Abstract
Sevoflurane is a widely used inhalational anesthetic in pediatric medicine that has been reported to have deleterious effects on the developing brain. Strategies to mitigate these detrimental effects are lacking. Sirtuin 2 (SIRT2) is a member of nicotinamide adenine dinucleotide (NAD+)-dependent protein deacetylases involved in a wide range of pathophysiological processes. SIRT2 inhibition has emerged as a promising treatment for an array of neurological disorders. However, the direct effects of SIRT2 on anesthesia-induced damage to the immature brain are unclear. Neonatal rats were exposed to 3% sevoflurane or 30% oxygen for 2 h daily with or without SIRT2 inhibitor AK7 pretreatment from postnatal day 7 (P7) to P9. One cohort of rats were euthanized 6, 12, and/or 24 h after the last gas exposure, and brain tissues were harvested for biochemical analysis and/or immunohistochemical examination. Cognitive functions were evaluated using the open field and Morris water maze tests on P25 and P28–32, respectively. SIRT2 was significantly up-regulated in neonatal rat hippocampus at 6 and 12 h post-anesthesia. Pretreatment with SIRT2 inhibitor AK7 reversed sevoflurane-induced hippocampus-dependent cognitive impairments. Furthermore, AK7 administration mitigated sevoflurane-induced neuroinflammation and microglial activation. Concomitantly, AK7 inhibited pro-inflammatory/M1-related markers and increased anti-inflammatory/M2-related markers in microglia. AK7 might prevent sevoflurane-induced neuroinflammation by switching microglia from the M1 to M2 phenotype. Downregulation of SIRT2 may be a novel therapeutic target for alleviating anesthesia-induced developmental neurotoxicity.
Similar content being viewed by others
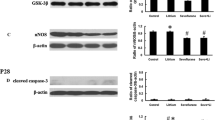
Avoid common mistakes on your manuscript.
Introduction
Growing evidence suggests that general anesthetics commonly used in the clinic may trigger neurotoxic changes in the developing brain. Research has reported an association between early-life anesthesia exposure and subsequent neurocognitive deficits (Bilotta et al. 2017), which is a concerning issue. Research over the past several decades has provided greater insight into potential mechanisms underlying anesthesia-induced developmental neurotoxicity (Satomoto and Makita 2016). Nevertheless, the precise underlying mechanisms are not clearly understood, and effective therapies are currently limited.
Cycles of acetylation/deacetylation are fundamental modulators of biological activity. In particular, sirtuins are members of the class III nicotinamide adenine dinucleotide (NAD+)-dependent histone deacetylases (HDACs) that play diverse physiological roles. In mammals, there are seven sirtuins (SIRT1–SIRT7) with distinct subcellular localizations and substrates encompassing histones, transcription factors, and enzymatic activity (Fujita and Yamashita 2018). SIRT2 is primarily a cytosolic protein and is highly expressed in the central nervous system (CNS). SIRT2 regulates various biological processes including gene expression, nutrient metabolism, mitochondrial function, and aging (Harting and Knoll 2010). Compelling evidence indicates that SIRT2 accumulates in several neurodegenerative disorders. Moreover, SIRT2 inhibition or deficiency has beneficial and therapeutic effects in models of various neurological diseases, including Parkinson’s disease (PK) and Huntington’s disease (HD), which are linked to regulation of metabolism and cellular survival (Chopra et al. 2012; Harrison et al. 2018; Outeiro et al. 2007). Collectively, these findings indicate that inhibition of SIRT2 may provide a novel strategy for preventing anesthesia-induced neurotoxicity.
Substantial evidence implies a pathological role of SIRT2 in the regulation of inflammation. For example, SIRT2 is required for lipopolysaccharide (LPS)-induced neuroinflammation and microglial activation in mice (Wang et al. 2016). Moreover, SIRT2 knockdown in mice ameliorated LPS-induced infiltration of neutrophils and macrophages during acute tubular injury (Jung et al. 2015). Neuroinflammation contributes to anesthesia-induced neurotoxicity by promoting microglial activation and pro-inflammatory cytokine release (Shen et al. 2013). Microglial cells are resident phagocytic cells in the CNS that act as key modulators in the regulation of immune responses and pathophysiological processes. From a resting state, microglia adopts two extreme polarization states in response to microenvironmental stimuli, including deleterious M1 and neuroprotective M2 phenotypes. Those of M1 phenotype release pro-inflammatory cytokines, which are associated with secondary tissue injury, while those of M2 phenotype release anti-inflammatory cytokines, which are responsible for tissue repair. Pharmacological strategies targeting M1/M2 phenotypes of microglia could alleviate brain damage and improve neurological function recovery after injury (Franco and Fernandez-Suarez 2015).
