Abstract
Rapid industrialization results in the production of large quantities of waste that are commonly discharged into water bodies, leading to the damage of the aquatic ecosystem and freshwater organisms. Copper (Cu) can induce oxidative damage in fish muscle, the main fish portion that is consumed by humans. However, the responses of the Amazon fish Cichlasoma amazonarum and its capacity to withstand acute Cu concentrations found in Amazon water around mines remain unknown. Thus, the aim of this study was to evaluate whether exposure to Cu causes muscle oxidative stress and/or oxidative damage and impairs the fillet fatty acid profile of C. amazonarum acutely exposed to Cu found in Amazon waters around mines. Muscle reactive oxygen species and protein carbonylation levels were significantly higher in fish exposed to 1500 μg/L Cu compared with the control group, while muscle lipid peroxidation levels were significantly higher in fish exposed to 500, 750, and 1500 μg/L Cu compared with control group. Muscle antioxidant capacity against peroxyl radical’s levels and glutathione peroxidase activity were significantly lower in fish exposed to 1500 μg/L Cu compared with the control group, while muscle superoxide dismutase activity was significantly lower in fish exposed to 750 and 1500 μg/L Cu compared with control group. The total content of saturated fatty acids was significantly higher in fish exposed to 1500 μg/L Cu compared with the control group, while the total content of monounsaturated fatty acids and sum of n3 fatty acids were significantly lower in fish exposed to 1500 μg/L Cu compared with control group. No significant difference was observed regarding muscle catalase, glutathione S-transferase, and glutathione reductase activities. Based on these lines of evidence, the results of this comprehensive study agree with the initial hypothesis that the exposure to Cu found in Amazon water around mines induces oxidative damage and inhibits enzymatic and non-enzymatic antioxidant response in the muscle of C. amazonarum exposed to high Cu levels. Moreover, the impairment of the fillet fatty acid profile appears to be mediated by oxidative damage, representing a negative impact on fish health.
Similar content being viewed by others
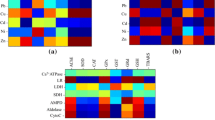
Explore related subjects
Discover the latest articles, news and stories from top researchers in related subjects.Avoid common mistakes on your manuscript.
Introduction
Metal pollution is largely recognized as a serious threat to the aquatic ecosystem due to the complex sources, persistent and toxic behavior, and bio-accumulation properties (He et al. 2019). Large quantities of metals have been released into aquatic environments worldwide primarily by anthropogenic sources (such as industrial drainages, mining, vehicle emissions, agricultural runoff, sewage disposal, and dam construction) and by natural sources (erosion and weathering of rocks) (Jafari et al. 2018; Hader et al. 2020; Fry et al. 2020), making metals a group of contaminants with high ecological importance due to their serious risk for animal and human health through direct ingestion, dermal contact, and food intake (Zhang et al. 2018).
Copper (Cu) is an abundant metal that occurs as a natural element due to weathering of rocks and volcano eruptions. It is used across the world in many industrial applications (ore refining, electroplating process, applications of fertilizers and pesticides) and consumer products, and consequently, it has been extracted in very high quantities (Siqueira-Gay et al. 2020). This extraction may cause elevation of waterborne Cu concentration and contamination of the aquatic ecosystem (Fry et al. 2020), which generates concerns due to its deleterious effects on the aquatic ecosystem when present in higher levels. Although physiologically essential, waterborne Cu at elevated concentrations can be toxic to fish, causing serious cellular injuries mediated principally by oxidative stress and oxidative damage (Abou Anni et al. 2019; Tesser et al. 2020). Oxidative stress is a reversible alteration of redox status in cell compartments. Oxidative stress can damage cellular molecules (via lipid peroxidation and protein carbonylation), and in so doing, can serve as a cellular signal (Viña et al. 2018). When the redox condition of the cell modulates to a more reduced state, there is an irreversible (i.e., non-physiological) process known as oxidative damage. In other words, oxidative stress precedes oxidative damage that occurs when reducing systems can no longer accommodate the rapid rate of cell component oxidation (Viña et al. 2018). To reduce or avoid the oxidative damage caused by excessive reactive oxygen species (ROS) generation, the antioxidant defense system (enzymatic or non-enzymatic) can be activated as a survival strategy to eliminate ROS overproduced during exposure to waterborne Cu (Boareto et al. 2018; Shekh et al. 2020). In this sense, the first line of antioxidant defense includes the enzymes superoxide dismutase (SOD) and catalase (CAT), while the second line of the antioxidant system includes glutathione peroxidase (GPx), glutathione reductase (GR), and glutathione S-transferase (GST), as well as non-enzymatic mechanisms that maintain the balance of oxidation-reduction reactions and consequently protection against oxidative damage (Sies et al. 2017). On the other hand, the antioxidant defense system can also be inhibited by Cu levels, which contribute to the intensification of Cu toxicity and fish mortality, as observed by Tesser et al. (2020) for curimbatá (Prochilodus lineatus) and Zebral et al. (2019) for killifish (Poecilia vivipara). To the best of our knowledge, the responses of Cichlasoma amazonarum and its capacity to withstand acute Cu exposure in terms of oxidative stress are unknown. Our hypothesis is that the muscle antioxidant system of C. amazonarum would be unable to cope with acute Cu exposure levels found in Amazon waters around mines.
