Abstract
Sediment flushing from dams can help desilting reservoirs and reinstate the longitudinal sediment transfer continuity in rivers, but negative ecological impacts might arise. We monitored the ecological effects of a flushing event conducted from 27th May to 14th June 2019 in the Rienz River, in South Tyrol (NE Italy). Using a multi-habitat scheme approach, we collected macroinvertebrates 10 days before, and then 40 and 74 days after the completion of the operations. We selected seven biological traits—organism size, life cycle duration, mobility (dispersal and locomotion), feeding type, substrate and current velocity preference—to characterize and compare the invertebrate communities before and after the sediment pulse disturbance. Turbidity was recorded continuously for the entire duration of the event. Results indicate that invertebrate assemblages exhibited a general decrease in taxonomic richness and Shannon diversity 40 days after the event, but density and richness recovered over time. Shifts in species composition were observed in post flushing samples, with a reduction in density of sensitive species (Heptageniidae) and shredders. Post-flushing samples were generally characterized by sediment-tolerant taxa, able to cope with the new habitat conditions. Altered taxonomic and functional community composition following the flushing prevented the full functional recovery to pre-disturbance conditions.
Similar content being viewed by others
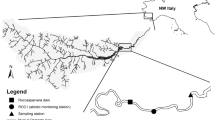
Explore related subjects
Discover the latest articles, news and stories from top researchers in related subjects.Avoid common mistakes on your manuscript.
Introduction
In Alpine rivers, hydropower production is a crucial energy source (Fette et al., 2007). However, hydroelectric power plants, and associated reservoirs, can profoundly modify rivers hydro-morphology (Meile et al., 2011; Bruno & Siviglia, 2012; Arheimer et al., 2017) and biodiversity (Vörösmarty et al., 2010; Reid et al., 2019). The most common type of hydroelectric facilities in Alpine regions are storage plants, which tend to accumulate considerable amounts of sediment from upstream soil erosion, and consequently, lose storage volume. Worldwide annual loss of reservoir storage capacity due to sedimentation is estimated between 0.5 and 1% (Trimble et al., 2012). Sediment trapping in reservoirs has dramatic consequences downstream, including channel bed erosion, bed armouring, and coastal erosion. For this reason, the longitudinal continuity of sediment transport is considered a key ecological issue, and several management actions can be implemented to restore it (Wohl et al., 2015).
In order to preserve reservoir storage capacity, sediment management techniques are used either in the reservoir or at the dam (e.g., sediment sluicing, flushing or venting) in order to minimize deposition or remove accumulated sediments (Kondolf et al., 2014). Flushing is one of the most common management strategies to desilt small-medium size reservoirs (Espa et al., 2016). Flushing involves resuspending deposited fine sediment and transporting it downstream through bottom outlets of the dam by releasing water and lowering the water level in the reservoir (Kondolf et al., 2014). The transfer of sediments accumulated behind dams can restore the longitudinal sediment transport and limit streambed incision and bed armouring downstream (Petts & Gurnell, 2005) while favouring the formation of vital hydrogeomorphic patches (sensu Thorp et al., 2006). However, flushing could be detrimental for river biota if water discharge and fine sediment transport is excessive and/or poorly timed (Kjelland et al., 2015; Bona et al., 2016; Espa et al., 2016; Grimardias et al., 2017) relative to the natural hydrological and sediment regimes.
Technical recommendation and threshold limits on suspended sediment concentrations are normally set by local authorities and environmental agencies, but are often based on simple concentration–time–response models simulating the impacts on fish populations (Newcombe & Jensen, 1996). However, legislation differs among countries, and the lack of reference case studies and of appropriate eco-hydraulic models hampers the accurate predictions of ecological effects and subsequent recovery. Specific impacts of sediment flushing on riverine biotic components other than fish (e.g., macroinvertebrates) have received less attention despite potentially drastic and long-lasting consequences (Gomi et al., 2010; Doretto et al., 2019). In particular, the impacts on macroinvertebrate communities can be severe: these include direct effects such as abrasion (McKenzie et al., 2020), induction of drift (Larsen & Ormerod, 2010; Bruno et al., 2016; Béjar et al., 2017), changes in community structure and function (Larsen et al., 2011), as well as indirect effects associated with substrate clogging (Bo et al., 2007) and declined availability of good-quality trophic resources (Doretto et al., 2016).
Responses of freshwater biota to anthropogenic impairment have been widely used to quantify ecosystems’ deviations from structural and functional integrity (Dolédec & Statzner, 2010). The recent use of functional approaches in bio-assessment is particularly useful to identify biological traits sensitive to specific impacts, and to permit comparison of functional responses among different eco-regions, because traits are independent of taxonomy (Dolédec & Statzner, 2010). Biological traits are well-defined functional attributes of the species related to morphological, physiological, behavioural and ecological characteristics that strongly influence organismal performance (McGill et al., 2006). Community metrics describing species life history traits are relevant for ecosystem functioning (Baird et al., 2008) and provide the mechanistic basis of ecological responses to stress (Dolédec & Statzner, 2008). Because anthropogenic disturbances select species possessing relevant adaptive traits, characteristic trait patterns can be used as indicators of the source of impairment (Statzner et al., 2004). For instance, traits conferring resistance and resilience (Van Looy et al., 2019) to fine sediment transport and deposition are associated with polivoltinism, short life cycles, and r-selected strategy (Buendia et al., 2013). The application of functional traits in bioassessment has well recognized advantages over taxonomic approaches (Dolédec & Statzner, 2010; Verberk et al., 2013; Enquist et al., 2015), and has been widely used to assess the effects of fine sediment in streams in other contexts (Larsen et al., 2011; Buendia et al., 2013; Doretto et al., 2016, 2018). Therefore, monitoring the ecological effects of desilting operations combining structural and functional approaches can provide key knowledge advancement to guide proper management schemes.
In the present study, we explored the effects of a 19-day sediment flushing from an Alpine reservoir dam in South Tyrol (NE Italy) on the structural and functional composition of aquatic macroinvertebrate communities. We used a “before-after” design and a combined approach of traditional taxonomic metrics and selected biological traits to examine the response of macroinvertebrates 40 and 74 days after the event over four sites. Specifically, we expected the flushing to: (i) cause a rapid decline in invertebrate diversity, with effects more pronounced in the upstream sites closer to the dam; (ii) cause an homogenisation of the macroinvertebrate taxonomic assemblages, due to the sudden increase in longitudinal connectivity among sites after the flushing, predicted by a “mass effect” process (sensu Leibold et al., 2004); (iii) affect sediment and flow sensitive taxa with low resistance to flow and sediment scouring, and favour resistant (euriecious) and pioneer taxa able to survive/rapidly recolonise the reaches after the disturbance, thus changing the functional composition of the macroinvertebrate assemblages.
Materials and methods
Study area
The Rio di Pusteria dam, completed in 1940, is located at 704 m asl on the Rienz-Rienza River (drainage area of ~ 1,900 km2), a left tributary of the Eisack-Isarco River. The two rivers join at the city of Brixen-Bressanone (South Tyrol, Northern Italy, Fig. 1) at an altitude of 565 m asl, ca. 84 km downstream of the Rienz River springs. The Rienz River is characterized by a nivo-glacio-pluvial regime, with snowmelt contribution in spring, and glacier melt flow—originating from the important Ahr-Aurino river sub-basin—in summer. Long-lasting, frontal precipitation events, and related floods, typically occur in late summer and early autumn. Average annual rainfall measured at Vandoies (~ 3 km upstream the reservoir) amounted to ~ 690 mm for the period 1989–2016. During the period 1985–2019, the mean monthly discharge of the Rienz River measured at Vandoies gauging station (managed by the Hydrographic Office) was 44.2 m3 s−1, with values ranging between 18.5 (February) and 91.4 (June) m3 s−1.