Sevoflurane is an inhalational agent commonly used in the induction and maintenance of pediatric anesthesia. In the present study, we aimed to investigate whether sevoflurane would induce SIRT2 overexpression in the hippocampus of rat pups. Furthermore, we sought to validate whether SIRT2 downregulation via administration of the chemical inhibitor AK7 could exert anti-inflammatory and neuroprotective effects by modulating microglial polarization. We hypothesized that SIRT2 inhibition would exert anti-inflammatory effects via regulation of microglia M1/M2 polarization in anesthesia-induced neurotoxicity.
Materials and Methods
Animals
All animal experimental protocols were reviewed and approved by the Animal Ethics Committee of Shengjing Hospital, China Medical University, Shenyang, China (Approval Number: 2016 PS028K). A total of 110 male Sprague–Dawley rat pups were purchased from the Experimental Animal Center of China Medical University. Rat pups and their dams were housed individually with free access to food and water. The environment was controlled on a 12/12-h light/dark cycle at a temperature of 25 ± 2 °C. All efforts were made to minimize the number of rats used and their suffering.
The first set of pups (n = 15 animals per group) was randomly divided into two groups: CON (control) group and SEV (sevoflurane) group. In the first experiment, hippocampal tissues were obtained from the brains of five randomly selected pups from each group at each time point (6 h, 12 h, and 24 h after last gas exposure) and were prepared for western blotting assay. The second set of pups (n = 20 animals per group) was randomly assigned into four groups: CON (control plus vehicle) group, CON + AK7 (control plus AK7), SEV (sevoflurane plus vehicle), and SEV + AK7 (sevoflurane plus AK7). According to the consequences of the first experiment, in the second experiment, hippocampal tissues of five pups in each group were collected at 12 h after gas exposure, for western blotting analysis. For immunofluorescence analysis, five rat pups in each group were perfused transcardially with phosphate-buffered saline (PBS) followed by 4% paraformaldehyde. Brains were left overnight at 4 °C and embedded in paraffin after dehydration in graded ethanol. The ten remaining pups in each group were subjected to the open field and Morris water maze tests at P25 and P28–32, respectively. The experimental schedule is presented in Fig. 1a.
Sevoflurane Exposure and Drug Administration
On P7, rat pups were separated from their mothers and placed in an anesthetizing container with home cage bedding material. Rat pups in the sevoflurane groups received 3% sevoflurane plus 30% oxygen for 2 h daily for 3 consecutive days. Rat pups in the control groups only received 30% oxygen for 2 h daily for 3 consecutive days in a similar container. The total gas flow was 2 L/min. The rat pups breathed spontaneously. Anesthetic, oxygen, and carbon dioxide concentrations were measured continuously by a calibrated side stream analyzer. Rat pups were kept warm on a heating pad maintained at 37 ± 1 °C throughout the experiment. Previous studies have shown that sevoflurane exposure does not significantly affect the value of pH, arterial oxygen, and carbon dioxide tensions compared with those of the control group (Zhang et al. 2015). After termination of anesthesia, rat pups were placed back into the maternal cage when the righting reflex was recovered.
The potent and selective SIRT2 inhibitor, AK7, is able to cross the blood brain barrier (Taylor et al. 2011). AK7 was dissolved in 10% cyclodextrin in physiologic solution, as previously described (Biella et al. 2016). AK7 (20 mg/kg) or vehicle was intraperitoneally injected 30 min before each of the 3 days of sevoflurane exposure. An equal number of oxygen-exposed rats was used as controls and also received AK7 or vehicle.