Fish is considered a healthy and important dietary resource for many populations worldwide. It is a rich source of health-friendly fatty acids, especially omega-3 (n3) and omega-6 (n6) polyunsaturated fatty acids (PUFA), as well as docosahexaenoic (DHA) and eicosapentaenoic (EPA) acids (Mohanty et al. 2019). Beyond the importance for human nutrition, fatty acids are the fundamental structural components of almost all forms of lipids, acting as precursors of bioactive molecules, and have structural and functional role lined to fish reproduction, osmoregulation, growth, and promoting fat-soluble vitamin absorption and transportation in the body (Sissener et al. 2020). However, due to the capability of fatty acids to accumulate in edible tissues, they might have undesirable effects on quality and freshness of fish flesh due to increase on total content of fats that augments the risk of rancidness, and be responsible for metal toxicity-related health risks in humans during consumption of fish (Das et al. 2018; Afridi et al. 2019). Despite muscle not being an active tissue in accumulating metals compared with other tissues, it is commonly analyzed because it is the main fish part consumed by humans, and although C. amazonarum is not commonly consumed by the human population, this species can provide a perspective and projection about meat quality of Amazon fishes linked to fatty acid profile during waterborne Cu exposure. Also, C. amazonarum is a fish species that develops a role in the food chain in the aquatic ecosystem that it is consumed by other big fishes, as pirarucu (Arapaima gigas), one of the most consumed fishes by Amazonian population (Hrbek et al. 2005); i.e., the Cu levels present in C. amazonarum can be deposited in pirarucu and consequently affecting humans. A recent study conducted by Das et al. (2018) revealed that long-term metal exposure (lead, Cu, cadmium, nickel, and zinc) alters the fatty acid composition of Indian major carps (Labeo rohita, Catla catla, and Cirrhinus cirrhosus) compromising fillet quality and revealing a direct relationship between oxidative damage/impairment on antioxidant defense system and changes of muscle fatty acid profile. Thus, our hypothesis is that acute exposure to Cu can affect the muscle fatty acid profile of C. amazonarum, and that oxidative damage can be involved in this process.
Based on these lines of evidence, the aim of this study was to evaluate whether exposure to Cu causes muscle oxidative stress and/or oxidative damage and impairs the fillet fatty acid profile of C. amazonarum acutely exposed to Cu found in Amazon waters around mines.
Material and methods
Chemical
Copper sulfate (CuSO4; 99% chemical purity; molecular weight 159.609 g/mol) was purchased from Sigma-Aldrich (St. Louis, MO, USA). A Cu stock solution (dissolved in water) was prepared from CuSO4 and spiked into the exposure waters 24 h before test, to allow equilibration.
Fish maintenance and experimental design
Cichlasoma amazonarum adults were bought from a fish farm (Amazonas, Brazil) and transferred to the Laboratory of Ecophysiology and Molecular Evolution (Amazonas, Brazil). A period of 30 days of acclimation was used to recover from stress and to acclimatize to laboratory conditions in continuously aerated 3000-L tanks with running water, being fed daily until satiation with commercial pelleted food (36% protein). Feeding was suspended 24 h prior to experiments.
A total of 108 juvenile C. amazonarum (mean weight, 20.94 ± 4.01 g; mean length, 8.14 ± 1.09 cm) were randomly transferred into eighteen 30-L glass aquaria and divided into six groups (n = 6 in each group, in triplicate), with increasing nominal Cu levels, as follows: 0.0 (control), 25, 250, 500, 750, and 1500 μg/L, for 96 h. Fish were fasted over the course of the test, mortality was recorded every day, and dead fish were removed. The Cu nominal levels used in this study were based on the environmental concentrations of Cu found in the Amazon water around mines (Santana and Barroncas, 2007), as well as in a curve of Cu concentrations used for other Amazon fishes, as cardinal tetra (Paracheirodon axelrodi) (Crémazy et al. 2016) and C. amazonarum (Baldissera et al., 2020). The water quality variables were evaluated daily and remained within acceptable limits throughout the experimental period, as follows: temperature (28.7 ± 0.4 °C), dissolved oxygen (6.3 ± 0.3 mg/L), and pH (5.7 ± 0.3). The Ethics Committee of INPA authorized all experimental procedures under the number 004/2018.
Water was collected in order to quantify the total Cu content on 0 h (beginning of the experiment), being determined by atomic absorption spectrometry using graphite furnace technique (Perkin-Elmer AAnalyst 800 AA spectrophotometer, Norwalk, CT, USA), as described in detail by Crémazy et al. (2016). The Cu concentrations in water of each group in the analyzed moments are represented in Table 1. Waterborne Cu levels of the control group was explained by Cu content on laboratory water, that is, 17.2 ± 1.6 μg/L.