The Rio di Pusteria reservoir is created by a 25-m high reinforced concrete dam on the Rienz River. From the reservoir, up to 50.9 m3 s−1 are delivered in a penstock—which also receives water from a second reservoir in the upper Eisack River -, to the hydropower plant of Brixen, located ~ 12 km downstream the dam. Brixen hydropower plant (124 MW total installed capacity) is one of the largest of South Tyrol, producing 9% of the hydropower energy of the entire South Tyrol. The Rio di Pusteria reservoir has a storage capacity of 1.36 × 106 m3. To our knowledge, sediment flushing of the reservoir has been carried out since 2001, every 2–3 years on average. Main bottom outlets consist of three square sluice gates (4.5 m × 4.5 m) which let water and sediment flow from the bottom of the dam (904 m3 s−1 overall for the three gates), while excess water can also overflow through two spillways located at the top of the dam (425 m3 s−1 overall) and from a lateral auxiliary bottom outlet (500 m3 s−1). Because of the relatively small reservoir storage capacity, occasional overspills might occur due to high sedimentation rates between two consecutive flushing events or in case of heavy and prolonged rainfall. The residual flow released by the Rio di Pusteria dam is constant and amounts to 5.35 m3 s−1. The downstream by-passed reach of the Rienz River—extending to Brixen power plant—receives only minor flow contributions of tributaries, and thus the flow regime is severely altered for its whole length.
The 2019 flushing operations
In 2019, the sediment flushing from the Rio di Pusteria reservoir lasted for 19 consecutive days, from 27th May to 14th June 2019. Clean water was initially released from the spillways (the reservoir was completely full) and successively from the bottom outlets before the proper sediment flushing started. Outflows during the operations were estimated applying mass balance equations using the reservoir water levels at the dam (provided by the hydropower company), the reservoir bathymetry before the flushing event, and the inlet flow rate from the Rienz River (Vandoies gauging station). Details on the legal restrictions, concentration limits during the operations, starting and ending procedures of the flushing are provided in Online Resource 1.
The volume of sediment mobilized from the reservoir through the flushing operations was estimated by comparing two Digital Elevation Models (DEM) acquired before and after the flushing (DTM resolution: 0.5 m, surface area: 271,665 m2; resolution of the topographic survey: 0.020 m, root-mean-square error of the difference: 0.028 m). The computed volume of sediments removed from the reservoir during the flushing was estimated to be about 580,000 m3 (~ 265,000 t), corresponding to a flushing efficiency of 0.4%, calculated as the ratio between the volume of evacuated sediment and the volume of water used (Morris & Fan, 1988).
Monitoring activities
Turbidity and suspended sediment concentration
Turbidity was monitored at 5-min intervals for the entire duration of the flushing event using two immersion probes, installed 10 days before. A Solitax ts-line turbidimeter (Hach Lange, accuracy: ± 0.001 NTU for turbidity up to 1,000 NTU, range up to 4,000 NTU) was installed ~ 350 m downstream from the reservoir outlet (hereafter referred to as ‘T1’, Fig. 1). A multiparametric probe including a turbidimeter (DS5 Hydrolab, accuracy: ± 5% for turbidity > 400 NTU, range up to 3,000 NTU) was installed ~ 4.5 km downstream of the Rienz-Eisack confluence to monitor the propagation of the sediment wave in the Eisack River (hereafter referred to as ‘T2’, Fig. 1). Before installation, probes were inter-calibrated in the laboratory using turbidity standard solutions at 0 and 1,000 NTU. Ten water samples per site were collected by an isokinetic sampler in the period 23 May–13 June 2019, covering a wide range of turbidity (25–1,400 NTU) and different stages of the flushing operations. The samples were analysed at the local Environmental Agency lab with the standard gravimetric method (Gray & Gartner, 2009)—based on vacuum filtration (0.45 μm filters), oven drying (105 °C) and repeated weighing—to obtain the suspended sediment concentration (SSC) in each sample. Therefore, it was possible to build SSC-NTU calibration curves and reconstruct the complete SSC dynamics for both monitored sites. The regression curves obtained by site-specific, simultaneous pairs of SSC (from the gravimetric method) and NTU (from the turbidimeters) were characterised by R2 equal to 0.95 at T1 and to 0.99 at T2.
Invertebrate sampling
We selected four sampling sites at increasing distance from the reservoir (named site1-4 thereafter, Fig. 1; Online Resource 2) based on aerial photographs, preliminary field surveys, accessibility, and location of turbidimeters. The three upstream-most sites were situated along the Rienz River, between the village of Naz-Sciaves and the town of Brixen, and the downstream-most site was located in the village of San Pietro Mezzomonte, 6 km downstream from the confluence of the Rienz and Eisack Rivers. The total distance from site1 to site4 is ~ 15 km, and site1 is ~ 3.5 km downstream of the reservoir, while site 2, 3 and 4 are ~ 7, 11.5 and 19 km distant from the dam, respectively.
We used a Surber sampler (0.23 m × 0.22 m, 100-µm mesh) to collect benthic macroinvertebrates on three sampling occasions, before (16–17 May 2019, hereafter referred to as ‘pre’), and twice after the flushing event (24 July and 27 August 2019, hereafter referred to as ‘post1’ and ‘post2’), which occurred 10 days before the beginning, and 40 and 74 days after the end of the flushing event, respectively. We adopted a multi-habitat scheme approach, designed to proportionally sample major habitats according to their occurrence within the river reach (Hering et al., 2003). At each site, we collected 10 subsamples, which were pooled into a single sample representing each study reach. Samples were preserved in 90% ethanol and returned to the laboratory for sorting and identification. Physical and chemical parameters of surface water such as temperature (°C), oxygen concentration (mg L−1), conductivity (µS m−1), pH, turbidity (NTU) were measured at each site, on each sampling occasion with a multi-parameter portable meter (Profi-Line pH/Cond 3320, WTW GmbH & Co., Weilheim, Germany). Mean flow velocity (at 60% total depth from water surface) was recorded by using a digital water velocity meter (Global Water Flow Probe, College Station, Texas, USA) and averaging velocity measurements taken at each subsample point. We identified all benthic invertebrates to the lowest possible taxonomic level usually genus or species, abundances of each taxon were expressed as the ratio of counts to total sampled surface (i.e., taxon density, expressed as N. ind m−2). In particular, we identified Ephemeroptera, Plecoptera and Trichoptera (EPT) to the genus or species level following Campaioli et al. (1994, 1999), Tachet et al. (2010), Fochetti and Tierno de Figueroa (2008), Belfiore (1983), Moretti (1983), and Waringer and Graf (2011), while most Diptera families (including Chironomidae, Empididae, Psychodidae, Simuliidae, Ceratopogonidae, Blephariceridae, Anthomyiidae, Thaumaleidae), and Oligochaeta, Copepoda, Ostracoda, Cladocera, Hydrachnidia, Collembola, Hirudinea, Tricladida were recorded as such.
Species traits
Information on functional traits was derived from literature and the online database on the ecology of freshwater organisms www.freshwaterecology.info (Schmidt-Kloiber & Hering, 2015). Seven functional traits (Table 1) were assigned to the collected taxa. Chironomidae, Oligochaeta, Hydrachnidia, Cladocera, Copepoda, Ostracoda, Collembola, Hirudinea (one individual) and Tricladida (one Planaria sp. and other two unidentified individuals) were excluded from the functional analysis due to the limited information on their functional traits either for the whole taxon, or at the undertaken classification level. The traits chosen to assess the impacts of sediment flushing on macroinvertebrate communities (see Table 1) relate to morphology (maximum potential size), life history (life span), mobility (dispersal, locomotion and ability to avoid drift), physiology (feeding types), and habitat ecological conditions (substrate preference, current velocity). Affinities of each taxon to each trait category were standardized between 0 and 1 using a fuzzy coding procedure (Chevene et al., 1994). To derive community-level trait proportions, affinities were weighted by species relative abundance using the ‘functcomp’ function from the FD package (Laliberté et al., 2014) of the R software (version 3.6.2, R Core Team, 2019).
Data analysis
Physical, chemical and hydrological characterization of the flushing
We used the R software to build curves for the reservoir water level, the outflow water discharge at the dam in the Rienz River and SSC data from T1 and T2 and to identify relative SSC peaks and mean values. We computed cross-correlation analysis (‘ccf’ function in R) to assess the relationship between the two turbidites and calculate lag-times. To test differences among sites in median values of water physical and chemical variables, we used the non-parametric Kruskal–Wallis one-way ANOVA test in R. Significance levels below 0.05 indicate that the medians of the sites differ.
Effects of flushing operations on taxonomic richness, diversity and functional composition of invertebrate communities
For each site and sampling date, we calculated the following community metrics: density (N. ind m−2), taxonomic richness (number of taxa), Shannon diversity (as effective number of species of order q = 1; Jost, 2006), and functional dispersion (FDis). FDis was chosen as a measure of functional diversity that is mathematically independent from taxa richness, and was calculated with the FD package in R (Laliberté et al., 2014). To calculate FDis, we first derived a distance matrix between taxa based on their functional traits. We used the first 8 components of a Fuzzy Correspondence Analysis (FCA; explaining 75% of trait variance) to ordinate taxa in the multivariate functional space. The score of each taxon on the FCA axes were used to construct a dissimilarity matrix (Euclidean distances) used to calculate community FDis.
