Abstract
The purpose of this research was to study chemical characteristics of precipitation in Peradeniya (Latitude 6.973701, Longitude 79.915256), Kandy District in Sri Lanka. This study was conducted during 2012 to 2014, and wet precipitation was analyzed for pH, conductivity, Na+, NH4+, K+, Mg2+, Ca2+, F−, Cl−, NO3−, SO42−, Pb, Cu, Mn, Al, Zn, and Fe for a total of 125 samples. Less than 2% events of acidic precipitation were recorded, and the VWA of the major ionic species present in precipitation samples were in the order of Ca2+ > Na+ > Cl− > NH4+ > SO42− > K+ > NO3− > Mg2+. Neutralization of acidity of precipitation is much more related to CaCO3 than NH3, and the presence of high content of Ca2+ strongly supports this fact. When considering marine contribution, SO42−/Na+, Ca2+/Na+, Mg2+/Na+, and K+/Na+ ratios are higher than the reference value suggesting that contribution of sources other than marine. Concentration of Zn is the highest while that of Mn is the lowest. Principal component analysis identified probable sources for major ionic and elemental sources as both natural and anthropogenic sources such as vehicular emission due to heavy traffic, waste incineration, bio mass burning, brass industry, and construction.
Similar content being viewed by others
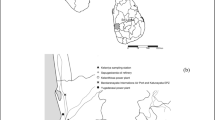
Explore related subjects
Discover the latest articles, news and stories from top researchers in related subjects.Avoid common mistakes on your manuscript.
Introduction
Atmospheric deposition can be referred to the introduction of different types of pollutants, mainly dust, metal ions and their compounds, anions, nutrients, and volatile organic compounds, generated through various anthropogenic and natural activities, into ecosystems (Yu et al. 2017; Rao et al. 2016; Huang et al. 2019; Sakihama et al. 2008). Among them, burning fossil fuels would probably contribute to significant level toward acid rain owing to the release of sulfur and nitrogen-containing compounds to the atmosphere (Ghorani-Azam et al., 2016). It has also been reported that sulfur concentrations in lake waters began to increase after the industrial revolution, supporting the above fact (Weyhenmeyer and Karlsson, 2009).
It is known that in-cloud scavenging and below-cloud scavenging are the two major types of wet scavenging processes of pollutants present in the atmosphere (Alastuey et al. 2001; Lee et al. 2000; Mahato et al. 2016; Cao et al. 2009; Herrera et al. 2009; Zhang et al. 2007; Carvalho et al. 2014; Zhu et al. 2016). It is believed that dissolution of pollutants would change the characteristics of rainwater, and therefore, determination of the chemical composition of rainwater, which is an indirect measure of the extent of pollution, would be very important for atmospheric quality management (Huang et al., 2008a; Alkhashman 2005; Wu and Han 2015; Tositti et al. 2018). Among various sources of atmospheric pollution, the prominent source of aerosols is the dust released from arid regions, followed by soil and marine erosion, and anthropogenic sources (Amodio et al. 2014). Although dry deposition appears to be a simple process, it occurs with several mechanisms, such as turbulent diffusion, sedimentation, Brownian diffusion, interception, inertial forces, thermophoresis and diffusiophoresis, and hence it is very complex (Amodio et al. 2014). Consequently, rate of dry deposition is controlled by meteorological factors (wind velocity, relative humidity), particle characteristics (size and shape), and surface characteristics (friction velocity, microscale roughness, and temperature) (Chen et al. 2012; Kim et al. 2016). On the other hand, wet deposition occurs through rain events, which is also governed by meteorological conditions, where the extent of deposition is determined through the concentration of chemical species in precipitation samples and the extent of precipitation. Thus, dry deposition is dominated by the transfer of particulate matter, total phosphorous, Ca2+, Mg2+, and K+, whereas wet deposition by the atmospheric delivery of Na+ and N species (Morales-Baquero et al., 2013).