Muscle collection
After 96 h Cu exposure, the muscle of two juveniles from each tank (n = 6 per treatment) was collected under anesthesia with tricaine methanesulfonate (MS-222) at 1.5 g/L followed by spinal cord sectioning.
Muscle oxidative stress-related parameters
A glass Potter homogenizer was used to homogenize muscle tissue with 10 mM Tris-HCl buffer pH 7.4. Thereafter, the homogenate was centrifuged at 2000g for 10 min, and the supernatants were collected and stored at − 20 °C until analyses. The techniques proposed by LeBel et al. (1992), Monserrat et al. (2003), and Reznick and Packer (1994) were used to determine muscle ROS, lipid peroxidation (LPO), and carbonyl protein levels, respectively. The results were expressed as U DCF/mg of protein, μmol CHP/g of tissue, and nmol of carbonyls formed/mg of protein, respectively.
The methods proposed by Amado et al. (2009), Marklund and Marklund (1974), Aebi (1984), Paglia and Valentine (1967), Habig et al. (1974), and Carlberg and Mannervik (1985) were used to measure muscle antioxidant capacity against peroxyl radicals (ACAP) levels, as well as SOD, CAT, GPx, GR, and GST activities, respectively. The results were presented as follows: fluorescence units/mg of protein, as U SOD/mg of protein, μmol CAT/mg of protein, nmol NADPH/h/mg of protein, μmol GS-DNB/min/mg of protein, and U GR/mg of protein, respectively.
Protein content was evaluated by the Coomassie blue G dye method using bovine serum albumin as the standard (Read and Northcote 1981).
Muscle fatty acid profile
The total lipids were extracted from muscle samples according to Bligh and Dyer (1959) method, and the lipids were transesterified/esterified to fatty acid methyl esters (FAME) as proposed by Hartman and Lago (1973), as described in detail by Baldissera et al. (2020). Results were expressed as a percentage of the total area of the chromatograms.
Muscle Cu levels
Muscle samples were decomposed with 2.0 mL 65% nitric acid (HNO3), as previously reported by Baldissera et al. (2020). The Cu levels were determined using an inductively coupled plasma mass spectrometer (ICP-MS) (Elan DCR II, Perkin-Elmer, Canada) equipped with a concentric nebulizer (Meinhard, USA), a cyclonic spray chamber (Glass Expansion, Inc., Australia), and a quartz torch with a quartz injector tube (2 mm diameter). The operational parameters to determine Cu levels via ICP-MS were as follows: radiofrequency power and plasma (1300 W), main flow rate (15 L/min), auxiliary flow rate (1.2 L/min), nebulizer flow rate (1.04 L/min), sampling cone and skimmer (Pt), and element monitored (65Cu).
Statistical analyses
Normality and homogeneity of variances for all data were tested by the Shapiro–Wilk’s and Levene’s tests, respectively. Data obtained were compared using a one-way analysis of variance (ANOVA) followed by the Tukey post hoc analysis. Significance was set at p < 0.05, and data were presented as mean ± standard error.
Results
Mortality
No mortality was observed after 24, 48, and 72 h of exposure to different Cu levels. On the other hand, 1 fish exposed to 750 μg Cu/L and 2 fish exposed to 1500 μg Cu/L water died after 96 h exposure.
Muscle oxidative stress-related parameters
Muscle ROS and protein carbonylation levels were significantly higher in fish exposed to 1500 μg/L Cu compared with the control group, while muscle LPO levels were significantly higher in fish exposed to 500, 750, and 1500 μg/L Cu compared with control group (Fig. 1).
Muscle reactive oxygen species (ROS) (a), lipid peroxidation (LPO) (b), and protein carbonylation (c) levels of Cichlasoma amazonarum adults maintained under control condition (no copper addition in the water) or exposed to waterborne copper for 96 h. Bars that do not share a common superscript letter differ significantly using a one-way analysis of variance (ANOVA) for independent samples followed by Tukey post hoc test (p < 0.05; n = 6 per group)
Muscle ACAP levels were significantly lower in fish exposed to 1500 μg/L Cu compared with the control group, while muscle SOD activity was significantly lower in fish exposed to 750 and 1500 μg/L Cu compared with control group and groups exposed to 25 and 250 μg/L Cu. No significant difference was observed between groups regarding muscle CAT activity (Fig. 2).
Muscle antioxidant capacity against peroxyl radicals (ACAP) (a) levels, superoxide dismutase (SOD) (b), and catalase (CAT) (c) activities of Cichlasoma amazonarum adults maintained under control condition (no copper addition in the water) or exposed to waterborne copper for 96 h. Bars that do not share a common superscript letter differ significantly using a one-way analysis of variance (ANOVA) for independent samples followed by Tukey post hoc test (p < 0.05; n = 6 per group)
Muscle GPx activity was significantly lower in fish exposed to 1500 μg/L Cu compared with the control group and groups exposed to 25 and 250 μg/L Cu. No significant difference was observed between groups regarding muscle GST and GR activities (Fig. 3).