To examine the effect of flushing on the taxonomic composition, we used Principal Coordinate Analysis (PCoA) based on Bray–Curtis dissimilarity of log(x + 1) invertebrate abundances using the ‘vegdist’ and ‘betadisper’ functions from the vegan (Oksanen et al., 2019) and ape (Paradis & Schliep, 2019) packages in R. Taxa present with density < 2 ind m−2 (9 taxa) were excluded from the PCoA. A one-way Analysis of Similarities (ANOSIM) was successively performed with the R ‘anosim’ function to test for differences in the invertebrate assemblages among sampling dates using a random Monte Carlo permutations test (999 permutations). Both P and R ANOSIM values were examined: R values > 0.75 indicated strong separation among groups, R = 0.75–0.25 indicated separate groups with overlapping values, and R < 0.25 as barely distinguishable groups (Clarke & Gorley, 2015). In order to assess which taxa contributed the most to the dissimilarity between pairs of sampling dates, and to calculate the similarity between groups of samples and within each group, we run a Similarity Percentage Analysis, SIMPER (Clarke, 1993), based on the log(x + 1)-transformed abundances and the resulting Bray–Curtis similarity matrix. Bray–Curtis similarity scores 100 when two samples are identical, and 0 if they have no species in common; only those taxa contributing to 70% of the total dissimilarity between pairs of samples were taken into account, thus rare taxa were ignored. SIMPER was run using the software PRIMER version 7 (Clarke & Gorley, 2015).
We used the Fuzzy Correspondence Analysis (FCA) (Chevene et al., 1994) based on the community-level trait proportions to assess and visualise changes in the functional composition of communities. FCA was performed with the ade4 R package (Chevene et al., 1994). The loadings of the trait categories over the FCA axes were used to guide interpretations of functional responses to flushing, and to select traits which were further analysed with univariate tests (see below) to formally quantify their response. Specifically, we selected trait modalities showing high correlation (> |0.6|) with the first two FCA components (see Table 3).
To quantify the effects of flushing on individual community metrics and selected trait categories, we used Linear Mixed-Effect Models (LME) using the nlme R package (Bates et al., 2015), including time as fixed effect (i.e.,, ‘pre’, ‘post1’, ‘post2’) and site as random intercept component (×4) to account for inherent differences among locations. Traits were expressed as proportions and were logit-transformed before univariate tests, as recommended (Warton & Hui, 2011). LME tests on diversity metrics and traits modalities were not corrected for multiple testing given the limited sample size available (N = 12).
Results
Physical, chemical and hydrological characterization of the flushing
The time series recorded during the flushing of the reservoir water level, the outflow water discharge at the dam in the Rienz River, and the SSC in Rienz and Eisack rivers are shown in Fig. 2, while the main results of the physical monitoring are provided in Online Resource 4.
a Water level (m asl) in the Rio di Pusteria reservoir. b Suspended sediment concentration (SSC, mg l-1) measured in the Rienz River (black line) ~ 350 m downstream the dam and in the Eisack River (grey line) ~ 4.5 km downstream the confluence with the Rienz River. c Outflow discharge at the dam outlet (m3 s−1) in the Rienz River during the flushing. Arrows indicate the start and end of the flushing
Mean outflow discharge as measured at the dam outlet during the first 4 h of the flushing was 39 ± 3 m3 s−1. Before the start of the flushing, excess water overflowed from the spillways and electricity was still generated. Once flushing operations started, less and less water was used for electricity production (down to a complete stop) while the bottom gates were gradually opened. As a result, the water level in the reservoir rapidly dropped (Fig. 2a) and the outflow discharge increased progressively (Fig. 2c), reaching the maximum of 177 m3 s−1 on 13th June 2019; the mean outflow discharge value over the entire flushing was 103 ± 32 m3 s−1, an average increase of more 1,800% respect to pre-flushing conditions (i.e., residual flow). Knowing the cross-sectional area (derived from high-resolution bathymetry surveys coupled with 2D hydraulic modelling) and the discharge, average water velocity for the entire flushing period was estimated as 2 m s−1 for the Rienz River in the reach directly downstream the dam, and as 1.5 m s−1 for the Eisack River in the reach downstream the Rienz-Eisack confluence. Estimated average bottom shear stress within the study period in the two above mentioned reaches were 93 and 31 N m−2, respectively. Mean (± SD) SSC recorded in the Rienz River at T1 (369 h measurements) was 2,419 ± 1,814 mg l−1 (corresponding to an average increase calculated for the total flushing period of ~ 2,500% compared to pre-flushing conditions), with values ranging from 64 to 5,770 mg l−1 (Fig. 2b, black line). SSC levels registered in the Eisack River at T2 (441 h measurements) varied between 50 and 6,500 mg l−1 (Fig. 2b, grey line), while mean SSC was 1,520 ± 1,304 mg l−1 (corresponding to an average increase of 2,890% compared to pre-flushing conditions). Overall, the recorded data showed that the sediment wave induced by the flushing travelled along the Rienz and into the Eisack River almost unchanged. The slightly lower SSC peak measured at T1 compared to T2 was most likely due to an underestimation occurred at the former station—presumably caused by extremely high flow aeration—during the few tens of minutes corresponding to the SSC peak. Such interpretation was confirmed by auxiliary data recorded at a third monitoring station (data not used as the station was dislodged by the flow after 8 days of operation, thus not covering the entire flushing event) located in close proximity to site3. Here, the highest SSC value reached 7,000 mg l−1. Sediment concentrations during winter minimum flows in the by-passed river reach were not measured; however, they are surely lower than those measured during pre-flushing conditions, affected to some extent by spring snowmelt runoff.
SSC at T2 was significantly cross-correlated with the one measured in the Rienz River near the dam outlet, with a relatively short time-lag (100 min). In the first 12 h of the flushing, the mean SSC in the Rienz River was 88 ± 23 mg l−1, with a maximum SSC value below 2,086 mg l−1 that corresponds to a SSC equal to 0.3% (expressed as the ratio of the SS volume to the total flushed volume, water + sediments) as requested by local authorities. In the following 6 h, mean SSC values increased by 581%, reaching a mean value of 596 ± 472 mg l−1, with peaks up to 1,336 mg l−1. This was due to a release of almost clean water (e.g., maximum SSC value was 214.73 mg l−1) during the first 12 h from the bottom gates; after ~ 16 h from the start of the flushing operation, SSC values peaked at values higher than 1,000 mg l−1 (see first peak of Fig. 2b).
The durations for selected SSC ranges are shown in Fig. 3. Both SSC time series were characterized by several brief peaks with values reaching 5,000–6,000 mg l−1, corresponding to 4.2% and 0.6% of the overall flushing duration according to T1 and T2, respectively. The highest peaks—with SSC values exceeding 6,000 mg l−1 on five occasions- were recorded by T2 in the first 3 days of the flushing event (6,000–7,000 mg l−1 SSC range, corresponding to 0.1% of the overall duration). These short and repeated peaks, visible in both SSC time series, were probably due to daily variations of inflow into the reservoir, and the sudden collapse of lateral deposited sediment banks being gradually eroded behind the dam. Mechanical digging (see Online Resource 1) in the reservoir was most probably responsible for the SSC peaks visible on 8–9 June for both SSC time series (Fig. 2b). The range of SSC in the Rienz River was 3,000–4,000 mg l−1 (29.2%) for the majority of flushing duration (Fig. 3), the second common-most range was 100–500 mg l−1 (26.7%); SSC in the Eisack River, although characterized by the absolute highest peaks, was for almost half of the time in the range of 100–500 mg l−1 (39.2%), followed by 1,000–3,000 mg l−1 (33.8%).
Selected SSC concentration ranges (mg l−1) durations (%) calculated for SSC measured in the Rienz River (in black) and in the Eisack River (in grey), expressed as percentage of the overall measurement hours of each turbidimeter. The turbidimeter installed in the Rienz River (T1) stopped working on 06-11-19 at ~ 19:00
The measured physical and chemical parameters of surface water did not differ significantly in any of the four sites and will not be discussed any further.
