Abstract
The root-knot nematode infestation is a significant challenge in chilli cultivation, necessitating the development of resistant cultivars. This study aimed to screen 200 chilli germplasm lines and identify resistant sources for root-knot nematode using multivariate analysis at ICAR-Indian Institute of Horticultural Research, Bangalore. The parameters such as shoot length, root length, shoot weight, root weight, shoot girth were assessed, and nematode infestation was quantified by counting galls and egg masses. The germplasm lines were categorized based on gall index and egg mass index into highly resistant, resistant, moderately resistant, moderately susceptible, susceptible, and highly susceptible groups. The analysis of variance revealed substantial variation among the germplasm lines. The Pearson correlation analysis revealed significant positive associations between nematode infestation and growth parameters. The cluster analysis identified three distinct groups of genotypes based on root knot nematode tolerance. The factor analysis 1 is associated with nematode infestation, and factor analysis 2 is related to growth parameters. The multi-trait ideotype genotype distance index analysis integrated multiple traits to identify top-performing genotypes. The germplasm lines EC771549-A, IHR3226 and IHR3575 showed high resistance, while Pusa Sadabahar was susceptible. The PCR-specific markers were used for validation of Me genes for root-knot nematode resistance. The simple sequence repeat (SSR) marker GPMS 171 and sequence characterized amplified region (SCAR) marker SCAR_CD showed clear polymorphism, correlating with phenotypic resistance. The germplasm line EC771549-A, is consistently resistant at phenotypic, molecular and selected based on multi-trait ideotype genotype distance index values, while Pusa Sadabahar was confirmed as susceptible. This study highlights the effectiveness of integrating molecular marker analysis with phenotypic evaluation and multivariate analysis to identify root-knot nematode resistant sources. The findings provide valuable insights into the genetic architecture of root-knot nematode resistance in chilli and candidate lines for breeding programme aimed at developing resistant cultivars, thereby contributing to sustainable chilli production and nematode management.
Similar content being viewed by others
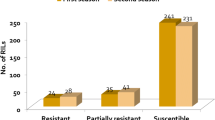
Avoid common mistakes on your manuscript.
Introduction
Chilli (Capsicum annuum L.), also known as hot pepper, is a crucial commercial vegetable and spice crop in India and a member of the Solanaceae family. It plays a significant role in culinary purposes. Capsicum genus is noted for its pungency and flavor, originated in the American tropics and was introduced to Southern India by the Portuguese, eventually spreading across the country by the late nineteenth century.
Root-knot nematodes (RKN) of the genus Meloidogyne are prevalent soil pests in warm climates or regions with mild winters (Williamson and Kumar 2006). This genus encompasses over 60 species that can infest around 2,000 species (Sanchez-Puerta and Masuelli 2011), among them four (M. javanica, M. arenaria, M. incognita, M. hapla) are significant global pests (Sanchez-Puerta and Masuelli 2011; Eisenbach and Triantaphyllou 1991; Thies and Fery 2002), with M. incognita race 2 being the most common in India (Cetintas and Yarba 2010). Controlling RKN is challenging due to their soil-borne nature and wide host range (Mitkowski and Abawi 2003). The chemical treatments, such as fumigants and nervous system toxins, are often used but pose risks to human health and the environment, making them less favourable (Fuller et al. 2008). Alternative methods, including soil solarization and crop rotation, have shown limited effectiveness against nematodes. Therefore, developing resistant chilli cultivars is regarded as the most effective control strategy.
In peppers several dominant genes that confer resistance to nematodes have been identified, demonstrating gene-for-gene interactions. Six heat-stable RKN resistance genes, termed as Me genes, have been discovered in various pepper cultivars which are located on chromosome nine. Notably, Me1, Me3, and Me7 provide resistance against multiple nematode species, each showing different levels of effectiveness depending on the species. Molecular markers have been developed to facilitate marker-assisted selection for these genes. Additionally, a unique RKN resistance gene from the "Mississippi Nemaheart" cultivar has been utilized to develop resistant lines such as Carolina Wonder and Charleston Bell. Allelism tests have confirmed that the N gene and Me3 are distinct, with the N gene located 7 cM from Me1 and 2 cM from Me3 on chromosome P9. Resistance is governed by nine genes such as N, Me1, Me2, Me3, Me4, Me5, Me7, Mech1, and Mech2 of which five genes such as Mech1, Mech2, Me1, Me3, and Me7 are mapped (Wang et al. 2009; Djian-Caporalino et al. 2007).