Muscle glutathione peroxidase (GPx) (a), glutathione S-transferase (GST) (b), and glutathione reductase (GR) (c) activities of Cichlasoma amazonarum adults maintained under control condition (no copper addition in the water) or exposed to waterborne copper for 96 h. Bars that do not share a common superscript letter differ significantly using a one-way analysis of variance (ANOVA) for independent samples followed by Tukey post hoc test (p < 0.05; n = 6 per group)
Muscle fatty acid profile
Total SFA was significantly highest, while the total of MUFA and omega 6 (n6) were significantly lowest in the muscle of fish exposed to 1500 μg/L Cu (Table 2).
Muscle levels of C10:0 (capric acid), C13:0 (tridecanoic acid), and C18:0 (stearic acid) fatty acids were significantly highest in fish exposed to 1500 μg/L Cu. Muscle levels of C16:1 (palmitoleic acid) and C18:1n7c (vaccenic acid) fatty acids were significantly lowest in fish exposed to 1500 μg/L Cu, while the levels of C18:1n9c (oleic acid) was significantly lower in fish exposed to 1500 μg/L Cu compared with control group and fish exposed to 500 μg/L Cu. Muscle levels of C18:2n6 (linoleic acid) fatty acid were significantly lowest in fish exposed to 1500 μg/L Cu, while the levels of C22:5n3 (docosapentaenoic acid) and C22:6n3 (docosahexaenoic acid) fatty acids were significantly lower in fish exposed to 500, 750, and 1500 μg/L Cu compared with control group (Table 3).
Muscle Cu levels
Muscle Cu levels were significantly highest in fish exposed to 1500 μg/L Cu (Table 4).
Discussion
The current study clearly demonstrates that short-term exposure to different Cu concentrations induced muscle oxidative damage of C. amazonarum via alteration in oxidative homeostasis and impairment of enzymatic and non-enzymatic antioxidant defense system. Moreover, our data revealed that some fatty acids in muscle fish were affected by exposure to Cu concentrations as a consequence of excessive muscle lipid peroxidation and impairment of antioxidant system, which can affect the fatty acid quality and freshness value of fish fillet.
Oxidative stress is considered an important mode of toxic action for Cu in many fish species, being considered as a molecular initiation event or an early key event in adverse outcomes of exposure to Cu. However, some evidence has suggested that differences in several aspects of Cu toxicity, including oxidative stress, may be related to species-specific physiological abilities to regulate the redox system (Shekh et al. 2020). In this sense, oxidative stress-related parameters were evaluated in C. amazonarum in order to investigate the sensitivity of this fish species to oxidative stress when exposed to waterborne Cu levels found in Amazon water around mines. In the present study, muscle ROS levels were significantly higher in fish exposed to the highest waterborne Cu levels tested (1500 μg/L), which suggests an increase in free radical formation during acute exposure to high Cu levels. In agreement with our observations, a study conducted by Jiang et al. (2015) revealed a significant increase on muscle ROS levels of common carp (Cyprinus carpio) exposed for 96 h to waterborne Cu level (560 μg/L), concluding that Cu exposure depleted the ROS scavenging ability of fish and oxidized the lipids and proteins in the muscle. As a consequence of muscle ROS overproduction, we observed a significant increase on muscle lipid peroxidation (500, 750, and 1500 μg/L Cu) and protein carbonylation (1500 μg/L Cu) compared with control group, which reveals that excessive muscle ROS formation elicits the oxidation of lipid and proteins, contributing to Cu toxic effects. Tesser et al. (2020) also reported muscle lipid damage in curimbatá (C. lineatus) exposed 96 h to 24 μg/L Cu, concluding that lipid oxidation contributes to impairment of membrane fluidity and permeability and consequently, increased fish mortality. At the best of our knowledge, there are no reports about the effects of Cu on muscle protein damage. However, Ransberry et al. (2016) reported that killifish (Fundulus heteroclitus) exposed for 96 h to a Cu concentration (25 μg/L) presented gill protein damage via augmentation on protein carbonylation levels, being a consequence of excessive free radicals production. Under the tested condition (exposure time, Cu level, and analyzed tissue), it is possible to suggest that C. amazonarum is little affected in terms of oxidative damage, being more sensitive to lipid peroxidation than protein damage when exposed to different Cu levels.