Effects on taxonomic and functional diversity
A total of 40,266 invertebrates, representing 58 taxa, were collected over all sites and sampling occasions, with a mean density of 6,842 ± 5,957 ind m−2 (mean ± SD, calculated combining all sites and sampling occasions). ‘Pre’ samples had the lowest mean density (2,940 ± 2,854 ind m−2), while the highest density was found in ‘post2’ samples (12,033 ± 7,003 ind m−2); ‘post1’ had an intermediate mean density (5,554 ± 2,336 ind m−2). The number of taxa varied between 16 (site2) and 25 (site3 and site4) in ‘pre’ samples, between 20 (site3) and 24 (site1 and site4) in ‘post1’ samples, ‘between 22 (site2 and site3) and 33 (site4) in post2’ samples. Baetis (Ephemeroptera), and the Chironomidae family (Diptera) were the most common taxa throughout the three sampling periods (Fig. 4) (e.g., Chironomidae represented on average more than 54% of total density in ‘post1’ samples).
Taxa richness and Shannon diversity (Fig. 5) declined 40 days after the end of the flushing (‘post1’), but recovered on the longer term (‘post2’). Conversely, macroinvertebrate density and FDis tended to increase over time. However, only invertebrate density differed significantly over time, particularly at the site1 and 2 closer to the dam, showing a remarkable increase in ‘post2’ (LME; P = 0.02, Table 2). This was mainly due to Chironomidae, whose average relative density increased from 31% in ‘post1’ to 57% considering all ‘post2’ samples. Taxa richness, Shannon diversity and FDis were not significantly affected by flushing (P > 0.05 for all metrics, Table 2).
Effects on community composition
The composition of the macroinvertebrate community differed among sampling dates (ANOSIM; R = 0.6157, P = 0.001), and flushing was clearly associated with directional changes in the overall taxonomic composition of the sites. The first two axes of the PCoA ordination explained, respectively, 32% and 18% of the variation; both axes defined the temporal gradients. In fact, the PCoA plot (Fig. 6a) shows three distinct clusters of macroinvertebrate assemblages associated with the three sampling dates, except for site1 in ‘post2’, which appeared more closely associated with samples from ‘post1’. Figure 6a shows that 40 days after the event (‘post1’), communities shifted along the first PCoA axis, but then showed a weak recovery towards pre-flushing conditions at ‘post2’, shifting along the second PCoA axis.
a PCoA plot of benthic macroinvertebrate communities based on Bray–Curtis dissimilarity. Numbers indicate site identity and polygons depict the three sampling dates. b FCA plot of macroinvertebrate functional composition, as described by the traits categories and modalities listed in Table 1. Crosses within the polygons indicate the group (date) centroid. Dashed grey line shows the trajectory of compositional changes during the flushing event
The results of the SIMPER analysis (Online Resource 3) listed the following taxa as characterizing the ‘pre’ samples Ephemeroptera Ecdyonurus sp. (total abundance 347 ind m−2), the Trichoptera Rhyacophila aurata Brauer, 1857 (81 ind m−2), the Plecoptera Isoperla sp., (67 ind m−2), Protonemoura sp. (1,742 ind m−2), and Leuctra sp. (272 ind m−2), and the Diptera Dicranota sp. (119 ind m−2). A more diverse assemblage characterized the post samples: the Trichoptera Hydropsyche sp. (70 ind m−2), Hyporhyacophila spp. (22 ind m−2), Pararhyacophila spp. (20 ind m−2), juvenile Ephemeroptera (3,260 ind m−2), and Diptera Blephariceridae (143 ind m−2) in ‘post1’; the Trichoptera Rhyacophila sp. (488 ind m−2), Rhyacophila gr. dorsalis (28 ind m−2), Hydropsyche angustipennis (Curtis, 1834) (22 ind m−2) and unidentified juveniles (126 ind m−2), the Ephemeroptera Rhithrogena sp. (980 ind m−2), and Baetis sp. (15,088 ind m−2), the Plecoptera Capnopsis schilleri (Rostock, 1892) (68 ind m−2), and unidentified juveniles (2,946 ind m−2), Diptera Simuliidae (8,009 ind m−2) and Ceratopogonidae (85 ind m−2), Crustacea Ostracoda (150 ind m−2) and Cladocera (62 ind m−2) in ‘post2’.
The lowest similarity was observed between ‘pre’ and ‘post2’ samples (i.e., the smallest Bray–Curtis similarity between samples, 59.24), while the largest occurred between ‘post1’ and ‘post2’ (i.e., Bray–Curtis similarity between samples, 65.46). The analyses also indicated that ‘post1’ samples where the least variable spatially (i.e., highest Bray–Curtis similarity within samples, 74.18), ‘post2’ the most variable (i.e., lowest Bray–Curtis similarity within samples, 64.70), while the ‘pre’ samples had an intermediate similarity value (68.88). This was further supported by the “betadisper” analysis, which showed that average distance to group centroid was 0.19, 0.15 and 0.21, respectively, for ‘pre’, ‘post1’ and ‘post2’ samples, further supporting the homogenisation effect of the flushing observed after 40 days, followed by a divergence after approximately 2.5 months (i.e., 74 days).
The trajectory of individual sites in multidimensional space differed. In particular, the FCA plot (Fig. 6b) suggests that changes in site1 were more substantial in terms of functional composition. The first two axes of the FCA explained, respectively, 63% and 23% of the variation in functional space. Flushing was associated with a shift along the first FCA axis, as we all a substantial shift of site1 along the second axis. Table 3 reports the loadings of each trait modality on the two axes, which were used to select specific trait modalities (with loadings > |0.6| on axis1; Table 3) for subsequent univariate LME tests. The LME models (Table 2) showed that flushing was associated with an increase in the relative proportion of taxa with large body size (size 2–4 cm), with sessile life (sessile organisms) or passive swimmers (passive swimmers), taxa living preferentially in muddy substrates (mud) and on periphyton (periphyton). Effects on feeding traits were also evident; passive filter feeders increased (filter feeders) and shredders decreased, with significant decline at ‘post2’ (shredders), particularly at site1 (Fig. 7). Figure 7 shows the response of selected trait modalities to the flushing.
Discussion
Characteristics of the flushing event
Suspended sediments increased remarkably in the studied sections of the Rienz and Eisack Rivers during the 19-day sediment flushing event from the Rio di Pusteria reservoir. The registered SSCs were in line with turbidity values observed during a natural flood such as the windstorm Vaia that hit NE Italy on 27–30 October 2018; on that occasion, SSC in the Eisack River peaked at 11,700 mg l−1 with mean values over 2,800 mg l−1 (SSC measured in Bolzano-Bozen, data provided by the Hydrographic Office of the Autonomous Province of Bolzano-Bozen). Yet, the duration of the 2019 flushing was much longer than what is naturally experienced by riverine invertebrates (e.g., the windstorm Vaia) in such a regulated river system.
Hypothesis 1: flushing causes a rapid decline in invertebrate diversity
In our study, we did not observe a significant decline in overall diversity, but rather an increase in density 74 days after the flushing in the sites closest (3.5–7 km) to the dam. In the sites located 11–19 km from the dam, after an initial increase in density 40 days after the flushing, densities increased negligibly or slightly decreased after 74 days. Thus, the 1st hypothesis is only partially supported by site1 displaying a decline in density, but a subsequent rapid recovery to higher density after 74 days. Also, no clear evidence was found that the impact of flushing would reflect sites’ proximity to the dam in terms of diversity metrics, with a general decline 40 days after the flushing, but a recovery after 74 days.