The multivariate analysis will help in the selection of ideal genotypes, techniques such as PCA and cluster analysis are commonly employed to visualize and reduce the dimensionality of multivariate datasets (Slonecki et al. 2023). PCA is particularly useful for identifying key traits essential for phenotypic characterization and provides a detailed understanding of the relationships between traits (Palumbo et al. 2019). However, the use of multiple principal components (PCs) can complicate the process of ranking genotypes based on trait values. Conversely, cluster analysis groups genotypes with similar traits to evaluate genetic diversity.
Usually, traditional ranking approaches are used to rank genotypes based on performance for single trait, which is often misleading, recently a new index such as multi-trait genotype ideotype distance index known as MGIDI which ranks genotypes based on multiple trait performance (Olivoto and Nardino 2021).
Identifying and validating molecular markers linked to resistance genes, such as the Me genes in chilli, significantly enhance the breeding process. These markers facilitate the accurate selection of resistant genotypes at the seedling stage, thereby considerably shortening the time needed to develop resistant cultivars. The Me genes confer resistance to various Meloidogyne species, making them crucial for marker-assisted selection (MAS) in chilli breeding programs.
This study aims to employ multivariate analysis to screen chilli genotypes for resistance to RKN and validate molecular markers associated with Me genes. By integrating phenotypic data with molecular marker information, the objective is to identify robust sources of resistance and improve the efficiency of breeding programme focused on developing RKN-resistant chilli cultivars.
Materials and methods
Plant materials
This study involved screening 200 chilli germplasm accessions for resistance to the root-knot nematode, M. incognita race 2. The accessions, maintained at the ICAR-Indian Institute of Horticultural Research (IIHR) in Bengaluru, were evaluated for RKN resistance under greenhouse conditions.
Experimental location and design
The greenhouse experiments were carried out at ICAR-IIHR, Bangalore, during February and April 2015. One-month-old seedlings of 200 C. annuum accessions were individually planted in 500 ml black nursery polybags containing a sterilized soil mixture composed of red soil, sand, and peat in a 1:1:1 ratio. Each germplasm was tested with five replications using a completely randomized design.
Collection and inoculation of nematodes
The eggs of M. incognita race 2 were extracted from infected C. annuum cv. Indra, plants maintained at the Nematology lab, ICAR-IIHR, Bangalore. The extraction process involved agitating the roots in a 0.05% NaOCl solution for 2 to 3 min (Hussey and Barker 1973). The collected eggs were rinsed with tap water using nested sieves with 150 and 25 μm pores. The second-stage juveniles (J2) emerging from the eggs were collected daily, and only those not older than five days were used for inoculation. The nematodes were introduced into three holes around the stem base, each approximately 2 cm deep.
Screening for root-knot nematode resistance
The chilli germplasms were evaluated for resistance against RKN using a greenhouse screening method described by Holbrook et al. (1983). One-month-old seedlings were transplanted into polybags and inoculated with 1,500 J2 of M. incognita two days post-planting. The plants were grown under greenhouse conditions at 27 ± 2 °C and regularly watered. After six weeks, the plants were carefully uprooted, and the roots were washed to remove soil particles. The plant growth parameters were measured, including shoot length (SL), root length (RL), shoot weight (SW), root weight (RW), shoot girth (SG), average number of galls (ANG), and average number of egg masses (ANEM). The gall index (GI), was assessed using a 0–5 scale based on Taylor and Sasser 1978 (Table 1). Similarly, the number of egg masses per root system was determined after staining the roots with 0.05% phloxine B solution, observed under a stereo zoom microscope (Motic SMZ 168 series). The egg mass index (EMI) was also scored on a 0–5 scale similar to the gall index.
To determine the nematode population density in the soil, methods described by Cobb (1918) and the modified Baermann funnel technique (Ravichandra 2010) were employed. These methods allowed for estimating the nematode population per 100 g of soil.
Identification of resistant germplasm sources using multivariate analysis
To ascertain the noteworthy impacts of different genetic types, an ANOVA was performed. Furthermore, various statistical methodologies were utilized to pinpoint chilli germplasm sources resistant to root-knot nematodes. These approaches encompassed correlation analysis, cluster analysis, and several multivariate techniques such as principal component PCA, factor analysis, and MGIDI analysis.