Western Blotting
For total protein extraction, the hippocampus was homogenized in RIPA lysis buffer containing 1% protease inhibitor cocktail and then incubated on ice for 30 min. Samples were centrifuged at 14,000 rpm at 4 °C for 30 min, and the supernatants were collected. The hippocampus was subjected to western blotting analysis as described in our previous studies (Wu et al. 2018). Antibodies against SIRT2 (1:1000; Proteintech Biotechnology, Chicago, IL, USA), acetylated tubulin (1:1000; Proteintech Biotechnology, Chicago, IL, USA), TNF-α (1:500, Wanlei Biotechnology, Shenyang, China), IL-1β (1:300, Wanlei Biotechnology, Shenyang, China), iNOS (1:1000, Wanlei Biotechnology, Shenyang, China), MCP-1 (1:500, Wanlei Biotechnology, Shenyang, China), PPARγ (1:500, Wanlei Biotechnology, Shenyang, China), Arg1 (1:500, Wanlei Biotechnology, Shenyang, China), α-tubulin (1:2000; Proteintech Biotechnology, Chicago, IL, USA), and Gapdh (1:5000; Proteintech Biotechnology, Chicago, IL, USA) were used to detect SIRT2, acetylated tubulin, TNF-α, IL-1β, iNOS, MCP-1, PPARγ, Arg1, α-tubulin, and Gapdh expression, respectively. The protein bands were detected using enhanced chemiluminescence and quantitated using Image J software (NIH Image, Bethesda, USA).
Immunofluorescence
For immunofluorescence staining, brains were cut into 3-μm-thick coronal sections passing through the dorsal hippocampus and mounted on poly-l-lysine coated slides. After performing heat-mediated antigen retrieval with citrate buffer, sections were incubated in 10% fetal bovine serum for 40 min at room temperature and then incubated with rat monoclonal anti-Iba1 antibody (1:50; Proteintech Biotechnology, Chicago, IL, USA) overnight at 4 °C. After three washes in PBS, the sections were incubated with secondary antibody for 3–4 h at room temperature. After three washes in PBS, the sections were counterstained with DAPI for 5 min at room temperature. Fluorescence images of the slices were obtained using a Leica confocal fluorescence microscope (Leica TCS SP5 II, Heidelberg, Germany). Negative controls were incubated with PBS without primary antibody.
Open Field Test
On P25, rats were placed individually in the center of a black plastic chamber (100 cm × 100 cm × 50 cm) over a period of 10 min to evaluate locomotor and exploratory activities. Tests were recorded and analyzed by EthoVision XT software (Noldus, Wageningen, The Netherlands). After each test, the arena was cleaned with 75% alcohol to avoid the presence of olfactory cues.
Morris Water Maze Test
The Morris water maze (MWM) test was used to evaluate spatial learning and memory on days P28–32 and was performed as previously described with minor modifications (Wu et al. 2018). Briefly, the maze consisted of a round pool (painted black, 180 cm in diameter, 60 cm in height) filled with water heated to 22 ± 2 °C. The pool was geographically divided into four equal quadrants, designated as I, II, III, and IV. An escape platform (8 cm in diameter) was placed at the center of quadrant IV, approximately 1.5 cm below the water surface. Rats were subjected to four training sessions daily for five consecutive training days to locate the hidden escape platform. Each trial started from a different quadrant and is limited to 90 s. If the rat could find the platform within 90 s, the time from beginning to end was considered the escape latency. When the rat boarded the platform, it was kept on the platform for 20 s. In either case, the time of escape latency was recorded as 90 s and the rat was put onto the platform for 20 s. On day 6, the probe trail was performed by allowing the rat to swim for 90 s in the absence of the platform. The swimming time and trajectory of the rats were recorded by a Noldus Ethovision XT video analysis system (The Netherlands).
Statistical Analysis
All statistical tests were performed using GraphPad Prism Version 7.0 (GraphPad Prism Software, Inc., CA, USA). Data are presented as mean ± standard deviation (SD). The normality assumption test was performed using the Shapiro–Wilk test. If the assumption of normality was met, statistical analysis was performed using one-way analysis of variance (ANOVA) followed by Tukey post hoc multiple comparison tests. If the assumption of normality was violated, Kruskal–Wallis H test was used. The escape latency was tested by two-way repeated measures ANOVA followed by Dunnett’s multiple comparisons tests. P < 0.05 was regarded statistically significant.
