range of 6.0–7.0 and 7.0–8.0, respectively (Molchanova et al. 2009; Anam et al. 2017).
Tetradesmus obliquus lectin showed optimal temperature of 20–50 °C and was stable at 50 °C for 90 min. Similar results were observed in lectins with high molecular mass such as red marine alga Vidalia obtusiloba lectin (Melo et al. 2004). On the other hand, Chlorella pyrenoidosa lectin (14–15 kDa) was tolerant of high temperature and its activity could be maintained even when the lectin solution was heated to 90 °C for 10 min (Yi et al. 2003). Normally, lectins with low molecular mass are more thermostable due to the three-dimensional structure of the protein and the presence of cysteines forming disulfide bonds (Nagano et al. 2002; Nascimento et al. 2006).
The addition of ions influenced hemagglutinating activity. As seen in many algal lectins, most of the lectins are non-dependent on divalent cations (Alvarez-Hernández et al. 1999; Chaves et al. 2018), for example, the lectins from the algae Amansia multifida (Costa et al. 1999), Bryothamnion triquetrum (Calvete et al. 2000), Hypnea musciformis (Nagano et al. 2002), Tichocarpus crinitus (Molchanova et al. 2009), and Codium giraffa (Alvarez-Hernández et al. 1999). On the other hand, the hemagglutinating activities of the red marine algae Vidalia obtusiloba (Melo et al. 2004) and Pterocladiella capillacea (Oliveira et al. 2002) were totally restored by the addition of the divalent cations (10 mM CaCl2, MgCl2). In contrast to the great majority of algal lectins, hemagglutinating activity exhibited by the purified lectin of T. obliquus was inhibited by ions and the most potent inhibitory substance was CaCl2 (10 mM), unlike many agglutinins (lectin) from other sources. This suggests that T. obliquus may provide a valuable source for unique agglutinins.
In conclusion, we report purification and characterization of a novel type of lectin isolated from the alga T. obliquus that is easily mass-cultured in the laboratory, making it easy to supply the useful lectin. It has unique characteristics as high molecular weight, inhibition by different ions, specificity for poor-sialic acid erythrocytes, and complex carbohydrate, and does not agglutinate bovine, horse, and human A, B, and AB erythrocytes. Thus, this lectin could be considered as an alternative for applications in several areas of biotechnology.
References
Abreu TM, Monteiro VS, Martins ABS, Teles FB, Rivanor RLC, Mota EF, Macedo DS, Vasconcelos SMM, Júnio JERH, Benedives NMB (2018) Involvement of the dopaminergic systemin the antidepressant-like effect of the lectin isolated from the red marine alga Solieria filiformis in mice. Int J Biol Macromol 111:534–541
Alvarez-Hernández S, De Lara-Isassi G, Arreguín-Espinoza R, Arreguín B, Hernández-Santoyo A, Rodríguez-Romero A (1999) Isolation and partial characterization of giraffine, a lectin from the Mexican endemic alga Codium giraffa Silva. Bot Mar 42:573–580
Anam C, Praseptiangga D, Nugraheni MA, Nurhayati T, Fajarningsih ND, Zilda DS, Chasanah E, Yunus A (2017) Preliminary characterization of crude lectin fraction of the red alga, Acrocystis nana from Wediombo Beach of the southern coast of Java Island, Gunung Kidul, Yogyakarta, Indonesia. IOP Conf Ser Mater Sci Eng 193: 012016
Angel MA, Burness ATH (1977) The attachment of encephalomyocarditis virus to erythrocytes from several animal species. Virology 83:228–232
Bokesch HR, O’Keefe BR, McKee TC, Pannell LK, Patterson GM, Gardella RS, Sowder RC 2nd, Turpin J, Watson K, Buckheit RW Jr, Boyd MR (2003) A potent novel anti-HIV protein from the cultured cyanobacterium Scytonema varium. Biochemistry 42:2578–2584
Bulai T, Bratosin D, Pons A, Montreuil J, Zanetta JP (2003) Diversity of the human erythrocyte membrane sialic acids in relation with blood groups. FEBS Lett 534:185–189
Calvete JJ, Costa FHF, Saker-Sampio S, Murciano MPM, Nagano CS, Cavada BS, Grangeiro TB, Ramos MV, Jr CB, Silveira SB, Freitas BT, Sampio AH (2000) The amino acid sequence of the agglutinin isolated from the red marine algae Bryothamnion triquetrum defines a novel lectin structure. Cell Mol Life Sci 57:343–350