Pearson correlation coefficient and cluster analysis
We conducted Pearson correlation analysis to examine the relationships between growth parameters and tolerance to root-knot nematodes. Additionally, cluster analysis using Ward's method and Euclidean distance (Ward 1963) was employed to group germplasms based on their similarities in growth and nematode tolerance traits.
In the correlation analysis, we explored how variables such as growth parameters correlate with measures of nematode tolerance. This allowed us to identify which growth parameters are most closely associated with resistance or susceptibility to nematodes.
The cluster analysis, utilizing Ward's method and Euclidean distance, enabled us to classify chilli germplasms into distinct groups according to their combined characteristics related to growth and nematode tolerance. This methodological approach helps in identifying clusters of germplasms that exhibit similar patterns of growth and nematode resistance, thereby suggesting potential sources of resistance for further study or use in breeding programme.
Principal component analysis (PCA)
The PCA was conducted to assess both growth parameters and resistance to root knot nematodes. This statistical technique helps in reducing the dimensionality of the data while preserving as much variance as possible, thereby revealing underlying patterns and correlations among variables.
In PCA, the original variables are transformed into a new set of orthogonal (uncorrelated) variables called principal components. These components are ordered by the amount of variance they explain in the data. By examining these components and their loadings (correlations between original variables and the components), we can identify which variables contribute the most to the observed patterns in the dataset.
Factor analysis
Initially, loadings were determined by focusing on factors with eigenvalues exceeding one. Subsequently, an analytic rotation using the varimax criteria (Kaiser 1958) was employed to refine and estimate the final loadings. Significant traits were then assessed to calculate heritability and selection gain using the below mentioned formulas.
whereas \({h}^{2}bs\) = broad sense heritability; \({\sigma }_{g}^{2}\) = genotypic variance; \({\sigma }_{p}^{2}\) = phenotypic variance
\({\overline{X} }_{s}\) = mean of selected genotypes; \({\overline{X} }_{o}\)= mean of all the genotypes; h2bs = broad sense heritability; SG = selection gain.
Multi-trait genotype ideotype distance index (MGIDI) analysis
The MGIDI was calculated done using Euclidean distance scores (Olivoto and Lucio, 2020), the following formula was used to calculate the MGIDI values of each genotype.
Validation of molecular markers linked to RKN resistance Me genes
The two sequence characterized amplified region (SCAR) markers along with two SSCP and one CAPS marker associated with Me genes were validated through linked PCR assays. Table 2 presents the specific PCR primers used for the validation of Me genes for RKN resistance.
DNA extraction
The fresh leaf tissues of C. annuum germplasms were used to extract DNA using the CTAB method (Doyle and Doyle 1987). The DNA quantification was carried out with a Nanodrop ND-1000 spectrophotometer.
SCAR analysis
Two SCAR markers, SCAR_B94 and SCAR_CD, which are associated with Me3–Me4 and Me7–Mech1 resistance genes respectively (Tai et al. 1999; Djian-Caporalino et al. 2007), were employed to validate root-knot nematode resistance. The DNA amplification was conducted in a 15 μL reaction mixture comprising 2 μL of 10X PCR buffer (50 mM KCl, 10 mM Tris–HCl, 1.5 mM MgCl2, pH 8.3), 2 μL of MgCl2, 0.5 μL of dNTPs (0.2 mM), 1.5 μL each of forward and reverse primers (10 pmol), 0.25 μL of Taq polymerase (Thermo Scientific EP0401), 15.75 μL of sterile distilled water, and 2 μL of DNA (~ 55 ng/μL).
The PCR amplification was carried out in thermocyclers Mastercycler epgradient (Eppendorf), The PCR programme consisted of an initial denaturation step of 3 min at 94 °C, followed by 30 cycles of denaturation at 94 °C for 30 s, with annealing temperature for each primer as mentioned in Table 3 for 30 s, extension at 72 °C for 1 min, and a final extension step of 5 min at 72 °C.
The PCR products were separated on 3% agarose (Himedia MB080) gels in 1X TAE buffer, stained with ethidium bromide along with 100 bp DNA ladder (Himedia MBT130-50LN) and documented using BioRad Gel Doc XR Gel Documentation System.