Sediment flushing is necessarily associated with increases in discharge (and associated increases in shear stress and substrate mobilization), variations of suspended sediment load and accumulation of fine sediment when the discharge decreases once operations are terminated. The individual effects of these factors (Gibbins et al., 2007a; Doretto et al., 2016, 2018; Béjar et al., 2017) are difficult to disentangle, but flushing operations generally have short-term impacts on benthic communities, typically represented by a reduction of density and richness immediately after the event (Espa et al., 2019). In a recent review of ten controlled sediment flushing operations carried out in the central Italian Alps, Espa et al. (2019) reported a decrease in macroinvertebrate density of more than 70%, and a drop in richness by 10–60% about one month after the flushing. The same study reports a recovery time ranging from 3 to 6 months, depending on the sediment dose (sensu Newcombe & Jensen, 1996), the flushing season, the persistence of the riverbed alteration, and the possible recolonization from unaltered upstream reaches (Espa et al., 2019, and references therein). The recovery time considered in our study (about 2.5 months) is therefore shorter than those reviewed by Espa et al. (2019); but the characteristics of the Rio di Pusteria reservoir, the related hydropower plant, and the flushing event are only partly comparable to those reviewed by the authors. Despite the average suspended sediment concentration recorded during the flushing was in the range of those observed by Espa et al. (2019), the mean discharge was much higher (103 m3 s−1 vs. 1–70 m3 s−1), as was the total amount of flushed sediment (~ 265 103 t vs. 4.6–74.9 103 t). Deposition of around 4–5 kg m−2 or less of fine sediments can significantly affect benthic assemblages (Larsen & Ormerod, 2010; Larsen et al., 2011), while sediment pulses can trigger massive drift independently from discharge variation (Bruno et al., 2016). Therefore, we did expect a relatively stronger response of macroinvertebrates to flushing in this study. However, the minor effects recorded on taxonomic metrics might be due to the greater discharge, which certainly helped in reducing the amount of deposited fines. In the several case studies analysed by Espa et al. (2019), faster recovery was in fact recorded at sites where freshwater releases from tributaries were used as mitigation measures. Our results appear to support the results of Espa et al. (2019), i.e., seasonality and site characteristics were more relevant than the sediment dose in determining the macroinvertebrate communities recovery to pre-flushing conditions.
Hypothesis 2: flushing causes a homogenisation of the macroinvertebrate taxonomic assemblages
This hypothesis was fully supported as the combination of increased SSCs and discharge during the flushing was associated with an initial homogenization of macroinvertebrate communities, followed by a divergence in composition. As hypothesised, the flushing disturbance likely increased the longitudinal connectivity of the rivers network, promoting organisms’ dispersal between up- and downstream communities, as suggested by the increased similarity among the sites 40 days after the event. Besides the relatively short-term homogenizing effect, the flushing substantially changed the overall composition of the communities, which did not recover to pre-disturbance conditions even after 74 days.
A large, unpredictable flow disturbance like a 19-day flushing event may indeed represent a dominant structuring force for stream ecosystems, effectively resetting assemblages to earlier successional stages (Death, 2010; Larsen et al., 2019). However, it is difficult to determine whether the observed effects of flushing were linked to higher concentrations of suspended solids (Doeg & Milledge, 1991) or to an increase in hydraulic force (Gibbins et al., 2007a) or to their interaction (Béjar et al., 2017). Although we did not directly measure it, invertebrate drift—either active, also called behavioural, or passive, also named catastrophic or mass drift—may be the likely mechanism driving the observed patterns. The direct effect of increased flow velocities and bottom shear stress during the first phase of the Rienz flushing likely represented alone a disturbance to the benthic communities, which was capable to trigger bed-movement and initiate invertebrates drift, as previously shown by Bond and Downes (2003) in flume experiments. Similarly, Gibbins et al. (2007a) found that once shear stress exceeds the threshold of 9 N m−2, bedload transport occurs and invertebrates drift increases. Given that we estimated peak shear stress values around 31–93 N m−2, rapid invertebrate drift is likely to have occurred during the initial phase of flushing. Moreover, experimental studies showed that, at a given discharge, the transport of sediment added to streams can increase the rate of behavioural drift, causing subsequent declines in benthic abundances (Culp et al., 1986; Doeg & Milledge, 1991). Hence, most likely, the compositional responses to flushing that we observed are due to the combined effect of increased suspended loads and discharge.
Hypothesis 3: flushing affects sediment and flow sensitive taxa and changes the functional composition of the macroinvertebrate assemblages
Observed changes in taxonomic (and functional) composition indicated that flow and sediment-sensitive taxa were particularly impacted by the flushing operations.
The taxa which were abundant and mostly associated with the ‘pre’ flushing samples were the Plecoptera Protonemoura sp., Leuctra sp., and the Ephemeroptera Ecdyonurus sp. The latter belongs to the Heptageniidae family, which is known to be highly sensitive to fine sediments (Extence et al., 2013; Turley et al., 2016). Although well adapted to tolerate high flow velocities and shear stress (i.e., possessing a dorso-ventrally flattened body that reduce the risk of dislodgement, Lancaster & Belyea, 2006), species of the genus Ecdyonurus require clean substrate surfaces for feeding (Rabení et al., 2005), and show indeed high propensity to drift when fine sand is deposited on the riverbed (Larsen & Ormerod, 2010). Only about 10% sediment cover is considered stressful for most Ecdyonurus sp. (Bryce et al., 2010). Similarly, individuals of the Protonemoura sp. might have become rare in the ‘post’ samples because of the effect of deposited and saltating fines that reduce the quality and availability of coarse and fine particulate organic matter from which they feed on (Navel et al., 2010; Doretto et al., 2017), and all Plecoptera are well known for requiring clean and well oxygenated waters with low turbidity (Chapman, 1996). These results are partly comparable to those of Espa et al. (2013) who monitored a sequence of 2-weeks yearly flushing over 3 years, and recorded a decrease in macroinvertebrates diversity associated with the loss of species sensitive to increased riverbed shear stress, such as Heptageniidae. All these sensitive taxa characterizing the ‘pre’ samples strongly declined in abundance after the flushing. The remaining taxa/individuals present in the ‘post’ samples were those that either resisted/survived the flushing, or recolonized the reaches after the event. The ‘post’ flushing benthic communities were mainly composed of Trichoptera Rhyacophila sp. and Hydropsyche sp. (the latter present only in the ‘post’ samples), the Ephemeroptera Rhithrogena sp. and Baetis sp., juvenile Ephemeroptera and Plecoptera, and Diptera Simuliidae. The high abundance of juveniles was possibly related to the phenology of the taxa which are typically bivoltine, with the second generation developing during summer to emerge in the fall; early larval instars often dominate in the shallow hyporheic sediment where they are sheltered from impacts occurring above in the benthic zone (Stubbington, 2012), thus increasing their chance of surviving the flushing. As reported in literature (Jones et al., 2012, and references therein), the taxa most likely to drift during high loads of suspended fines are either ubiquitous, opportunistic taxa with high mobility (e.g., Baetidae, Rhithrogena sp.), or filter feeders whose guts fill with inert particles (e.g., Simuliidae), or whose nets become clogged with fine sediments (e.g., Hydropsychidae). This might explain the high abundances of Baetis sp., Simuliidae and the exclusive presence of Hydropsyche sp. in the ‘post’ samples, which could have drifted from the upstream reaches during the flushing. Rhyacophilidae are among the main predators of Simuliidae (Malmqvist & Sackmann, 1996), and the greater abundance of Rhyacophilidae in ‘post’ samples may also be related to the high abundance of prey (i.e., Simuliidae, among others).
Even though we excluded Chironomidae from our functional analysis, and this taxon is known to be highly adapted to live in fine sediments (Wood & Armitage, 1997), we detected important changes in the functional structure of macroinvertebrate communities after the flushing. In fact, the functional analysis indicated that flushing greatly altered the relative proportion of feeding traits at the upstream-most site (3.5 km from the dam), with a dramatic reduction in shredders up to 74 days after the event. Taxonomic changes caused by the flushing were accompanied by evident functional shifts, because the environmental changes associated with the flushing event represented selective filters that favoured relatively large body-sized taxa, taxa displaying high affinity for mud and/or periphyton substrates, which were either tightening to hard substrate, plants or other animals or drifting passively, and primarily filter-feeding on suspended FPOM. Conversely, shredders appeared particularly affected. The fact that relatively large body size organisms were favoured by the flushing disturbance may suggest that this trait modality could be associated with a sessile or clinging behaviour of taxa such as Simuliidae and Trichoptera Rhyacophila sp. and Hydropsyche sp. characterizing the ‘post’ samples. However, Wilkes et al. (2017) analysed the fine sediment indices commonly used in biomonitoring and found that body size was not an informative trait. Wilkes et al. (2017) also reported ‘shredder’ as the only trait which was constantly associated with sensitivity to fine sediment, possibly due to the burial of leaf litter and/or the reduction in its nutritional quality through inhibition of fungal growth (Wilkes et al., 2017). Similarly, Doretto et al. (2016) investigated a low-order Alpine stream and reported how the increase of fine sediment reduced the amount of coarse particulate organic matter, and this consequently affected the abundance of invertebrate shredders. These data are consistent with our results which indicated that flushing greatly altered the relative proportion of feeding traits at site1, with a dramatic reduction in shredders up to 74 days after the event.