In response to the increase of free radical formation, including ROS, the enzymatic or non-enzymatic antioxidant system can be upregulated or downregulated, and this response exerts a significant role in Cu toxicity. Thus, to evaluate whether Cu-induced ROS overproduction is related to inhibited scavenging capability, we determined the ROS scavenge ability in fish muscle via measurement of antioxidant enzymes and non-enzymes. In consequence of exacerbated muscle ROS production, fish muscle ACAP levels (1500 μg/L) and muscle SOD (750 and 1500 μg/L) activity were significantly inhibited by Cu exposure, indicating a minor capacity to scavenge the Cu-induced ROS overproduction. As our observation, Jiang et al. (2015) showed that muscle SOD activity is inhibited in common carp (C. carpio) exposed for 96 h to 560 μg/L, which contributed to Cu-induced lipid and protein damage. The antioxidant enzyme SOD acts as the first line of defense against oxygen toxicity through the conversion of superoxide anion to hydrogen peroxide (H2O2) and water (H2O) (Feng et al. 2015), and its inhibition indicates a minor capacity to eliminate an important ROS molecule, which can also explain its muscle increase in fish exposed to high Cu levels. In our study, no significant difference was observed regarding muscle CAT activity, which reveals that this enzyme is not involved on ROS elimination in the tested conditions, since its plays an important role in ROS detoxification during waterborne Cu exposure via degradation of H2O2 into H2O and O2 (Díaz-Alba et al. 2017). Also, it is important to emphasize that the absence of significant difference on muscle CAT activity may be a consequence of no significant increase on H2O2, since this enzyme changes in response to alteration on H2O2 levels. However, it is important to emphasize that both conditions have been found in the literature; i.e., CAT activity can be inhibited or stimulated, and these conditions are dependent on the length of time of exposure, Cu concentration, tissue analyzed, and fish species (Kim et al. 2014). Moreover, a significant decrease in muscle GPx activity was found in C. amazonarum exposed to high waterborne Cu concentration (1500 μg/L), as observed by Jiang et al. (2015) in the muscle of common carp exposed for 96 h to 560 μg/L. This enzyme presents widespread cellular distribution and catalyzes the reduction of both H2O2 and organic peroxides, indicating that GPx is one of the most important enzymes of the antioxidant defense system against Cu-induced oxidative damage (Díaz-Alba et al. 2017). Moreover, GPx plays a key role in protecting cell against lipid damage, and its depression can also explain the lipid damage observed in C. amazonarum exposed to Cu levels. Finally, no significant difference was observed regarding muscle GST and GR activities, as reported by Jiang et al. (2015) that verified that both enzymatic activities were not significantly affected in common carp (C. carpio) exposed for 96 h to 600 μg/L Cu. Also, it is important to highlight that reduced activities of antioxidant enzymes would result in the accumulation of ROS, confirmed by a sharp increase in LPO and protein carbonyl content in C. amazonarum. In the process, the weakened antioxidant defense system could not eliminate or neutralize the excessive ROS, which may further, in turn, inhibit enzyme activities or even degrade the enzymes through ROS-induced enzyme oxidation and protein damage (Yuan et al. 2017). Moreover, it is important to emphasize that Cu was able to accumulate only in the muscle of fish exposed to 1500 μg/L Cu, i.e., at the same level that caused an increase in ROS levels and impairment on the muscle antioxidant system. This allows us to hypothesize a direct effect of Cu accumulation on Cu-induced oxidative damage.
Recently, a study conducted by Das et al. (2018) revealed that alteration in oxidative balance and diminished activity of antioxidant enzymes are involved in change in the muscle fatty acid composition of Indian carps exposed to different metals. In this sense, we decided to evaluate whether acute exposure to Cu concentrations is capable of altering the muscle fatty acid composition of C. amazonarum. In the present study, exposure to a high Cu concentration (1500 μg/L) caused a significant increase on SFA level, while the total contents of MUFA and sum of n6 were significantly lower than the other groups of fatty acids, i.e., alterations on muscle fatty acid composition occurred at the concentration that Cu-induced oxidative damage and alterations on the muscle antioxidant system, which suggests a relationship between oxidative damage/alteration on the antioxidant system and alterations on the muscle fatty acid profile of C. amazonarum. Partially in agreement with our observations, Afridi et al. (2019) reported that Mrigal (Cirrhinus mrigala) exposed for 96 h to 1340 μg/L Cu presented a significant increase on the total content of muscle SFA, while no significant difference was observed regarding the total of MUFA and PUFA levels, as well as the sum of n3 and n6 fatty acids. Besides the effect of oxidative damage, these results can also be explained and can occur by two reasons: the first is linked to lipid mobilization to meet the energy demand through the oxidation process to help the fish to cope with challenge imposed by metals, as reviewed by Javed and Usmani (2019) for fish exposure to other metals. The second can be linked to a self-regulating and compensative mechanism of the fish muscle membrane to maintain the structural and functional stability when exposed to metals, as observed by Xiang et al. (2020) in gills of common carp exposed to silver. It is very important to emphasize that exposure to 500, 750, and 1500 μg/L Cu elicited a significant reduction on EPA and DHA fatty acids, two important fatty acids for fish health linked to the improvement of fish growth, enzyme activity, and cell signaling process (Calder and Yaqoob 2009). Thus, these alterations on the muscle fatty acid profile can negatively impair fish health, and probably affect human health.