Simple sequence repeat (SSR) analysis
The eight SSR primers were employed to validate resistance against root-knot nematodes. Table 3 provides details of the SSR primers used for marker validation, including their melting temperature (Tm) and specific annealing temperatures. The amplification of DNA was done in a 15 μL reaction mixture composed of 2 μL of 10X PCR buffer, 2 μL of dNTPs (0.2 mM), 1.5 μL each of forward and reverse primers, 0.5 μL of Taq polymerase (Thermo Scientific EP0401), 5.5 μL of sterile distilled water, and 2 μL of DNA (~ 55 ng/μL).
The PCR protocol was executed in a thermocycler Mastercycler epgradient (Eppendorf), with an initial denaturation step at 94 °C for 5 min (3 min for SSCP primers), followed by 35 cycles of denaturation at 94 °C for 30 s, annealing at the specific temperature for each primer pair for 30 s, extension at 72 °C for 1 min, and a final extension step at 72 °C for 5 min. Following amplification, The PCR products were separated on 3% agarose (Himedia MB080) gels in 1X TAE buffer, stained with ethidium bromide along with 100 bp DNA ladder (Himedia MBT130-50LN) and documented using BioRad Gel Doc XR Gel Documentation System. The polymorphic markers identified through this process were subsequently utilized across the entire population.
Data analysis
The data analysis was conducted using the R statistical software, specifically version 4.3.2, and employing the metan package as described by Olivoto 2019.
Results
Analysis of variance
Table 4 presents the results of the analysis of variance for growth parameters and RKN incidence in chilli germplasm. The variables SL, RL, SW, RW, SG, ANG, ANEM, GI, and EMI showed high significance (p < 0.01) level of variation. Genotype was identified as the primary source of variance, with replication also contributing significantly. This underscores that the observed variations in RKN incidence are largely influenced by genotype, indicating distinct differences among genotypes in their susceptibility to RKN.
Screening for root-knot nematode resistance
The evaluation of 200 chilli germplasm lines for resistance to root-knot nematodes involved assessing multiple parameters including SL, RL, SW, RW, SG, ANG and ANEM. From each germplasm line, three plants were randomly selected, uprooted, washed, and quantified for average gall and egg mass counts. The severity of gall formation was rated using Taylor and Sasser's scale, and egg masses were enumerated following staining with phloxin B solution, enhancing the assessment of nematode infestation severity.
Based on the GI and EMI the genotypes were categorized into various resistance/susceptibility groups. The summarized response of the germplasm lines against root-knot nematodes is detailed in Table 5.
This classification provided a spectrum of responses, ranging from immune reactions to differing degrees of susceptibility. Notably, 21 lines demonstrated high resistance to root-knot nematode infestation, while 13 lines exhibited resistance. Additionally, 39 lines showed moderate resistance, indicating they possess some capacity to tolerate nematode infestation. Conversely, 49 lines were moderately susceptible, 65 lines were susceptible, and 12 lines were highly susceptible, underscoring the diverse susceptibility profiles observed within the germplasm collection.
Identification of resistant germplasm sources using multivariate analysis
Pearson correlation coefficient
Figure 1 depicts the results of Pearson’s correlation analysis among growth parameters, average number of galls, and average number of egg masses. The analysis shows that the AVG, ANEM, GI and EMI exhibit highly significant and positive correlations with each other. On the other hand, RW, SL, SW, and SG display highly significant and positive associations among themselves. Interestingly, there was no significant correlation observed between the growth parameters (RW, SL, SG, SW) and the number of galls or egg masses. This indicates that while certain growth parameters are closely interrelated, their relationship with nematode infestation indicators such as galls and egg masses is not significant in this analysis.
Cluster analysis
Figure 2 displays a dendrogram resulting from cluster analysis, which categorizes 200 chilli germplasm lines into three main clusters. Cluster I comprise genotypes categorized as tolerant to root-knot nematodes namely G3, G6, G37, G39, G40, G48, G59, G63, G101, G103, G104, G110, G129, G146, G150, G161, G162, G167, G177, G181, and G187. Cluster III encompasses germplasm lines considered susceptible or showing varying levels of susceptibility to nematodes, such as G67, G68, G69, G70, G72, G73, G84, G92, G97, G119, G138, and G192. Cluster II contains germplasm lines that exhibit moderate resistance or susceptibility to RKN.