Undesirable effects of atmospheric deposition can be predicted with the aid of bioindicators, such as moss species and bryophytes (Jayalath et al. 2020; Schröder et al. 2017; Shi et al. 2017). Sensitivity of bryophytes toward nitrogen levels has made it possible to use them to monitor atmospheric nitrogen deposition which could be due to environmental pollution and land use practices (Shi et al. 2017). A study on the use of moss species to compare atmospheric deposition in two geographic areas in Sri Lanka has also been reported (Weerasundara, et al. 2018). However, it is important to investigate whether the concentrations of nitrogen and/or heavy metals estimated through biomonitoring of atmospheric deposition lead to meaningful results (Schröder et al. 2017).
Rapid economic development of South Asia, South-East Asia, and China during the last few decades increased demand for energy (Li et al. 2016; Park et al. 2015). According to the state environmental protection administration of China, acid precipitation has generally affected about 30% of the country, and seriously affected in several parts including industrialized areas of East and South China. Unlike water pollution, which is restricted to certain areas, atmospheric pollution has no boundaries, and transboundary effects could cause environmental damages to neighboring countries. It has already been reported that transboundary pollution originating from countries in the Asian region, such as India, China, and Thailand, has contributed to deposition of environmental pollutants in Sri Lanka (Ileperuma 2015).
Sri Lanka, being an island has both positive and negative impacts with regard to atmospheric pollution. Diffusing out of atmospheric pollutants from coastal cities is an example for former, while ship movements around the country contributing to acid deposition is be an example for the latter (Ileperuma 2015, Chathuranga et al. 2020). Kandy, the second largest city in Sri Lanka is located in the central hills surrounded by mountains. Although there are not much industrial activities in Kandy, atmospheric pollutants once generated within the city area would not diffuse out at a significant rate, requiring a regular atmospheric pollution monitoring program for the city of Kandy.
There were few isolated studies on atmospheric deposition in Sri Lanka. Among them, heavy metals and microorganisms present in atmospheric deposition have been characterized in Kandy, Sri Lanka, together with health implications (Weerasundara et al. 2017, 2018). It has been reported that Al and Fe are at uncontaminated levels, and other metals investigated, Cr, Mn, Ni, Cu, Zn, Cd and Pb, are in the range of uncontaminated to contaminated levels, but with the potential to exacerbate in the long-term (Weerasundara et al. 2018). It has also been implicated that traffic and land use influence atmospheric metal concentrations. Nevertheless, detailed investigation on the composition of atmospheric deposition with regard to anions, cations, and other pollution parameters and their correlation studies have not been reported to date. Although there are few studies related to the bulk deposition, this detailed study is the first of that sort carried out in Sri Lanka to the best of our knowledge.
The results of this research can be used in prediction and modeling of rain water quality variation due to anthropogenic activities which could alter the atmospheric environment. Also, the scarcity of water has already affected many parts of the world, and as the access to good quality water is becoming a problem, rain water harvesting has been paid much attention. However, it is important to study temporal variation of rain water quality which could impact harvesting of water. This project thus aims to fill this void by investigating composition of rain water collected in a selected area in Peradeniya, Sri Lanka, for selected chemical parameters.
Materials and methods
Study area
The sampling location is University of Peradeniya (Latitude 6.973701, Longitude 79.915256) in Kandy District of the Central Province of Sri Lanka. The mean altitude of this location is 400 m above sea level and covered by mountains. University of Peradeniya is located near the Kandy-Colombo (A1) highway where high traffic digestion is present during daytime. High rainfall occurs in Peradeniya by monsoon, and much rainfall is received during South Western monsoon from May to September. Less socioeconomic impact can be seen in this area with less industrial activities (Fig. 1).
Sampling
The sample collector (Polyethylene container and funnel) was placed 120 cm above the ground level (Fig. 2). Diameter of the funnel was 20.6 cm. All glassware, funnels, and containers used for sampling, storage, and standard preparation were first cleaned with phosphate-free detergent solution followed by deionized water. Thereafter, they were placed in 5% HNO3 acid solution overnight followed by thoroughly cleaning by ASTM type 1 water until all acid was washed out. Sampling was done after events of precipitation starting from December 2012 to June 2014. If a rain event did not occur for 1 week, the container and the funnel were washed using 200 ml of deionized water and replaced with new collector. A total of 110 wet samples were collected during the entire period of sampling.