Although C. amazonarum is not a fish used for human food, these data are an alarm about the possible quality of fillet associated with the composition of fatty acid in other fish in the Amazon. The fatty acid composition of the fish fillet is an important trait for consumers because some MUFA and PUFA fatty acids promote health (DiNicolantonio 2017), while some SFA are considered unhealthy (Mensink 2016). An increase of total content of SFA in the muscle of fish exposed to 1500 μg/Cu can be considered a negative impact for human nutrition due to their effects on cholesterol metabolism and higher LDL cholesterol levels, both of which are risk factors for coronary heart disease (CHD) (Mensink 2016). Similarly, a reduction in the total content of MUFAs can also be negative for human health since their consumption has been related to a reduction in cardiovascular diseases because they decrease inflammation, reduce triglycerides, and total cholesterol levels (Michielsen et al. 2019). Regarding specific fatty acids, only 9 from a total of 24 were significantly altered in the muscle of C. amazonarum exposed to Cu concentrations. In disagreement with our observations, Afridi et al. (2019) did not observe a significant difference in any of these fatty acids in the muscle of mrigal exposed for 96 h to 1340 μg/L Cu, but only a significant increase on C14:0 and a significant reduction on C22:0 (docosanoic acid) fatty acids compared with fish unexposed to Cu. Although without significant effects on the total content of PUFA, exposure to 500, 750, and 1500 μg/L Cu elicited a significant reduction on EPA and DHA fatty acids, which represents a concern for consumers. A meta-analysis by Kotwal et al. (2012) revealed that the consumption of EPA and DHA protects against cardiovascular death. Moreover, a meta-analysis of 31 clinical trials conducted by Delgado-Lista et al. (2012) revealed that consumption of marine-derived EPA and DHA for a period of 6 months reduced any cardiovascular event by 10%, and lowered risk of cardiac death and fatal or non-fatal coronary events by 9% and 18%, respectively, evidencing the importance of these fatty acids for human nutrition. In summary, exposure to Cu concentrations impairs the fish muscle fatty acid profile, which can cause negative impacts for fish consumers.
Based on this evidence, the results of this comprehensive study agree with the initial hypothesis that the exposure to waterborne Cu induced oxidative damage and inhibited enzymatic and non-enzymatic antioxidant response in the muscle of C. amazonarum exposed to high Cu levels. Moreover, the impairment of the fillet fatty acid profile appears to be mediated by oxidative damage, representing a negative impact on fish health and is a concern for fish consumers.
References
Abou Anni IS, Zebral YD, Afonso SB, Moreno Abril SI, Lauer MM, Bianchini A (2019) Life-time exposure to waterborne copper III: effects on the energy metabolism of the killifish Poecilia vivipara. Chemosphere 227:580–588
Aebi H (1984) Catalase in vitro. Methods Enzymol 105:121–126
Afridi AJ, Zuberi A, Yousafzai AM, Kamran M, Ullah S (2019) Hemp (marijuana) reverted copper-induced toxic effects on the essential fatty acid profile of Labeo rohita and Cirrhinus mrigala. Mol Biol Rep 46:391–401
Amado LL, Garcia ML, Ramos PB, Freitas RF, Zafalon B, Ferreira JLR, Yunes JS, Montserrat JM (2009) A method to measure total antioxidant capacity against peroxyl radicals in aquatic organisms: application to evaluate microcystins toxicity. Sci Total Environ 407:2115–2123
Baldissera MD, Souza CF, Barroso DC, Pereira RS, Alessio KO, Bizzi C, Baldisserotto B, Val AL (2020) Acute exposure to environmentally relevant concentrations of copper affects branchial and hepatic phosphoryl transfer network of Cichlasoma amazonarum: Impacts on bioenergetics homeostasis. Comp Biochem Physiol C Toxicol Pharmacol 238:108846
Bligh EG, Dyer WJ (1959) A rapid method of total lipid extraction and purification. Can J Biochem Physiol 37:911–917
Boareto AC, Giareta EP, Guiloski IC, Rodrigues MS, Freire CA, Silva-de-Assis HC (2018) Effects of short-term exposure to copper on biochemical biomarkers in juvenile freshwater fish. Pan-American J Aquatic Scie 13:135–147
Calder PC, Yaqoob P (2009) Omega-3 polyunsaturated fatty acids and human health outcomes. Biofactors 35:266–272
Carlberg I, Mannervik B (1985) Glutathione reductase. Methods Enzymol 113:484–490
Crémazy A, Wood CM, Smith DS, Ferreira MS, Johannsson OE, Giacomin M, Val AL (2016) Investigating copper toxicity in the tropical fish cardinal tetra (Paracheirodon axelrodi) in natural Amazonian waters: measurements, modeling, and reality. Aquatic Toxicol 180:353–363