Principal component analysis
PCA analysis revealed two principal components, PC1 and PC2, with eigenvalues of 3.71 and 3.04, respectively, as detailed in Table 6. Together, these components account for a cumulative variation of 75.1% of the total variance. The principal component matrix (eigenvectors) provided in Table 7 indicates that the first component (PC1) predominantly includes ANG, ANEM, GI and EMI. On the other hand, the second principal component (PC2) primarily consists of SL, RL, SW, RW and SG.
Factor analysis
All variables showed significant genotype effects at the 0.05 level based on P values from the likelihood ratio test. The factor analysis with rotation identified two factors, FA1 and FA2 (Table 8). The FA1 includes ANG, ANEM, GI, and EMI, while FA2 includes SL, RL, SW, RW, and SG. The average communality was 76.66%, with uniqueness reported at 24.33%. The parameters SW, ANG, ANEM, GI, and EMI exhibited higher communality values compared to the average.
The mean of selected genotypes was higher than the mean of all genotypes across all traits. The parameters SL, RL, and RW showed higher uniqueness values as compared to the average uniqueness. The high selection differential percentages and selection gains were observed for GI, SG, and RL. The broad-sense heritability was high for all variables, indicating substantial genetic influence. The sense was indicated an increase across all parameters.
Selection of germplasm lines using MGIDI
Figure 3 illustrates the results of the MGIDI analysis, which identified specific germplasm lines as selected for their favourable performance for growth parameters. These selected genotypes include G33, G68, G93, G134, G15, G16, G9, G67, G8, G96, G135, G1, G95, G62, G12, G7, G13, G89, G5, G137, G44, G18, G184, G139, G71, G36, G10, G65, G54, and G196.
Figure 4 depicts a chart detailing the strengths and weaknesses of selected genotypes in predicting their contribution to overall genotype performance. According to the chart, FA1 shows the highest contribution in the performance of genotype G67, followed by G68, G13, G71, G95, G44, G65, and G196. On the other hand, FA2 exhibits the highest contribution in the performance of genotype G7, followed by G9, G33, G5, G184, G8, and G10.
FA1 primarily encompasses traits related to root-knot nematode screening, while FA2 consists of growth parameters. This categorization suggests that certain genotypes excel in nematode resistance traits (FA1) or growth-related traits (FA2), highlighting their respective strengths in contributing to the performance of genotypes.
Selection of root knot nematode resistant germplasm sources using MGIDI
Figure 5 illustrates the results of the MGIDI analysis, which identifies specific germplasm lines selected for their resistance to root knot nematodes. These selected genotypes include G101, G103, G104, G110, G129, G146, G150, G161, G162, G167, G178, G182, G188, G3, G37, G39, G40, G48, G59, G6, G63, G105, G149, G198, G66, G122, G174, G186, G21, and G52. These germplasm lines are noted for their potential resistance traits against root knot nematodes, highlighting their suitability for breeding programme for developing nematode-resistant cultivars.
Validation of molecular marker linked to Me genes
A total of 11 eleven genotypes viz., G1, G2, G3, G4, G6, G39, G67, G92, G101, G111 and G193 were selected for validation of molecular markers linked to Me genes. Among the SSR primers used, the primer GPMS 171 (Fig. 6), showed polymorphic bands. The germplasm lines G1, G2, G3, G4, G6, G92, G111 and G193 were identified as resistant, whereas germplasm lines G39, G67, and G101 were found to be susceptible. The two SCAR markers were applied to detect polymorphism. Multiple bands were observed for SCAR_B94, indicating potential complexity or multiple alleles, whereas SCAR_CD (Fig. 7) showed clear polymorphism. The germplasm lines G3, G4, G39, and G101 were identified as resistant to the markers tested, while germplasm lines G1, G2, and G67 were susceptible. These results highlight the genetic variability among the tested germplasm lines concerning resistance to the markers assessed, emphasizing specific lines as either resistant or susceptible based on the polymorphism observed.