Results
SIRT2 is Significantly Up-Regulated in Neonatal Rat Hippocampus After Repeated Exposure to Sevoflurane
In order to study the role of SIRT2 in the physiopathology of sevoflurane-induced damage to the immature brain, the expression of SIRT2 protein was measured in rat hippocampus homogenates. SIRT2 expression significantly increased at 6 h and peaked at 12 h post-anesthesia, followed by a reduction at 24 h post-anesthesia (Fig. 2).
Sevoflurane up-regulated SIRT2 expression in the rat hippocampus. a Representative western blotting images for the expression levels of SIRT2. b Quantification of SIRT2 normalized to Gapdh. n = 5 per group. Data are expressed as mean ± SD of control mean values. One-way ANOVA followed by Tukey post hoc multiple comparison tests was used for data analysis. **P < 0.01, compared with CON group (6 h); #P < 0.05, compared with CON group (12 h)
SIRT2 Inhibition Alleviates Sevoflurane-Induced Learning and Memory Impairments
To evaluate locomotor activity and anxiety-like behavior in a novel environment, rats underwent the open field test at P25. No differences were observed among groups in total distance traveled or time spent in the center region (Fig. 3a–c). These results suggested that neither sevoflurane nor AK-7 influenced locomotor activity or anxiety-like behavior in rats.
Effects of sevoflurane and AK7 on long-term neurobehaviour assessments of rats. a Representative traces of rat movement in the open field test. b Total distance traveled in the open field test. c Time spent in the center in the open field test. d Graphical representation of search patterns during MWM tests. e Escape latency to find the platform in the MWM tests. f Platform crossover in the target quadrant in the MWM tests. n = 10 per group. Data are expressed as mean ± SD. One-way ANOVA followed by Tukey post hoc multiple comparison tests was used for total distance traveled in the open field test. Kruskal–Wallis H test was used for time spent in the center in the open field test and platform crossover in the target quadrant in the MWM tests. Two-way repeated ANOVA followed by Dunnett’s multiple comparisons tests was used for escape latency. *P < 0.05, compared with CON group; **P < 0.01, compared with CON group; ***P < 0.001, compared with CON group; #P < 0.05, compared with SEV group; ###P < 0.001, compared with SEV group. MWM, Morris water maze
From P28, the MWM test was used to evaluate the influence of early-life sevoflurane exposure on long-term learning and memory ability. Rats in the sevoflurane group exhibited longer escape latency (Fig. 3d, e) and less platform crossings (Fig. 3d, f) than those in the control group. However, compared with that in the sevoflurane group, escape latency was decreased (Fig. 3d, e) and platform crossings were increased (Fig. 3d, f) with AK7 pretreatment. These data indicated that neonatal sevoflurane-induced spatial learning, and memory deficits were attenuated by AK7.
SIRT2 Inhibition Attenuates Activation of Microglia and Neuroinflammation Caused by Sevoflurane Exposure
α-tubulin is a well-known protein substrate for SIRT2 (North et al. 2003). Therefore, we detected the expressions of SIRT2 (Fig. 4b, c) and acetylated α-tubulin in each group (Supplementary Fig. 1a, b). There was no change of SIRT2 or acetylated α-tubulin protein between CON group and CON + AK7 group. Compared with CON group, SIRT2 expression significantly increased, while acetylated α-tubulin significantly decreased in SEV group. However, the expression of SIRT2 was inhibited while the expression of acetylated α-tubulin was up-regulated in SEV + AK7 group. Next, we assessed the effects of sevoflurane and AK7 on microglial responses and neuroinflammation in the rat hippocampus. Figure 4a shows representative photomicrographs of immunostaining with anti-Iba1 antibody in the hippocampus 12 h post-sevoflurane exposure from each group. In the sevoflurane group, strongly immunolabeled anti-Iba1-stained cells with highly branched processes were observed in the CA1 and CA3 areas of the rat hippocampus. These cells were consistent with activated microglia based on immunoreactivity and morphology. Immunoblotting for markers of inflammation, TNF-α and IL-1β, revealed that sevoflurane led to neuroinflammation, at least in the hippocampus (Fig. 4b, d, e). However, AK7 pretreatment decreased anti-Iba1-stained cells (Fig. 4a) and levels of TNF-α and IL-1β (Fig. 4b, d, e) in the hippocampus. Collectively, SIRT2 inhibition suppressed activation of microglia and neuroinflammation induced by sevoflurane.