Das D, Das P, Moniruzzaman M, Sarkar MP, Mukherjee J, Chakraborty SB (2018) Consequences of oxidative damage and mitochondrial dysfunction on the fatty acid profile of muscle of Indian major carps considering metal toxicity. Chemosphere 207:385–396
Delgado-Lista J, Perez-Martinez P, Lopez-Miranda J, Perez-Jimenez F (2012) Long chain omega-3 fatty acids and cardiovascular disease: a systematic review. Br J Nutr 107:S201–S213
Díaz-Alba M, Raya AC, Granado-Costa MD, Ramírez MO, El Mai B, García FC, Troyano-Montoro M, Espada-Bellido E, Santiago RT, Galindo-Riaño MD (2017) Biomarker responses of Cu-induced toxicity in European seabass Dicentrarchus labrax: assessing oxidative stress and histopathological alterations. Mar Poll Bul 124:336–348
DiNicolantonio JJ (2017) Good fats versus bad fats: a comparison of fatty acids in the promotion of insulin resistance, inflammation, and obesity. Miss Med 114:303–307
Feng M, He Q, Meng L, Zhang X, Sun P, Wang Z (2015) Evaluation of single and joint toxicity of perfluorooctane sulfonate, perfluorooctanoic acid, and copper to Carassius auratus using oxidative stress biomarkers. Aquatic Toxicol 161:108–116
Fry KL, Wheeler CA, Gillings MM, Flegal AR, Taylor MP (2020) Anthropogenic contamination of residential environments from smelter As, Cu and Pb emissions: implications for human health. Environm Poll 262:114235
Habig WH, Pabst MJ, Jakoby WB (1974) Glutathione S transferases. The first enzymatic step in mercapturic acid formation. J Biol Chem 249:7130–7139
Hader DP, Banaszak AT, Villafane VE, Narvarte MA, González RA, Helbing EW (2020) Anthropogenic pollution of aquatic ecosystems: emerging problems with global implications. Sci Total Environm 713:136586
Hartman L, Lago RC (1973) Rapid preparation of fatty acid methyl esters from lipids. Lab Pract 22:475–477
He Z, Li F, Dominech S, Wen X, Yang S (2019) Heavy metals of surface sediments in the Changjiang (Yangtze River) estuary: distribution, speciation and environmental risks. J Geochem Expl 198:18–28
Hrbek T, Farias IP, Crossa M, Sampaio I, Porto JIR, Meyer A (2005) Population genetic analysis of Arapaima gigas, one of the largest freshwater fishes of the Amazon basin: implications for its conservation. Anim Conserv 8:297–308
Jafari AJ, Kermani M, Kalantary RR, Arfaeinia H (2018) The effect of traffic on levels, distribution and chemical partitioning of harmful metals in the street dust and surface soil from urban areas of Tehran. Iran Environ Earth Sci 77:38
Javed M, Usmani N (2019) An overview of the adverse effects of heavy metal contamination on fish health. Proc Natl Acad Sci 89:389–403
Jiang WD, Liu Y, Jiang J, Wu P, Feng L, Zhou XQ (2015) Copper exposure induces toxicity to the antioxidant system via the destruction of Nrf2/ARE signaling and caspase-3-regulated DNA damage in fish muscle: amelioration by myo-inositol. Aquatic Toxicol 159:245–255
Kim WK, Park JW, Lim ES, Lee SK, Kim J, Lee SW, Choi K, Jung J (2014) Tissue-specific antioxidant responses in pale chub (Zacco platypus) exposed to copper and benzo[a]pyrene. Bull Environ Contam Toxicol 92:540–545
Kotwal S, Jun M, Sullivan D, Perkovic V, Neal B (2012) Omega 3 fatty acids and cardiovascular outcomes: systematic review and meta-analysis. Circ Cardiovasc Qual Outcomes 5:808–818
LeBel CP, Ischiropoulos H, Bondy SC (1992) Evaluation of the probe 2′,7′-dichlorofluorescin as an indicator of reactive oxygen species formation and oxidative stress. Chem Res Toxicol 5:227–231
Marklund S, Marklund G (1974) Involvement of the superoxide anion radical in the autoxidation of pyrogallol and a convenient assay for superoxide dismutase. Eur J Biochem 47:469–474
Mensink R (2016) Effects of saturated fatty acids on serum lipids and lipoproteins: a systematic review and regression analysis. World Health Organization, Geneva
Michielsen CCJR, Hangelbroek RWJ, Feskens EJM, Afman LA (2019) Disentangling the effects of monounsaturated fatty acids from other components of a mediterranean diet on serum metabolite profiles: a randomized fully controlled dietary intervention in healthy subjects at risk of the metabolic syndrome. Mol Nutr Food Res 63:e1801095
Mohanty BP, Mahanty A, Ganguly S, Mitra T, Karunakaran D, Anandan R (2019) Nutritional composition of food fishes and their importance in providing food and nutritional security. Food Chem 293:561–570
Monserrat JM, Geracitano LA, Pinho GLL, Vinagre TM, Faleiros M, Alciati JC, Bianchini A (2003) Determination of lipid peroxides in invertebrates using the Fe (III) xylenol orange complex formation. Arch Environ Contam Toxicol 45:177–183