After measuring the volume of each sample, they were filtered using 0.45 μm nylon filters and divided into two parts; one part was stored in pre-cleaned polyethylene bottles and refrigerated at 4 °C prior to ion analysis (Rao et al. 2016; Huang et al., 2008b). The other part was stored in pre-cleaned polyethylene bottles followed by acidification to about pH < 2 by adding analytical grade HNO3 (BDH VWR, 69%, AnalaR NORMAPUR) and refrigerated at 4 °C prior to metal analysis. Conductivity and pH of rainwater samples were measured soon after sampling using Sens ION+ EC5 portable conductivity meter and Orion 3 Star pH meter, respectively.
Selected cations (Na+, NH4+, K+, Mg+2, Ca+2) and anions (Cl−, NO3−, SO42−) were quantified using Thermo Dionex ICS 900 ion chromatograph with chemical suppression mode. For anion analysis, Dionex ionpack AS23 (4 × 250 mm) column was used, while Dionex ionpack CS12A (4 × 250 mm) column was used for cation analysis. The detection limits of F−, Cl−, NO3−, and SO42− ions were found to be 1.6, 1.1, 1.0, and 2.0 μeq l−1, respectively, and those of Na+, NH4+, K+, Mg2+, and Ca2+ were 2.2, 2.8, 1.5, 6.5, and 6.0 μeq l−1, respectively (Liyandeniya et al. 2020a).
Trace metals (Al, Fe, Mn, Zn, Cu, Pb) were determined using Graphite Furnace Atomic Absorption Spectrometer (GFAAS) (Thermo electron M5 GF95 Graphite Furnace System) with deuterium background correction. Detection limits for elements were within the range of 0.1–2.0 μg l−1.
Quality of the analysis was achieved by running known standards with the same matrix as analyte (both ions and elements) between 20 sample-intervals to check reproducibility of analytical results. Confidence level of 95% was maintained for all analyses. Weather data were measured using Watchdog 2700 automated weather stations located at two stations.
Statistical analysis
The principal component analysis (PCA) was applied to the determination of the sources of respective pollutants whether anthropogenic or natural. Minitab 16 statistical software was used for statistical treatment of data.
Results and discussion
Rainfall data
Figure 3 shows rainfall measurements throughout the period of sampling at Peradeniya. Rain is received at Peradeniya during two monsoons; South-Western monsoon that prevails during May to September, and North-Eastern monsoon that prevails during October to February. Consequently, moderate drought season usually prevails in the months from December to March. This might lead to increase dry particulate matter in the atmosphere and deposited with rain as well as with dry deposition. However, in the year 2014, the first inter-monsoon rain started in April. Nevertheless, when the entire year 2014 was considered, it had more dry periods and lower rainfall as compared to the previous year.
Investigation of pH variation of depositions
Figure 4 shows the frequency histogram of pH for samples of atmospheric precipitation. Only two acid precipitation events were recorded during the entire sampling period which accounts for only 2% of total samples collected. The average pH was 6.55, and the VWA of pH values was 6.22 within the pH range between 5.26 and 7.48. This pH variation reflects the minor anthropogenic activities, such as traffic congestion, in this area, mainly during daytime. On the other hand, neutralization would occur due to the presence of considerable amounts of Ca2+ and NH4+ in the atmosphere. When these values are compared with those reported in other parts of the world including India, it is clear that the pH values recorded in the study area are on the high side.