Besides altering streambed habitat structure, sediment flushing from dams can also release large amounts of nutrients that can promote periphyton growth in-stream (Asaeda et al., 2015). This could explain the observed increase in the proportion of taxa using periphyton as a habitat rather than food. Taxa swimming passively could be favoured by their ability to search shelter in more protected areas such as riparian vegetation (Robinson et al., 2004), and promptly recover after the disturbance ceases. The increased abundance of sessile organisms after the flushing could also reflect their ability to withstand high flows and scour. Taxa able to adhere to hard substrata by means of suckers or adhesives body parts, for example, and to avoid fine sediment transport disturbance via deliberately drifting downstream, display higher resistance and resilience compared to those invertebrates entrained in the water column along with fine sediment (Gibbins et al., 2007b).
We recorded an increase in density of filter-feeder Simuliidae after the flushing event. Although the transport of fine sediments is considered detrimental to filter-feeding organisms such as blackflies (Jones et al., 2012), a significant increase in filter feeders could reflect the higher availability of suspended particles (Fuller et al., 2010), and the higher densities recorded after the flushing could represent a trade-off between the risk of damages to the filtering devices and the higher availability of suspended food particles. Alternatively, the high densities of blackflies recorded after the flushing may be associated to phenology (i.e., Simuliidae are mainly bivoltine in the investigated geographical area, with the second generation quickly developing in summer) and to behavioural drift from upstream reaches. Naman et al. (2016) pointed out that the first flow-related threshold increasing catastrophic drift is represented by a critical level of shear stress causing organic matter entrainment, with animals using detritus or algal mats as substrate. Bruno et al. (2016) listed Simuliidae as one of the dominant taxa drifting behaviourally during a simulated sediment flushing.
Conclusions
This study showed that pulse disturbance such as flushing events can have immediate and lasting consequences on bottom dwelling organisms, with potential implications for food web and ecosystem dynamics (Ledger et al., 2012). Although the effects on diversity were apparently moderate, compositional and functional changes were evident and persisted for more than 2 months, with full recovery to pre-disturbance conditions not yet observed, at least for the time span of the study (i.e., 84 days in total).
In conclusion, our results suggest that the recovery of invertebrate communities from a pulse disturbance in dammed rivers can have complex recovery time frames; compositional recovery in fragmented systems such as dammed rivers is expected to be slower compared to more open systems where dispersal from un-impacted reaches is allowed. This supports the hypothesis that sensitive species need longer recovery time, and further indicates that in the absence of functional redundancy, i.e., substitution of one species with another performing similar roles in communities and ecosystems (Rosenfeld, 2002), altered species composition jeopardises the full functional recovery. Finally, our analysis demonstrates that resistance traits of pioneer and sediment-tolerant taxa are important for the persistence of invertebrate communities after a reservoir sediment flushing.
Studies specifically assessing the ecological effects of dam flushing operations are limited in number (e.g., Crosa et al., 2010; Gomi et al., 2010; Doretto et al., 2019; Espa et al., 2019), and this study provides further insights on their potential ecological impacts. Results indicate that managing reservoir siltation through flushing operations can significantly alter macroinvertebrate assemblages up to 2.5 months after the operation, with little sign of recovery.
As dam management operations to desilt reservoirs are likely to become more frequent due to the combined effects of climate change and flow abstraction on sediment transfer (Bakker et al., 2018), we argue that coordinated and standardised effort is needed to investigate the ecological implications over the longer terms, and in different ecological settings. This could provide the basis for implementing more sustainable management schemes, also in highly regulated streams such as those investigated here. Different flushing management (e.g., shorter but more frequent flushing mimicking natural sediment regimes for each specific river) and different timing of operations (e.g., after the emergence period of more sensitive species) would likely lead to different ecological implications, but this needs to be properly verified by monitoring several flushing under different structural and operational conditions.
Data availability (data transparency)
Not applicable.
Code availability (software application or custom code)
Not applicable.
References
Arheimer, B., C. Donnelly & G. Lindström, 2017. Regulation of snow-fed rivers affects flow regimes more than climate change. Nature Communications 8: 62.
Asaeda, T., M. H. Rashid & H. L. K. Sanjaya, 2015. Flushing sediment from reservoirs triggers forestation in the downstream reaches. Ecohydrology 8: 426–437.
Baird, D. J., M. N. Rubach & P. J. Van den Brink, 2008. Trait-based ecological risk assessment (TERA): the new frontier? Integrated Environmental Assessment and Management 4: 2–3.
Bakker, M., A. Costa, T. A. Silva, L. Stutenbecker, S. Girardclos, J.-L. Loizeau, P. Molnar, F. Schlunegger & S. N. Lane, 2018. Combined flow abstraction and climate change impacts on an aggrading Alpine River. Water Resources Research 54: 223–242.
Bates, D., M. Mächler, B. M. Bolker & S. C. Walker, 2015. Fitting linear mixed-effects models using lme4. Journal of Statistical Software American Statistical Association 67: 1–48.
Béjar, M., C. N. Gibbins, D. Vericat & R. J. Batalla, 2017. Effects of suspended sediment transport on invertebrate drift. River Research and Applications 33: 1655–1666.
Belfiore, C., 1983. Efemerotteri. Consiglio Nazionale delle Ricerche. Collana del progetto “Promozione della qualità dell’ambiente” AQ./1/201, Guide per il riconoscimento delle specie animali delle acque interne italiane, Roma.
Bo, T., S. Fenoglio, G. Malacarne, M. Pessino & F. Sgariboldi, 2007. Effects of clogging on stream macroinvertebrates: an experimental approach. Limnologica 37: 186–192.
Bona, F., A. Doretto, E. Falasco, V. La Morgia, E. Piano, R. Ajassa & S. Fenoglio, 2016. Increased sediment loads in alpine streams: an integrated field study. River Research and Applications 32: 1316–1326.
Bond, N. R. & B. J. Downes, 2003. The independent and interactive effects of fine sediment and flow on benthic invertebrate communities characteristic of small upland streams. Freshwater Biology 48: 455–465.
Bruno, M. C., M. Carolli, B. Palmia & G. Zolezzi, 2016. Effects of fine suspended sediment releases on benthic communities in artificial flumes. La Houille Blanche. https://doi.org/10.1051/LHB/2016061.
Bruno, M. C. & A. Siviglia, 2012. Assessing impacts of dam operations-interdisciplinary approaches for sustainable regulated river management. River Research and Applications 28: 675–677.
Bryce, S. A., G. A. Lomnicky & P. R. Kaufmann, 2010. Protecting sediment-sensitive aquatic species in mountain streams through the application of biologically based streambed sediment criteria. Journal of the North American Benthological Society 29: 657–672.
Buendia, C., C. N. Gibbins, D. Vericat, R. J. Batalla & A. Douglas, 2013. Detecting the structural and functional impacts of fine sediment on stream invertebrates. Ecological Indicators 25: 184–196.
Campaioli, S., P. F. Ghetti, A. Minelli & S. Ruffo, 1994. Manuale per il riconoscimento dei macroinvertebrati delle acque dolci italiane, Vol. I. Trento, Provincia Autonoma di Trento.
Campaioli, S., P. F. Ghetti, A. Minelli & S. Ruffo, 1999. Manuale per il riconoscimento dei macroinvertebrati delle acque dolci italiane, Vol. II. Trento, Provincia Autonoma di Trento.
Chapman, D., 1996. Water Quality Assessment: A Guide to the Use of Biota, Sediments and Water in Environmental Monitoring. Taylor & Francis, London.
Chevene, F., S. Doléadec & D. Chessel, 1994. A fuzzy coding approach for the analysis of long-term ecological data. Freshwater Biology 31: 295–309.
Clarke, K. R., 1993. Non-parametric multivariate analyses of changes in community structure. Austral Ecology 18: 117–143.
Clarke, K. R. & R. N. Gorley, 2015. PRIMER v7: User Manual/Tutorial. PRIMER-E Ltd, Plymouth: 300.
Crosa, G., E. Castelli, G. Gentili & P. Espa, 2010. Effects of suspended sediments from reservoir flushing on fish and macroinvertebrates in an alpine stream. Aquatic Sciences 72: 85–95.
Culp, J. M., F. J. Wrona & R. W. Davies, 1986. Response of stream benthos and drift to fine sediment deposition versus transport. Canadian Journal of Zoology 64: 1345–1351.