Paglia DE, Valentine WN (1967) Studies on the quantitative and qualitative characterization of erythrocytes glutathione peroxidase. J Lab Clin Med 70:158–169
Ransberry VE, Blewett TA, McClelland GB (2016) The oxidative stress response in freshwater-acclimated killifish (Fundulus heteroclitus) to acute copper and hypoxia exposure. Comp Biochem Physiol C 179:11–18
Read SM, Northcote DH (1981) Minimization of variation in the response to different proteins of the Coomassie blue G dye-binding assay for protein. Anal Biochem 116:53–64
Reznick AZ, Packer L (1994) Oxidative damage of proteins: spectrophotometer for carbonyl assay. Methods Enzymol 233:357–363
Santana GP, Barroncas DSRP (2007) Estudo de metais pesados (Co, Cu, Fe, Cr, Ni, Mn, Pb e Zn) na Bacia do Tarumã-Açu Manaus (AM). Acta Amaz 37(1):11–118
Shekh K, Alcaraz AJ, Niyogi S, Hecker M (2020) Comparative analyses of oxidative stress response and metallothione in induction in white sturgeon and rainbow trout during acute waterborne copper exposure. Comp Biochem Physiol C 231:108723
Sies H, Berndt C, Jones DP (2017) Oxidative stress. Ann Review Biochem 86:715–748
Siqueira-Gay J, Sonter LJ, Sánchez LE (2020) Exploring potential impacts of mining on forest loss and fragmentation within a biodiverse region of Brazil’s northeastern Amazon. Res Pol 67:101662
Sissener NH, Araujo P, Saele O, Roselund G, Stubhaug I, Sanden M (2020) Dietary 18:2n-6 affects EPA (20:5n-3) and ARA (20:4n-6) content in cell membranes and eicosanoid production in Atlantic salmon (Salmo salar L.). Aquaculture 522:735098
Tesser ME, de Paula AL, Risso WE, Monteiro RA, Pereira AES, Fraceto LF, Martinez CBR (2020) Sublethal effects of waterborne copper and copper nanoparticles on the freshwater Neotropical teleost Prochilodus lineatus: a comparative approach. Sci Total Environ 704:135332
Viña J, Borras C, Gomez-Cabrera MC (2018) A free radical theory of frailty. Free Rad Biol Med 124:358–363
Xiang QQ, Wang D, Zhang JL, Ding CZ, Luo X, Tao J, Ling J, Shea D, Chen LQ (2020) Effect of silver nanoparticles on gill membranes of common carp: modification of fatty acid profile, lipid peroxidation and membrane fluidity. Environm Poll 256:113504
Yuan SS, Ming Z, Zhu AY, Zheng JL, Wu CW (2017) Negative effect of chronic cadmium exposure on growth, histology, ultrastructure, antioxidant and innate immune responses in the liver of zebrafish: preventive role of blue light emitting diodes. Ecotoxicol Environ Saf 139:18–26
Zebral YD, Roza M, Fonseca JS, Costa PG, de Oliveira CS, Zocke TG, Dal Pizzol JL, Robaldo RB, Bianchini A (2019) Waterborne copper is more toxic to the killifish Poecilia vivipara in elevated temperatures: linking oxidative stress in the liver with reduced organismal thermal performance. Aquatic Toxicol 209:142–149
Zhang Z, Lu Y, Li H, Tu Y, Liu B, Yang Z (2018) Assessment of heavy metal contamination, distribution and source identification in the sediments from the Zijiang River, China. Scie Total Environ 645:235–243
Acknowledgments
The authors would like to thank to the technician Reginaldo Oliveira for all logistical support. Thanks are due to Maria de Nazaré Paula da Silva for laboratory support.
Credit author statement
Matheus Baldissera: conceptualization, sample collection, data curation, and writing-reviewing; Danilo Barroso, Rogério Pereira, Cezar A. Bizzi, and Keiti Alessio: Cu analysis; Carine Souza: oxidative stress analysis; Fernanda C. de Oliveira and Roger Wagner: fatty acid analysis; Bernardo Baldisserotto and Adalberto Val: conceptualization and review.
Funding
This research was funded by a joint grant from the Brazilian National Research Council (CNPq, 465540/2014-7), the Amazonas State Research Foundation (FAPEAM, 062.01187/2017), and Coordination for the Improvement of Higher Education Personnel (CAPES, finance code 001) to ALV (INCT ADAPTA). ALV is the recipient of a research fellowship from the CNPq. CFS was the recipient of a fellowship from FAPEAM.
Author information
Authors and Affiliations
Corresponding author
Ethics declarations
Competing interests
The authors declare that they have no competing interests.
Additional information
Publisher’s note
Springer Nature remains neutral with regard to jurisdictional claims in published maps and institutional affiliations.
Rights and permissions
About this article
Cite this article
Baldissera, M.D., Souza, C.F., Barroso, D.C. et al. Consequences of oxidative damage on the fatty acid profile in muscle of Cichlasoma amazonarum acutely exposed to copper. Fish Physiol Biochem 46, 2377–2387 (2020). https://doi.org/10.1007/s10695-020-00884-8
Received:
Accepted:
Published:
Issue Date:
DOI: https://doi.org/10.1007/s10695-020-00884-8