Chemical composition
Table 1 presents VWA values, ranges obtained for pH, conductivity, inorganic ions including F−, Cl−, NO3−, SO42−, Na+, NH4+, K+, Mg2+, and Ca2+ along with chemical elements for a total of 125 precipitation samples collected. The VWA value and range for conductivity were 19.40 μS cm−1 and 4.00–136.10 μS cm−1, respectively. The VWA concentrations of the major ionic species present in precipitation samples were in the order of Ca2+ > Na+ > Cl− > NH4+ > SO42− > K+ > NO3− > Mg2+. Although there is a difference in the order of ions, Ca2+, Na+, Cl−, NH4+, and SO42− are found to be the dominant ions. The F− ion was undetectable for all samples. Similar observation has been made in India in 2017 (Bhaskar & Rao 2017; Bisht et al. 2017). It is clearly indicated in Fig. 5 that a much higher contribution of 22.6% was recorded for Ca2+, while Mg2+ contributes to only 4%. It has also been reported that marine contribution was indicated by the presence of Na+ and Cl− at high concentrations (Li et al. 2016) although the sampling locations were far away from the coast. The most abundant cation in the sampling sites was Ca2+, which is probably be due to dust particles which contains Ca2+(Martins, et al., 2019; Migliavacca et al. 2005). The Ca2+ ion plays a major role along with NH4+ in neutralization of acidified rain. This is further supported by the fact that high pH was recorded together high SO42− concentration. More importantly, these ions are mainly found in dry months during November to February where South-East monsoon is not occurred.
Quantification of chemical elements
Concentration of elements (Pb, Cu, Al, Zn, Fe, and Mn) determined in rainwater, as shown in Table 1, indicates that Pb levels in samples collected at almost all stations are lower than the MDL value. This is probably due to the lack of Pb sources in these areas, and abandoning the usage of leaded gasoline which had been used many years ago. The element having the highest concentration was Zn followed by Al, Fe, Cu, and Mn. Major input pathways of Zn, Cu, and Fe into air are brass industry in smaller scale, waste incineration, traffic during daytime and occasional forest burning. On the other hand, Al and Mn are mostly from natural processes, and further, Al is one of the major constituents in soil. Moreover, construction and soil erosion could also contribute to Al in the atmosphere. Dissolution of the above chemical elements is one of the important phenomena to leach them into rainwater. This may be governed by moderately acidic pH and some rain events with acidic pH below 5.60. Apart from the above-stated contributions, Sri Lanka, being a small island in the middle of the Indian Ocean, trans-boundary pollution also plays an important role for elevated levels of these elements.
Acidification and neutralization potentials
Acidity of precipitation is mainly controlled by strong acids, such as H2SO4 and HNO3, and to lesser extent by organic acids. Neutralization of these species would take place due to the presence of NH4+ and CaCO3 (Migliavacca. et al. 2005). As stated earlier, the pH of precipitation is strongly controlled by the presence of these acids and bases, and neutralization reactions among them. Figure 6 shows the linear regression analysis applied to the variables with acidification and variables with neutralization potential. The correlation coefficient is found to be 0.39.
Table 2 shows the results of regression coefficient analysis, and approximately 63.7% of SO42−and 93.3% of NO3− were able to be explained by the two independent variables, Ca2+ and NH4+. Of 63.7% of SO42− explained, 32.2% appears as CaSO4 and only 31.5% as (NH4)2SO4. As for NO3−, it is found as Ca(NO3)2 in 62.6% of the cases and as NH4NO3 in only 30.7% of the cases in bulk precipitation. Further, neutralization of acidity of precipitation is much more related to CaCO3 than to NH3, and high content of Ca2+ ions strongly supports these results. Source of NH3 is mainly the application of fertilizers and biomass burning.
Marine contribution
Ratio between the ion analyzed and Na+ is higher than the ratio of the two ions for sea water indicating negligible marine contribution (Herrera et al. 2009). Ratios for SO42−/Na+, Ca2+/Na+, K+/Na+, and Mg2+/Na+, being higher than the reference values suggest that the marine contribution is negligible, and contribution of anthropogenic sources is high for these ions (Table 3) (Migliavacca. et al. 2005). Furthermore, the ratios for Cl−/Na+ is less than those of the reference values indicating the strong marine influence for these ions. However, the ratio for Ca2+/Na+ is 1.16, and such a higher ratio can be attributed to the contribution from dust material containing CaCO3 in addition to other anthropogenic activities. The presence of CaCO3 in the atmosphere is contributed by many dolomite quarries established in surrounding areas of the sampling location.