Death, R. G., 2010. Disturbance and riverine benthic communities: what has it contributed to general ecological theory? River Research and Applications 26: 15–25.
Doeg, T. & G. Milledge, 1991. Effect of experimentally increasing concentration of suspended sediment on macroinvertebrate drift. Marine and Freshwater Research 42: 519.
Dolédec, S. & B. Statzner, 2008. Invertebrate traits for the biomonitoring of large European rivers: an assessment of specific types of human impact. Freshwater Biology 53: 617–634.
Dolédec, S. & B. Statzner, 2010. Responses of freshwater biota to human disturbances: contribution of J-NABS to developments in ecological integrity assessments. Journal of the North American Benthological Society 29: 286–311.
Doretto, A., T. Bo, F. Bona, M. Apostolo, D. Bonetto & S. Fenoglio, 2019. Effectiveness of artificial floods for benthic community recovery after sediment flushing from a dam. Environmental Monitoring and Assessment 191: 88.
Doretto, A., F. Bona, E. Falasco, E. Piano, P. Tizzani & S. Fenoglio, 2016. Fine sedimentation affects CPOM availability and shredder abundance in Alpine streams. Journal of Freshwater Ecology 31: 299–302.
Doretto, A., F. Bona, E. Piano, I. Zanin, A. C. Eandi & S. Fenoglio, 2017. Trophic availability buffers the detrimental effects of clogging in an alpine stream. Science of The Total Environment 592: 503–511.
Doretto, A., E. Piano, F. Bona & S. Fenoglio, 2018. How to assess the impact of fine sediments on the macroinvertebrate communities of alpine streams? A selection of the best metrics. Ecological Indicators 84: 60–69.
Enquist, B. J., J. Norberg, S. P. Bonser, C. Violle, C. T. Webb, A. Henderson, L. L. Sloat, & V. M. Savage, 2015. Scaling from Traits to Ecosystems: developing a General Trait Driver Theory via Integrating Trait-Based and Metabolic Scaling Theories In Pawar, S., G. Woodward, & A. I. B. T.-A. in E. R. Dell (eds), Trait-Based Ecology - From Structure to Function. Academic Press: 249–318.
Espa, P., R. J. Batalla, M. L. Brignoli, G. Crosa, G. Gentili & S. Quadroni, 2019. Tackling reservoir siltation by controlled sediment flushing: impact on downstream fauna and related management issues. PLoS ONE 14:e0218822
Espa, P., M. L. Brignoli, G. Crosa, G. Gentili & S. Quadroni, 2016. Controlled sediment flushing at the Cancano Reservoir (Italian Alps): management of the operation and downstream environmental impact. Journal of Environmental Management 182: 1–12.
Espa, P., E. Castelli, G. Crosa & G. Gentili, 2013. Environmental effects of storage preservation practices: controlled flushing of fine sediment from a small hydropower reservoir. Environmental Management 52: 261–276.
Extence, C. A., R. P. Chadd, J. England, M. J. Dunbar, P. J. Wood & E. D. Taylor, 2013. The assessment of fine sediment accumulation in rivers using macro-invertebrate community response. River Research and Applications 29: 17–55.
Fette, M., C. Weber, A. Peter & B. Wehrli, 2007. Hydropower production and river rehabilitation: a case study on an alpine river. Environmental Modeling and Assessment 12: 257–267.
Fochetti, R. & J. M. Tierno De Figueroa, 2008. Plecoptera Fauna d’Italia, Vol. XLIII. Calderini Editore, Bologna.
Fuller, R. L., C. Griego, J. D. Muehlbauer, J. Dennison & M. W. Doyle, 2010. Response of stream macroinvertebrates in flow refugia and high-scour areas to a series of floods: a reciprocal replacement study. Journal of the North American Benthological Society 29: 750–760.
Gibbins, C., D. Vericat & R. J. Batalla, 2007a. When is stream invertebrate drift catastrophic? The role of hydraulics and sediment transport in initiating drift during flood events. Freshwater Biology 52: 2369–2384.
Gibbins, C., D. Vericat, R. J. Batalla & C. M. Gomez, 2007b. Shaking and moving: low rates of sediment transport trigger mass drift of stream invertebrates. Canadian Journal of Fisheries and Aquatic Sciences 64: 1–5.
Gomi, T., S. Kobayashi, J. N. Negishi & F. Imaizumi, 2010. Short-term responses of macroinvertebrate drift following experimental sediment flushing in a Japanese headwater channel. Landscape and Ecological Engineering 6: 257–270.
Gray, J. R., & J. W. Gartner, 2009. Technological advances in suspended-sediment surrogate monitoring. Water Resources Research 45: W00D29.
Grimardias, D., J. Guillard & F. Cattanéo, 2017. Drawdown flushing of a hydroelectric reservoir on the Rhône River: impacts on the fish community and implications for the sediment management. Journal of Environmental Management 197: 239–249.
Hering, D., A. Buffagni, O. Moog, L. Sandin, M. Sommerhäuser, I. Stubauer, C. Feld, R. Johnson, P. Pinto, N. Skoulikidis, P. Verdonschot & S. Zahrádková, 2003. The development of a system to assess the ecological quality of streams based on macroinvertebrates-design of the sampling programme within the AQEM Project. International Review of Hydrobiology 88: 345–361.
Jones, J. I., J. F. Murphy, A. L. Collins, D. A. Sear, P. S. Naden & P. D. Armitage, 2012. The impact of fine sediment on macro-invertebrates. River Research and Applications 28: 1055–1071.
Jost, L., 2006. Entropy and diversity. Oikos 113: 363–375.
Kjelland, M. E., C. M. Woodley, T. M. Swannack & D. L. Smith, 2015. A review of the potential effects of suspended sediment on fishes: potential dredging-related physiological, behavioral, and transgenerational implications. Environment Systems and Decisions 35: 334–350.
Kondolf, G. M., Y. Gao, G. W. Annandale, G. L. Morris, E. Jiang, J. Zhang, Y. Cao, P. Carling, K. Fu, Q. Guo, R. Hotchkiss, C. Peteuil, T. Sumi, H.-W. Wang, Z. Wang, Z. Wei, B. Wu, C. Wu & C. T. Yang, 2014. Sustainable sediment management in reservoirs and regulated rivers: experiences from five continents. Earth’s Future American Geophysical Union (AGU) 2: 256–280.
Laliberté, E., P. Legendre, & B. Shipley, 2014. FD: measuring functional diversity from multiple traits, and other tools for functional ecology. R package version 1.0-12.
Lancaster, J. & L. R. Belyea, 2006. Defining the limits to local density: alternative views of abundance-environment relationships. Freshwater Biology 51: 783–796.
Larsen, S. & S. J. Ormerod, 2010. Low-level effects of inert sediments on temperate stream invertebrates. Freshwater Biology 55: 476–486.
Larsen, S., G. Pace & S. J. Ormerod, 2011. Experimental effects of sediment deposition on the structure and function of macroinvertebrate assemblages in temperate streams. River Research and Applications 27: 257–267.
Larsen, S., U. Karaus, C. Claret, F. Sporka, L. Hamerlík & K. Tockner, 2019. Flooding and hydrologic connectivity modulate community assembly in a dynamic river-floodplain ecosystem. PLoS ONE 14:e0213227
Ledger, M. E., L. E. Brown, F. K. Edwards, A. M. Milner & G. Woodward, 2012. Drought alters the structure and functioning of complex food webs. Nature Climate Change 2: 1–5.
Leibold, M. A., M. Holyoak, N. Mouquet, P. Amarasekare, J. M. Chase, M. F. Hoopes, R. D. Holt, J. B. Shurin, R. Law, D. Tilman, M. Loreau & A. Gonzalez, 2004. The metacommunity concept: a framework for multi-scale community ecology. Ecology Letters 7: 601–613.
Malmqvist, B. & G. Sackmann, 1996. Changing risk of predation for a filter-feeding insect along a current velocity gradient. Oecologia 108: 450–458.
McGill, B. J., B. J. Enquist, E. Weiher & M. Westoby, 2006. Rebuilding community ecology from functional traits. Trends in Ecology and Evolution 21: 178–185.
McKenzie, M., K. L. Mathers, P. J. Wood, J. England, I. Foster, D. Lawler & M. Wilkes, 2020. Potential physical effects of suspended fine sediment on lotic macroinvertebrates. Hydrobiologia 847: 697–711.
Meile, T., J. L. Boillat & A. J. Schleiss, 2011. Hydropeaking indicators for characterization of the Upper-Rhone River in Switzerland. Aquatic Sciences 73: 171–182.