Comparative analysis of phenotypic, molecular and MGIDI analysis for identification of RKN resistant genotypes
Table 9 provides a comparative analysis of SSR primer GPMS171, SCAR marker SCAR_CD, phenotypic data, and MGIDI analysis for various genotypes. It indicates that genotype EC771549-A exhibits resistance at molecular, phenotypic level and as per MIGIDI analysis, while genotype Pusa Sadabahar is susceptible according to both molecular, phenotypic level and as per MIGIDI analysis. For genotypes EC378688, EC39108, EC378632, IHR-3226, (Ms 12 × Az) X Ms3, IHR-9498, IHR3575, EC769394, and IHR3626, further confirmation is required to determine their resistance or susceptibility reactions. These genotypes show ambiguous or inconclusive results based on the current analysis, necessitating additional investigation to clarify their response to the markers and phenotypic traits under study.
Discussion
The comprehensive screening of 200 chilli germplasm lines for root-knot nematode resistance has revealed significant insights into the genetic variability and resistance mechanisms within the collection. Utilizing a multifaceted approach that included ANOVA, Pearson correlation coefficients, cluster analysis, PCA, factor analysis, and MGIDI analysis has enabled a robust evaluation and categorization of the germplasm lines based on their response to RKN infestation.
The ANOVA results underscored significant genotype effects on both growth parameters and nematode infestation levels, highlighting substantial genetic variation among the chilli germplasm lines. This genetic diversity is crucial for breeding programme aimed at enhancing RKN resistance, as it provides a diverse pool of genetic resources from which to select and develop resistant cultivars. The categorization of germplasm lines into highly resistant, resistant, moderately resistant, moderately susceptible, susceptible, and highly susceptible groups based on GI and EMI illustrated a broad spectrum of responses. Notably, 21 lines were identified as highly resistant, making them prime candidates for breeding programme focused on RKN resistance. 13 lines exhibited resistance, while 39 lines showed moderate resistance, indicating partial resistance that can be improved through further breeding programme. The identification of 49 moderately susceptible, 65 susceptible, and 12 highly susceptible lines highlights the range of susceptibility within the germplasm, which is essential for understanding the genetic basis of resistance and susceptibility. These findings align with previous research conducted by Pandravada et al. (2010) in chilli.
The correlation analysis revealed significant positive associations among the number of galls, egg masses, GI, and EMI, suggesting that higher nematode infestation leads to increased gall formation and egg mass production. Additionally, strong positive correlations among root and shoot growth parameters indicate the interdependence of these traits in chilli plants. These findings are critical for selecting genotypes that balance both growth and resistance traits.
The cluster analysis identified three distinct clusters based on RKN tolerance levels, providing valuable insights for breeders in selecting appropriate parental lines. Cluster I comprised genotypes exhibiting tolerance to RKN, Cluster II included genotypes with moderate susceptibility or tolerance, and Cluster III consisted of susceptible genotypes. This classification aids in targeted breeding strategies by identifying genotypes with desirable resistance traits. PCA revealed two principal components explaining a significant proportion of variance in RKN screening traits. These components serve as useful indicators for summarizing the complex relationships among the studied variables. Factor analysis further identified two factors (FA1 and FA2) influencing RKN resistance and growth parameters. FA1 is primarily encompassed traits related to nematode infestation, while FA2 is included growth-related traits, offering a comprehensive understanding of the underlying factors influencing RKN resistance and growth in chilli.
The MGIDI analysis refined the selection of promising germplasm lines based on their performance in growth parameters and RKN resistance, identifying specific genotypes with strengths and weaknesses in their overall performance, aiding in the identification of superior lines for further breeding programmes.
Notably, the SSR primer GPMS 171 and SCAR marker SCAR_CD showed clear polymorphism, enabling the differentiation of resistant and susceptible germplasm lines. The integration of molecular marker analysis with phenotypic evaluation provides a powerful approach for validating RKN resistance in chilli germplasm. The use of eight SSR primers and two SCAR markers enabled the assessment of polymorphism and the correlation of molecular markers with phenotypic resistance or susceptibility. The alignment between molecular marker data and phenotypic evaluation validates the utility of these markers in predicting RKN resistance. These findings are consistent with previous reports in chilli by Ozturk (2012) and in tomato by Palanisamy et al. (2023).
The consistency observed between molecular and phenotypic assessments underscores the reliability of markers such as GPMS 171 and SCAR_CD for RKN resistance. The germplasm line EC771549-A exhibited resistance at both molecular and phenotypic levels and was also selected as resistant by MGIDI, corroborating its status as a truly resistant line. Conversely, the genotype Pusa Sadabahar demonstrated susceptibility at both phenotypic and molecular levels highlighting the accuracy of the markers used.