Principal component analysis for major ions
After applying principal component analysis, three principal components were selected for ionic species with eigenvalues greater than 1 (Table 4). Three PCs selected explained 69.2% variation from the total variation. Among them, PC1 explained 39.4% variation which showed higher loading for Cl−, SO42−, Na+, and Mg2+. These species are emitted by both anthropogenic and natural activities. Further, Cl− and Na+ are originated from marine and transferred long distances with wind. On the other hand, acidic SO42− is originated from anthropogenic activities, such as vehicular emission, and it is further supported by heavy traffic present near Peradeniya suburb. Mg2+ ions originated from soil, and surrounding soil is composed of Mg bearing minerals further supported this finding. PC2 explained 16.6% variation and showed higher loading for H+ and NO3− (Migliavacca. et al. 2005). Higher loading of NOx may be due to fossil fuel burning which is further supported by heavy traffic in the area at times. Also, it increases H+ ions and shows positive correlation with NO3−. PC3 which only explains about 13.1% of the total variation showed higher loading for H+. At the same time, it showed large but negative loading for both NH4+ (− 0.645) and Ca2+ (− 0.405) supporting neutralization of acidic species present in the wet precipitation. Sources for the NH4+ are frequent bio mass and waste incineration, and fertilizer application while Ca2+ probably from dolomite quarries present in nearby areas (Liyandeniya et al. 2020b).
Principal component analysis for elements
Two principal components were selected which have eigenvalue greater than 1 for elements present in wet precipitation samples collected. The results of the principal component analysis explain 46.8% of the total variance of the data (Table 5). The PC1 has shown higher loading of Cu, Al, Zn, and Fe with 29.2% of the total variation. Sources for these elemental species are from both anthropogenic and natural (Al and Fe). Traditional brassware industry may resulted the higher loading of Cu while Al and Fe loading is due to the construction (Liyandeniya et al. 2020a). Also, Al is due to natural sources such as dust which contains alumino silicates. Major sources of Zn include roofing materials, lubricants, and metal plating (Rathnayaka at al. 2020). Second component explains 17.6% of total variation, and higher loading of Mn and H+ can be due to automobile emission and waste incineration which may contain Mn as an impurity or additive. This can be further supported by loading of H+ as such processes may release acidic gases during combustion of fossil fuel and waste incineration.
Conclusion
Analysis of wet precipitation at Peradeniya in Kandy District of Sri Lanka covering a period of 22 months starting from 2012 to 2014 indicates that majority of events recorded the pH ranging from 6.00 to 7.00 with less than 2% events of acidic precipitation in which pH was less than 5.60.. Approximately, 63.7% of SO42− and 93.3% of NO3−, in wet precipitation events at Peradeniya, were able to be explained by the two independent variables, Ca2+ and NH4+. Further, neutralization of acidity of precipitation is much more related to CaCO3 than to NH3, and higher content of Ca2+ ions strongly supports this result. The ratios, SO42−/Na+, Ca2+/Na+, Mg2+/Na+, and K+/Na+ are higher than the reference values, suggesting that marine contribution is negligible, and contribution of anthropogenic sources is predominant for these ions. On the other hand, the ratios of Cl−/Na+ are less than the reference values indicating marine influence of Cl− ions. It is also found that Zn is the most predominant element, while Mn is the least. Investigation of principal component analysis of major ionic species and elements (Ca2+, SO42−, Mg2+, Al, Cu, Fe, Zn, and H+) in wet precipitation samples identified probable anthropogenic sources, such as heavy traffic, biomass burning, waste incineration, construction activities, and brass industry, in nearby locations.
References
Alastuey, A., Querol, X., Chaves, A., Lopezsoler, A., & Ruiz, C. R. (2001). Wet-only sequential deposition in a rural area in north-eastern Spain. Tellus Series B: Chemical and Physical Meteorology, 53, 40–52. https://doi.org/10.3402/tellusb.v53i1.16535.
Alkhashman, O. (2005). Study of chemical composition in wet atmospheric precipitation in Eshidiya area, Jordan. Atmospheric Environment, 39, 6175–6183. https://doi.org/10.1016/j.atmosenv.2005.06.056.