Chaves RP, Silva SR, Silva JPF, Carneiro RF, Sousa BL, Abreu JO, Carvalho FCT, Rocha CRC, Farias WR, Sousa OV, Silva ALC, Sampaio AH, Nagano CS (2018) Meristiella echinocarpa lectin (MEL): a new member of the OAAH-lectin family. J Appl Phycol 30:2629–2638
Chu CY, Liao WR, Huang R, Lin LP (2004) Haemagglutinating and antibiotic activities of freshwater microalga. World J Microbiol Biotechnol 20:817–825
Chu CY, Huang R, Lin LP (2006) Purification and characterization of a novel haemagglutinin from Chlorella pyrenoidosa. J Ind Microbiol Biotechnol 33:967–973
Coelho LCBB, Silva PMS, Lima VLM, Pontual EV, Paiva PMG, Napoleão TH, Correia MTS (2017) Lectins, interconnecting proteins with biotechnological/pharmacological and therapeutic applications. Evid Based Complement Alternat Med 1594074:1–22
Correa MTS, Coelho LCBB (1995) Purification of glucose/mannose specific lectin, isoform 1, from seeds of Cratylia mollis (camaratu bean). Appl Biochem Biotechnol 55:261–273
Costa FHF, Sampaio AH, Neves SA, Rocha MLA, Benevides NMB, Freitas ALP (1999) Purification and characterization of a lectin from the red marine alga Amansia multifida. Physiol Mol Biol Plants 5:53–61
Hamed SM, Samy S, Klöck G, AbdElgawade H (2017) Sensitivity of two green microalgae to copper stress: growth, oxidative and antioxidants analyses. Ecotoxicol Environ Saf 144:19–25
Hung LD, Ly BM, Trang VTD, Ngoc NTD, Hoa LT, Trinh PTH (2012) A new screening for hemagglutinins from Vietnamese marine macroalgae. J Appl Phycol 24:227–235
Ish C, Ong GL, Desai N, Mattes MJ (1993) The specificity of alternative complement pathway-mediated lysis of erythrocytes: a survey of complement and target cells from 25 species. Scand J Immunol 38:113–122
Ishaq AG, Matias-Peralta HM, Basri H (2016) Bioactive compounds from green microalga – Scenedesmus and its potential applications: a brief review. Pertanika J Trop Agric Sci 39:1–16
Kawakubo A, Makino H, Onhishi J, Hirohara H, Hori K (1999) Ocurrence of highly yielded lectins homologous within the genus Eucheuma. J Appl Phycol 11:149–156
Khan F, Khan RH, Sherwani A, Mohmood S, Azfer MA (2002) Lectins as markers for blood grouping. Med Sci Monit 8:RA293–RA300
Khang NQ, JeanLuc G, Hoebeke J (1990) Blood group A specific lectin from the seeds of Crotolaria striata. Biochim Biophys Acta 1033:201–2013
Leite YFMM, Silva LMCM, Amorim RC das N, Freire EA, de Melo JDM, Grangeiro TB, Benevides NMB (2005) Purification of a lectin from the marine red alga Gracilaria ornata and its effect on the development of the cowpea weevil Callosobruchus maculatus (Coleoptera: Bruchidae). Biochim Biophys Acta, Gen Subj 1724:137–145
Liao WR, Huang R (2000) Agglutination of human and animal erythrocytes in marine unicellular algae. J Ind Microbiol Biotechnol 24:262–266
Masuko T, Minami A, Iwasaki N, Majima T, Nishimura SI, Lee YC (2005) Carbohydrate analysis by a phenol–sulfuric acid method in microplate format. Anal Biochem 339:69–72
Medeiros DS, Medeiros TL, Ribeiro JKC, Monteiro NKV, Migliolo L, Uchoa AF, Vasconcelos IM, Oliveira AS, de Sales MP, Santos EA (2010) A lactose specific lectin from the sponge Cinachyrella apion: purification, characterization, N-terminal sequences alignment and agglutinating activity on Leishmania promastigotes. Comp Biochem Physiol B 155:211–216
Melo F, Benevides NMB, Pereira MG, Holanda ML, Mendes FNP, Oliveira SRM, Freitas ALP, Silva LMCM (2004) Purification and partial characterization of a lectin from the red marine alga Vidalia obtusiloba C. Agardh. Braz J Bot 27:263–269
Molchanova V, Chernikov O, Chikalovets I, Lukyanov P (2009) Purification and partial characterization of the lectin from the marine alga Tichocarpus crinitus (Gmelin) Rupr (Rhodophyta). Bot Mar 53:69–78
Munksgaard PS, Skals M, Reinholdt J, Poulsen K, Jensen MR, Yang C, Leipziger J, Vorup-Jenses T, Praetoriu HA (2014) Sialic acid residues are essential for cell lysis mediated by leukotoxin from Aggregatibacter actinomycetemcomitans. Infect Immun 82:2219–2228
Naeem A, Ahmad E, Khan RH (2007) An alternate high yielding purification method for Clitoria ternatea lectin. Int J Biol Macromol 41:481–486