The successful identification and validation of root-knot nematode resistance in chili genotypes through both traditional screening and advanced multivariate approaches highlight a significant breakthrough in chilli breeding. The germplasm line EC771549-A, consistently demonstrating resistance, has been confirmed through linked molecular markers such as SSR, CAPS, and SCAR. This dual validation emphasizes the reliability of the selected genotypes and underscores the effectiveness of combining phenotypic and molecular methods in breeding programme. The germplasm line EC771549-A can be utilized in breeding programme to develop RKN-resistant chilli cultivars, which would contribute to more sustainable and productive chilli cultivation.
Conclusion
This study underscores the significance of integrating phenotypic evaluation with molecular marker analysis to identify and validate RKN resistance in chilli germplasm. A significant genetic variation was observed among the 200 chilli germplasm lines, emphasizing the importance of genotype selection for the RKN resistance breeding programme. The SSR marker GPMS 171 and the SCAR marker SCAR_CD were effective in distinguishing RKN-resistant and susceptible lines, showing strong concordance with phenotypic data. The germplasm line EC771549-A, is confirmed as resistant both phenotypically and molecularly and it has been selected as resistant by MGIDI, while Pusa Sadabahar was consistently susceptible. The cluster and PCA analyses provided a clear classification of genotypes based on RKN tolerance levels, aiding in the selection of superior breeding lines.
The identified markers and resistant germplasm lines offer valuable resources for developing RKN-resistant chilli cultivars, contributing to sustainable chilli production and nematode management. Overall, the integration of multivariate analyses and molecular marker validation presents a robust framework for enhancing RKN resistance in chilli breeding programme.
Data Availability
The datasets underpinning the findings presented in this article are accessible upon request. Please feel free to reach out to the corresponding author for further information regarding data availability and access procedures.
References
Cetintas R, Yarba MM (2010) Nematicidal effect of five plant essential oils on the southern root-knot nematode, M incognita race 2. J Anim Vet Adv 9(2):222–225
Cobb (1918) Estimating the nema populations of soil. USDA Technical Circular 1, pp. 48.
Djian-Caporalino C, Fazari A, Arguel MJ et al (2007) Root-knot nematode (Meloidogyne spp.) Me resistance genes in pepper (Capsicum annuum L.) are clustered on the P9 chromosome. Theor App Genet 114:473–486
Doyle JJ, Doyle JL (1987) A rapid DNA isolation procedure for small quantities of fresh leaf tissue. Phytochem Bull 19(1):11–15
Eisenbach JD, Triantaphyllou HH (1991) Root-knot nematodes Meloidogyne species and races. In: Nickle WilliamR (ed) Manual of agricultural nematology. Marcel Dekker, INC., New York, pp 281–286
Fuller VL, Lilley CJ, Urwin PE (2008) Nematode resistance. New Phytol 180:27–44
Holbrook CC, Knauft DA, Dickson DW (1983) A technique for screening peanut for resistance to Meloidogyne arenaria. Plant Dis 67:957–958
Hussey RS, Barker KR (1973) A comparison of methods of collecting inoculum for Meloidogyne spp., including a new technique. Plant Dis Rep 57:1025–1028
Kaiser HF (1958) The varimax criterion for analytic rotation in factor analysis. Psychometrika 23:187–200. https://doi.org/10.1007/BF02289233
Mitkowski NA, Abawi GS (2003) Root-knot nematode. Plant Health Instr. https://doi.org/10.1094/PHI-I-2003-0917-01
Olivoto T, Lúcio AD (2020) metan: An R package for multi-environment trial analysis. Methods Ecol Evol 11:783–789. https://doi.org/10.1111/2041-210X.13384
Olivoto T, Nardino M (2021) MGIDI: toward an effective multivariate selection in biological experiments. Bioinformatics 37:1383–1389. https://doi.org/10.1093/bioinformatics/btaa981
Olivoto T (2019) Metan: multi environment trials analysis. R Package Version 1.1.0. https://github.com/TiagoOlivoto/metan.
Ozturk L (2012) Development of molecular markers linked to Me1 gene conferring resistance to nematode in pepper. M.Sc Thesis. Graduate School of Engineering and Sciences of İzmir Institute of Technology.