Amodio, M., Catino, S., Dambruoso, P. R., de Gennaro, G., Di Gilio, A., Giungato, P., & Tutino, M. (2014). Atmospheric deposition: sampling procedures, analytical methods, and main recent findings from the scientific literature. Advances in Meteorology, 2014, 27. https://doi.org/10.1155/2014/161730.
Bhaskar, V. V., & Rao, P. S. P. (2017). Annual and decadal variation in chemical composition of rain water at all the ten GAW stations in India. Journal of Atmospheric Chemistry, 74(1), 23–53. https://doi.org/10.1007/s10874-016-9339-3.
Bisht, D. S., Srivastava, A. K., Joshi, H., Ram, K., Singh, N., Naja, M., & Tiwari, S. (2017). Chemical characterization of rainwater at a high-altitude site “Nainital” in the central Himalayas, India. Environmental Science and Pollution Research, 24(4), 3959–3969. https://doi.org/10.1007/s11356-016-8093-z.
Cao, Y. Z., Wang, S., Zhang, G., Luo, J., & Lu, S. (2009). Chemical characteristics of wet precipitation at an urban site of Guangzhou, South China. Atmospheric Research, 94(3), 462–469. https://doi.org/10.1016/j.atmosres.2009.07.004.
Carvalho, S. C. P., de Lima, J. L. M. P., & de Lima, M. I. P. (2014). Rainwater sequential sampler: assessing intra-event water composition variability. Journal of Engineering and Technology Research, 1(1), 1–7http://journal.iugaza.edu.ps/index.php/JERT/article/view/26.
Chathuranga, R. A. J., Liyandeniya, A. B., Dharmapriya, T. N., Deeyamulla, M. P., & Priyantha, N. (2020). Risk assessment and source apportionment of wet bulk deposition in three typical sites of Gampaha District, Sri Lanka. SN Applied Sciences, 2, 1394. https://doi.org/10.1007/s42452-020-3007-6.
Chen, L., Peng, S., Liu, J., & Hou, Q. (2012). Dry deposition velocity of total suspended particles and meteorological influence in four locations in Guangzhou, China. Journal of Environmental Sciences, 24(4), 632–639. https://doi.org/10.1016/S1001-0742(11)60805-X.
Ghorani-Azam, A., Riahi-Zanjani, B., & Balali-Mood, M. (2016). Effects of air pollution on human health and practical measures for prevention in Iran. Journal of Research in Medical Sciences, 21(5), 65. https://doi.org/10.4103/1735-1995.189646.
Herrera, J., Rodríguez, S., & Baéz, A. P. (2009). Chemical composition of bulk precipitation in the metropolitan area of Costa Rica, Central America. Atmospheric Research, 94(2), 151–160. https://doi.org/10.1016/j.atmosres.2009.05.004.
Huang, Y., Wang, Y., & Zhang, L. (2008a). Long-term trend of chemical composition of wet atmospheric precipitation during 1986-2006 at Shenzhen City, China. Atmospheric Environment, 42(16), 3740–3750. https://doi.org/10.1016/j.atmosenv.2007.12.063.
Huang, K., Zhuang, G., Xu, C., Wang, Y., & Tang, A. (2008b). The chemistry of the severe acidic precipitation in Shanghai, China. Atmospheric Research, 89(1–2), 149–160. https://doi.org/10.1016/j.atmosres.2008.01.006.
Huang, F., Zhou, J., Chen, N., Li, Y., Li, K., & Wu, S. (2019). Chemical characteristics and source apportionment of PM2.5 in Wuhan, China. Journal of Atmospheric Research, 76(3), 245–262. https://doi.org/10.1007/s10874-019-09395-0.
Ileperuma, O. (2015). Model assessment of acid deposition potential by SOx in Sri Lanka. Journal of the National Science Foundation of Sri Lanka, 43(3), 281. https://doi.org/10.4038/jnsfsr.v43i3.7956.
Jayalath, K. G., Deeyamulla, M. P., & de Silva, R. C. L. (2020). Atmospheric deposition of polycyclic aromatic hydrocarbons (PAHs) around two metropolitan areas in Sri Lanka using moss as a biomonitor. Pollution Research, 39(3), 626–631.