Moretti, G. P., 1983. Tricotteri. Consiglio Nazionale delle Ricerche. Collana del progetto “Promozione della qualità dell’ambiente” AQ./1/201, Guide per il riconoscimento delle specie animali delle acque interne italiane, Roma.
Morris, G. L. & J. Fan, 1988. Reservoir Sedimentation Handbook: Design and Management of Dams. Reservoirs and Watersheds for Sustainable Use. McGraw-Hill Book Co., New York.
Naman, S. M., J. S. Rosenfeld & J. S. Richardson, 2016. Causes and consequences of invertebrate drift in running waters: from individuals to populations and trophic fluxes. Canadian Journal of Fisheries and Aquatic Sciences 73: 1292–1305.
Navel, S., F. Mermillod-Blondin, B. Montuelle, E. Chauvet, L. Simon, C. Piscart & P. Marmonier, 2010. Interactions between fauna and sediment control the breakdown of plant matter in river sediments. Freshwater Biology 55: 753–766.
Newcombe, C. P. & J. O. T. Jensen, 1996. Channel suspended sediment and fisheries: a synthesis for quantitative assessment of risk and impact. North American Journal of Fisheries Management 16: 693–727.
Oksanen, J., F. G. Blanchet, M. Friendly, R. Kindt, P. Legendre, D. McGlinn, P. R. Minchin, R. B. O’Hara, G. L. Simpson, P. Solymos, E. Stevens, M. H. H., Szoecs, & H. Wagner, 2019. Vegan: community ecology package. R package version 2.5-6. .
Paradis, E. & K. Schliep, 2019. ape 5.0: an environment for modern phylogenetics and evolutionary analyses in R. Bioinformatics Oxford University Press 35: 526–528.
Petts, G. E. & A. M. Gurnell, 2005. Dams and geomorphology: research progress and future directions. Geomorphology 71: 27–47.
R Core Team, 2019. R: a language and environment for statistical computing. R Foundation for Statistical Computing, Vienna, Austria. https://www.R-project.org/. Vienna, Austria.
Rabení, C. F., K. E. Doisy & L. D. Zweig, 2005. Stream invertebrate community functional responses to deposited sediment. Aquatic Sciences 67: 395–402.
Reid, A. J., A. K. Carlson, I. F. Creed, E. J. Eliason, P. A. Gell, P. T. J. Johnson, K. A. Kidd, T. J. MacCormack, J. D. Olden, S. J. Ormerod, J. P. Smol, W. W. Taylor, K. Tockner, J. C. Vermaire, D. Dudgeon & S. J. Cooke, 2019. Emerging threats and persistent conservation challenges for freshwater biodiversity. Biological Reviews 94: 849–873.
Robinson, C. T., S. Aebischer & U. Uehlinger, 2004. Immediate and habitat-specific responses of macroinvertebrates to sequential, experimental floods. Journal of the North American Benthological Society 23: 853–867.
Rosenfeld, J. S., 2002. Functional redundancy in ecology and conservation. Oikos 98: 156–162.
Schmidt-Kloiber, A. & D. Hering, 2015. www.freshwaterecology.info – an online tool that unifies, standardises and codifies more than 20,000 European freshwater organisms and their ecological preferences. Ecological Indicators 53: 271–282.
Statzner, B., S. Dolédec & B. Hugueny, 2004. Biological trait composition of European stream invertebrate communities: assessing the effects of various trait filter types. Ecography 27: 470–488.
Stubbington, R., 2012. The hyporheic zone as an invertebrate refuge: a review of variability in space, time, taxa and behaviour. Marine and Freshwater Research 63: 293–311.
Tachet, H., P. Richoux, M. Bournaud & Usseglio-Polatera, 2010. Invertébrés d’eau douce systématique, biologie, écologie. CNRS éditions, Paris.
Thorp, J. H., M. C. Thoms & M. D. Delong, 2006. The riverine ecosystem synthesis: biocomplexity in river networks across space and time. River Research and Applications 22: 123–147.
Trimble, S. W., B. Wilson, R. Herschy, B. Dargahi, H. Chanson, R. W. Herschy, R. W. Herschy, J. Albergel, V. V. S. G. Rao, E. Carmack, N. Filatov, T. Gronskaya, V. A. Rumyantsev, S. A. Kondratyev, A. V. Izmaylova, & V. P. Saltankin, 2012. Reservoir Sedimentation Encyclopedia of Earth Sciences Series. Springer: 628–649
Turley, M. D., G. S. Bilotta, R. P. Chadd, C. A. Extence, R. E. Brazier, N. G. Burnside & A. G. G. Pickwell, 2016. A sediment-specific family-level biomonitoring tool to identify the impacts of fine sediment in temperate rivers and streams. Ecological Indicators 70: 151–165.
Van Looy, K., J. D. Tonkin, M. Floury, C. Leigh, J. Soininen, S. Larsen, J. Heino, N. LeRoy Poff, M. Delong, S. C. Jähnig, T. Datry, N. Bonada, J. Rosebery, A. Jamoneau, S. J. Ormerod, K. J. Collier & C. Wolter, 2019. The three Rs of river ecosystem resilience: resources, recruitment, and refugia. River Research and Applications 35: 107–120.
Verberk, W. C. E. P., C. G. E. Van Noordwijk & A. G. Hildrew, 2013. Delivering on a promise: integrating species traits to transform descriptive community ecology into a predictive science. Freshwater Science 32: 531–547.
Vörösmarty, C. J., P. B. Mcintyre, M. O. Gessner, D. Dudgeon, A. Prusevich, P. Green, S. Glidden, S. E. Bunn, C. A. Sullivan, C. Reidy Liermann & P. M. Davies, 2010. Global threats to human water security and river biodiversity. Nature 467: 555–561.
Waringer, J. & W. Graf, 2011. Atlas der mitteleuropäischen Köcherfliegenlarven. Erik Mauch Verlag, Germany.
Warton, D. I. & F. K. C. Hui, 2011. The arcsine is asinine: the analysis of proportions in ecology. Ecology 92: 3–10.
Wilkes, M. A., M. Mckenzie, J. F. Murphy & R. P. Chadd, 2017. Assessing the mechanistic basis for fine sediment biomonitoring: inconsistencies among the Literature, Traits and Indices. River Research and Applications 33: 1618–1629.
Wohl, E., B. P. Bledsoe, R. B. Jacobson, N. L. Poff, S. L. Rathburn, D. M. Walters & A. C. Wilcox, 2015. The natural sediment regime in rivers: broadening the foundation for ecosystem management. BioScience 65: 358–371.
Wood, P. J. & P. D. Armitage, 1997. Biological effects of fine sediment in the lotic environment. Environmental Management 21: 203–217.
Acknowledgments
This study would not have been possible without the help of numerous people, first in installing the turbidimeters, and secondly in collecting invertebrates. For their help, SF wishes to thank Velio Coviello, Gianluca Vignoli, Silvia Simoni, Corrado Lucarelli, Alessio Bendazzoli, Giulia Stradiotti, Sebastiano Piccolroaz, Francesca Vallefuoco, Miyata Sushuke. SF extends her thankfulness to Alperia spa for allowing us to carry out this work and providing the reservoir water levels, and to the Environmental Protection Agency of the Province of Bolzano-Bozen for analysing water samples. Two anonymous reviewers greatly helped to improve the manuscript with their constructive comments.
Funding
Partial financial support was received from the project Sediplan-r (FESR1002), financed by the European Regional Development Fund (ERDF) Investment for Growth and Jobs Programme 2014–2020.
Author information
Authors and Affiliations
Corresponding author
Ethics declarations
Conflict of interest
The authors have no conflicts of interest or competing interest to declare that are relevant to the content of this article.
Additional information
Handling editor: María del Mar Sánchez-Montoya
Publisher's Note
Springer Nature remains neutral with regard to jurisdictional claims in published maps and institutional affiliations.
Supplementary Information
Below is the link to the electronic supplementary material.
Rights and permissions
About this article
Cite this article
Folegot, S., Bruno, M.C., Larsen, S. et al. The effects of a sediment flushing on Alpine macroinvertebrate communities. Hydrobiologia 848, 3921–3941 (2021). https://doi.org/10.1007/s10750-021-04608-8
Received:
Revised:
Accepted:
Published:
Issue Date:
DOI: https://doi.org/10.1007/s10750-021-04608-8