Palanisamy M, Jat GS, Kalia P et al (2023) Screening and validation of Ty-1, Ty-3 and Ty-3a specific markers in Solanum chilense accessions for resistance to tomato leaf curl virus. Genet Resour Crop Evol 70:2567–2574. https://doi.org/10.1007/s10722-023-01586-9
Palumbo F, Galvao AC, Nicoletto C, Sambo P, Barcaccia G (2019) Diversity analysis of sweet potato genetic resources using morphological and qualitative traits and molecular markers. Genes 10(840):1–21. https://doi.org/10.3390/genes10110840
Pandravada SR, Varaprasad KS, Reddy KJ, Rao ES (2010) Screening and identification of sources of resistance against root-knot nematode (Meloidogyne javanica) in chilli (Capsicum annuum) germplasm. Indian J Agric Sci 80(1):92–94
Ravichandra NG (2010) Methods and techniques in plant nematology. PHI Learning Pvt Ltd New Delhi, India, p 595
Sanchez-Puerta MV, Masuelli RW (2011) Evolution of nematode-resistant Mi-1 gene homologs in three species of Solanum. Mol Genet Genom 285:207–218
Slonecki TJ, Rutter WB, Olukolu BA, Yencho GC, Jackson DM, Wadl PA (2023) Genetic diversity, population structure, and selection of breeder germplasm subsets from the USDA sweetpotato (Ipomoea batatas) collection. Front Plant Sci 13:1–15. https://doi.org/10.3389/fpls.2022.1022555
Tai T, Dahlbeck D, Stall RE, Peleman J, Staskawicz BJ (1999) High-resolution genetic and physical mapping of the region containing the Bs2 resistance gene of pepper. Theor App Genet 99:1201–1206
Taylor AL, Sasser JN (1978) Biology, identification and control of root-knot nematodes (Meloidogyne species). A cooperative publication of the Department of Plant Pathology, North Carolina State University, Raleigh, NC and the USAID, p 111
Thies JA, Fery RL (2002) Evaluation of a core of the US Capsicum germplasm collection for reaction to the northern root knot nematode. Hort Sci 37(5):805–810
Wang LH, Gu XH, Hua MY et al (2009) A SCAR marker linked to the N gene for resistance to root knot nematodes (Meloidogyne spp.) in pepper (Capsicum annuum L.). Sci Hortic 122:318–322
Ward JH (1963) Hierarchical Grouping to optimize an objective function. J Am Stat Assoc 58:236–244. https://doi.org/10.1080/01621459.1963.10500845
Williamson VM, Kumar A (2006) Nematode resistance in plants: the battle underground. Trends Geneti 22(7):396–403
Acknowledgements
I acknowledge the invaluable support received from Dr. Krishna Reddy in conducting this research. I extend my gratitude to all the staff of the Division of Plant Protection and Nematology at ICAR-IIHR for their assistance and cooperation throughout the study. Their expertise and dedication have been instrumental in the successful completion of this work.
Funding
The investigation detailed in this paper constitutes a vital component of the professional attachment training at ICAR-IIHR, Bangalore. No funding has been received during the preparation of this manuscript.
Author information
Authors and Affiliations
Contributions
KHG and KMR have designed the experiment, performed the experiments, carried out statistical analysis and wrote the manuscript. UM has designed the experiment, and performed the experiments. PP, VH and RA have assisted in the formal analysis, review and editing of the manuscript and all authors have read and approved the manuscript for publication.
Corresponding authors
Ethics declarations
Conflict of interest
The authors declare that there is no conflict of interest regarding the publication of this manuscript.
Additional information
Publisher's Note
Springer Nature remains neutral with regard to jurisdictional claims in published maps and institutional affiliations.
Rights and permissions
Springer Nature or its licensor (e.g. a society or other partner) holds exclusive rights to this article under a publishing agreement with the author(s) or other rightsholder(s); author self-archiving of the accepted manuscript version of this article is solely governed by the terms of such publishing agreement and applicable law.
About this article
Cite this article
Hanume Gowda, K., Madhavi Reddy, K., Maheshwari, U. et al. Screening, identification of root-knot nematode resistance sources using multivariate analysis and validation of molecular markers linked to Me genes in chilli (Capsicum annuum L.). Genet Resour Crop Evol (2024). https://doi.org/10.1007/s10722-024-02124-x
Received:
Accepted:
Published:
DOI: https://doi.org/10.1007/s10722-024-02124-x