Kim, Y., Wellum, G., Mello, K., Strawhecker, K. E., Thoms, R., Giaya, A., & Wyslouzil, B. E. (2016). Effects of relative humidity and particle and surface properties on particle resuspension rates. Aerospace Science and Technology, 50(4), 339–352. https://doi.org/10.1080/02786826.2016.1152350.
Lee, B. K., Hong, S. H., & Lee, D. S. (2000). Chemical composition of precipitation and wet deposition of major ions on the Korean peninsula. Atmospheric Environment, 34(4), 563–575. https://doi.org/10.1016/S1352-2310(99)00225-3.
Li, Y. C., Zhang, M., Shu, M., Ho, S. S. H., Liu, Z. F., Wang, X. X., & Zhao, X. Q. (2016). Chemical characteristics of rainwater in Sichuan basin, a case study of Ya’an. Environmental Science and Pollution Research, 23(13), 13088–13099. https://doi.org/10.1007/s11356-016-6363-4.
Liyandeniya, A. B., Deeyamulla, M. P., & Priyantha, N. (2020a). Atmospheric chemical composition of bulk deposition at two geographically distinct locations in Sri Lanka. Environmental Monitoring and Assessment, 192(452), 452. https://doi.org/10.1007/s10661-020-08412-0.
Liyandeniya, A. B., Deeyamulla, M. P., & Priyantha, N. (2020b). Source apportionment of rainwater chemical composition in wet precipitation at Kelaniya in Sri Lanka. Air Quality and Atmospheric Health. https://doi.org/10.1007/s11869-020-00903-w.
Mahato, K., Singh, K., Singh, K., & Tiwari, K. (2016). Assessment of major ionic compositions and anthropogenic influences in the rainwater over a coal mining environment of Damodar River basin, India. Pollution, 2(4), 461–474. https://doi.org/10.7508/pj.2016.04.008.
Martins, E. H., Nogarotto, D. C., Mortatti, J., & Pozza, S. A. (2019). Chemical composition of rainwater in an urban area of the southeast of Brazil. Atmospheric Pollution Research, 10(2), 520–530. https://doi.org/10.1016/j.apr.2018.10.003.
Migliavacca, D., Teixeira, E., Wiegand, F., Machado, A., & Sanchez, J. (2005). Atmospheric precipitation and chemical composition of an urban site, Guaba hydrographic basin, Brazil. Atmospheric Environment, 39(10), 1829–1844. https://doi.org/10.1016/j.atmosenv.2004.12.005.
Morales-Baquero, R., Pulido-Villena, E., & Reche, I. (2013). Chemical signature of saharan dust on dry and wet atmospheric deposition in the south-western mediterranean region. Tellus Series B: Chemical and Physical Meteorology, 65(1), 1–12. https://doi.org/10.3402/tellusb.v65i0.18720.
Park, S. M., Seo, B. K., Lee, G., Kahng, S. H., & Jang, Y. (2015). Chemical composition of water soluble inorganic species in precipitation at Shihwa Basin, Korea. Atmosphere, 6(6), 732–750. https://doi.org/10.3390/atmos6060732.
Rao, P. S. P., Tiwari, S., Matwale, J. L., Pervez, S., Tunved, P., Safai, P. D., & Hopke, P. K. (2016). Sources of chemical species in rainwater during monsoon and non-monsoonal periods over two mega cities in India and dominant source region of secondary aerosols. Atmospheric Environment, 146, 90–99. https://doi.org/10.1016/j.atmosenv.2016.06.069.
Rathnayaka, I. M. S. K., Dharmapriya, T. N., Liyandeniya, A. B., Deeyamulla, M. P., & Priyantha, N. (2020). Trace metal composition of bulk precipitation in selected locations of Kandy District, Sri Lanka. Water, Air, & Soil Pollution, 231, 492. https://doi.org/10.1007/s11270-020-04840-3.
Sakihama, H., Ishiki, M., & Tokuyama, A. (2008). Chemical characteristics of precipitation in Okinawa Island, Japan. Atmospheric Environment, 42(10), 2320–2335. https://doi.org/10.1016/j.atmosenv.2007.12.026.