Nagano CS, Moreno FBMB, Bloch C Jr, Prates MV, Calvete JJ, Saker-Sampaio S, Farias WRL, Tavares TD, Nascimento KS, Grangeiro TB, Cavada BS, Sampaio AH (2002) Purification and characterization of a new lectin from the red marine alga Hypnea musciformis. Protein Pept Lett 9:159–165
Nascimento KS, Nagano CS, Nunes EV, Rodrigues GV, Calvete JJ, Saker S, Sampaio WRL, Farias SAH (2006) Isolation and characterization of a new agglutinin from red marine alga Hypnea cervicornis J Agardh. Biochem Cell Biol 84:49–54
Oliveira AS, Lóssio CF, Rangel AJ, Martins MGQ, Nascimento FEP, Andrade MLL, Cavada BS, Lacerda SR, Nascimento KS (2017) Detection, purification and characterization of a lectin from freshwater green algae Spirogyra spp. Ann Acad Bras Cienc 89:2113–2117
Oliveira SRM, Nascimento AE, Lima MEP, Leite YFMM, Benevides NMB (2002) Purification and characterisation of a lectin from the red marine alga Pterocladiella capillacea (S.G. Gmel.) Santel. & Hommers. Revista Brasil Bot 25:397–403
Raja SB, Murali MR, Kumar NK, Devaraj SN (2011) Isolation and partial pharacterisation of a novel lectin from Aegle marmelos fruit and its effect on adherence and invasion of Shigellae to HT29 cells. PLoS One 6:1–9
Renkonen KO (1948) Studies on haemagglutinins present in seeds of some representatives of leguminoseae. Ann Med Exp Biol Fenn 26:66–72
Sato Y, Murakami M, Miyazawa K, Hori K (2000) Purification and characterization of a novel lectin from a freshwater cyanobacterium, Oscillatoria agardhii. Comp Biochem Physiol B 125:169–177
Shetty V, Sibi G (2015) Relationship between total phenolics pontent and antioxidant activities of microalgae under autotrophic, heterotrophic and mixotrophic growth. J Food Res 4:1–9
Singh RS, Walia AK (2018) Lectins from red algae and their biomedical potential. J Appl Phycol 30:1833–1858
Smith PK, Krohn RI, Hermanson GT, Mallia AK, Gartner FH, Provenzano M, Klenk DC (1985) Measurement of protein using bicinchoninic acid. Anal Biochem 150:76–85
Strejckova A, Dorak M, Hynstova V, Hedbavny J, Ridoskova A, Klejdus B, Huska D (2016) Effect of copper on secondary metabolism of microalgae Scenedesmus quadricauda. MendelNet 23:1016–1021
Wang S, Zhong FD, Zhang YJ, Wu ZJ, Lin QY, Xie LH (2004) Molecular characterization of a new lectin from the marine alga Ulva pertusa. Acta Biochim Biophys Sin 36:111–117.
Wynne MJ, Hallan JK (2016) Reinstatement of Tetradesmus G. M. Smith (Sphaeropleales, Chlorophyta). Feddes Repertorium 126:83–86
Yamaguchi M, Jimbo M, Sakai R, Muramoto K, Kamiya H (1998) Purification and characterization of Microcystis aeruginosa (freshwater cyanobacterium) lectin. Comp Biochem Physiol B 119:593–597
Yamaguchi M, Ogawa T, Muramoto K, Kamio Y, Jimbo M, Kamiya H (1999) Isolation and characterization of a mannan-binding lectin from the freshwater cyanobacterium (blue-green algae) Microcystis viridis. Biochem Biophys Res Commun 265:703–708
Yi Z, Ping Y, Yanru L (2003) Purification and partial characterization of Chlorella pyrenoidosa lectin. Acta Hydrobiol Sin 27:36–40
Acknowledgments
The authors gratefully acknowledge Fundação de Amparo à Ciência e Tecnologia do Estado de Pernambuco (FACEPE) and Conselho Nacional de Desenvolvimento Científico e Tecnológico (CNPq).
Funding
This study was financed in part by Coordenação de Aperfeiçoamento de Pessoal de Nível Superior (CAPES) – Finance Code 001.
Author information
Authors and Affiliations
Corresponding author
Ethics declarations
Conflict of interest
The authors declare that they have no conflict of interest.
Additional information
Publisher’s note
Springer Nature remains neutral with regard to jurisdictional claims in published maps and institutional affiliations.
Rights and permissions
About this article
Cite this article
Silva, A.J., Cavalcanti, V.L.R., Porto, A.L.F. et al. The green microalgae Tetradesmus obliquus (Scenedesmus acutus) as lectin source in the recognition of ABO blood type: purification and characterization. J Appl Phycol 32, 103–110 (2020). https://doi.org/10.1007/s10811-019-01923-5
Received:
Revised:
Accepted:
Published:
Issue Date:
DOI: https://doi.org/10.1007/s10811-019-01923-5