Schröder, W., Nickel, S., Schönrock, S., Schmalfuß, R., Wosniok, W., Meyer, M., & Zechmeister, H. G. (2017). Bioindication and modelling of atmospheric deposition in forests enable exposure and effect monitoring at high spatial density across scales. Annals of Forest Science, 74(2), 31. https://doi.org/10.1007/s13595-017-0621-6.
Shi, X. M., Song, L., Liu, W. Y., Lu, H. Z., Qi, J. H., Li, S., & Wu, C. S. (2017). Epiphytic bryophytes as bio-indicators of atmospheric nitrogen deposition in a subtropical montane cloud forest: response patterns, mechanism, and critical load. Environmental Pollution, 229, 932–941. https://doi.org/10.1016/j.envpol.2017.07.077.
Tositti, L., Pieri, L., Brattich, E., Parmeggiani, S., & Ventura, F. (2018). Chemical characteristics of atmospheric bulk deposition in a semi-rural area of the Po Valley (Italy). Journal of Atmospheric Chemistry, 75(1), 97–121. https://doi.org/10.1007/s10874-017-9365-9.
Weerasundara, L., Amarasekara, R. W. K., Magana-Arachchi, D. N., Ziyath, A. M., Karunaratne, D. G. G. P., Goonetilleke, A., & Vithanage, M. (2017). Microorganisms and heavy metals associated with atmospheric deposition in a congested urban environment of a developing country: Sri Lanka. Science of the Total Environment, 584–585, 803–812. https://doi.org/10.1016/j.scitotenv.2017.01.121.
Weerasundara, L., Magana-Arachchi, D. N., Ziyath, A. M., Goonetilleke, A., & Vithanage, M. (2018). Health risk assessment of heavy metals in atmospheric deposition in a congested city environment in a developing country: Kandy City, Sri Lanka. Journal of Environmental Management, 220, 198–206. https://doi.org/10.1016/j.jenvman.2018.04.036.
Weyhenmeyer, G. A., & Karlsson, J. (2009). Nonlinear response of dissolved organic carbon concentrations in boreal lakes to increasing temperatures. Limnology and Oceanography, 54(6 PART 2), 2513–2519. https://doi.org/10.4319/lo.2009.54.6_part_2.2513.
Wu, Q., & Han, G. (2015). Sulfur isotope and chemical composition of the rainwater at the three gorges reservoir. Atmospheric Research, 155, 130–140. https://doi.org/10.1016/j.atmosres.2014.11.020.
Yu, H., He, N., Wang, Q., Zhu, J., Gao, Y., Zhang, Y., & Yu, G. (2017). Development of atmospheric acid deposition in China from the 1990s to the 2010s. Environmental Pollution, 231(3), 182–190. https://doi.org/10.1016/j.envpol.2017.08.014.
Zhang, M., Wang, S., Wu, F., Yuan, X., & Zhang, Y. (2007). Chemical compositions of wet precipitation and anthropogenic influences at a developing urban site in southeastern China. Atmospheric Research, 84(4), 311–322. https://doi.org/10.1016/j.atmosres.2006.09.003.
Zhu, G., Guo, Q., Chen, T., Lang, Y., Peters, M., Tian, L., & Wang, C. (2016). Chemical and sulfur isotopic composition of precipitation in Beijing, China. Environmental Science and Pollution Research, 23(6), 5507–5515. https://doi.org/10.1007/s11356-015-5746-2.
Acknowledgments
This study received financial support from the National Research Council of Sri Lanka (Grant No. NRC/11/127) in providing equipment and other expenses.
Author information
Authors and Affiliations
Corresponding author
Additional information
Publisher’s note
Springer Nature remains neutral with regard to jurisdictional claims in published maps and institutional affiliations.
Rights and permissions
About this article
Cite this article
Liyandeniya, A.B., Deeyamulla, M.P., Abeysundara, S.P. et al. Chemical characteristics of wet precipitation at Peradeniya in Sri Lanka. Environ Monit Assess 193, 14 (2021). https://doi.org/10.1007/s10661-020-08772-7
Received:
Accepted:
Published:
DOI: https://doi.org/10.1007/s10661-020-08772-7