AK7 inhibited activation of microglia and neuroinflammation caused by sevoflurane exposure. a Immunofluorescence staining for Iba1 (green) and DAPI (blue) in the hippocampus of rats. b Representative western blotting images for the expression levels of SIRT2, TNF-α, and IL-1β. c Quantification of SIRT2 normalized to Gapdh. d Quantification of TNF-α normalized to Gapdh. e Quantification of normalized to Gapdh. n = 5 per group. Data are expressed as mean ± SD of control mean values. One-way ANOVA followed by Tukey post hoc multiple comparison tests was used for data analysis. *P < 0.05, compared with CON group; #P < 0.05, compared with SEV group; ##P < 0.01, compared with SEV group
SIRT2 Inhibition Promotes Microglial M2 Polarization
To assess whether AK7 exerted anti-inflammatory activity in the brain by regulating microglial polarization, western blotting was performed to quantitatively assess M1 versus M2 microglial phenotypes. M1-related markers, iNOS and MCP-1, were up-regulated in sevoflurane-exposed rats (Fig. 5a–c). These effects were reversed by AK7 (Fig. 5a–c). M2-related markers, PPARγ and Arg1, were downregulated in the sevoflurane-exposed group (Fig. 5a–c) but up-regulated by AK7 (Fig. 5a–c). Collectively, AK7 induced activated M1 microglia to convert to an M2 neurotrophic phenotype.
AK7 promoted microglial M2 polarization. a Representative western blotting images for the expression levels of iNOS, MCP-1, PPARγ, and Arg1. b Quantification of iNOS normalized to Gapdh. c Quantification of MCP-1 normalized to Gapdh. d Quantification of PPARγ normalized to Gapdh. e Quantification of Arg1 normalized to Gapdh. n = 5 per group. Data are expressed as mean ± SD of control mean values. One-way ANOVA followed by Tukey post hoc multiple comparison tests was used for data analysis. *P < 0.05, compared with CON group; **P < 0.01, compared with CON group; ***P < 0.001, compared with CON group; #P < 0.05, compared with SEV group; ##P < 0.01, compared with SEV group
Discussion
In the current study, we demonstrated the involvement of SIRT2 in sevoflurane-induced learning and memory deficits in the developing rat brain. Multiple exposures to sevoflurane in neonatal rats resulted in elevated SIRT2 expression in the hippocampus at different time points. To evaluate the potential role of SIRT2, we used the pharmacological SIRT2 inhibitor, AK7. Our results showed that AK7 pretreatment protected against sevoflurane-mediated cognitive impairments and neuroinflammation in rats, possibly by enhancing the proportion of microglia with an M2-phenotype.
Histone acetyltransferases (HATs) and HDACs are antagonistic enzymes which modulate gene expression through acetylation and deacetylation of histone and non-histone proteins. It is well established that an imbalance between the actions of HATs/HDACs in the CNS triggers aberrant gene expression profiles and failure of neuronal homeostasis (Penney and Tsai 2014; Sen 2015). Activation of HATs or inhibition of HDACs has been proposed as a promising epigenetic therapeutic in anti-neurodegenerative agents (Ganai et al. 2016a, b). SIRT2 is an abundant deacetylase in the brain. Accumulation of SIRT2 in CNS has been implicated in aging and neurodegenerative diseases (Maxwell et al. 2011; Singh et al. 2017). Elevated expression levels of SIRT2 were detected in the rat brain at 6 h and 12 h after repeated exposure to sevoflurane in the present study. Genetic or pharmacologic downregulation of SIRT2 exerts beneficial effects in multiple neurological diseases, especially PD and HD. For instance, α-synuclein acts as a key mediator in PD pathogenesis; inhibition of SIRT2 in cellular and animal models of PD reduces α-synuclein-induced (Outeiro et al. 2007). Another example is that pharmacological inhibition of SIRT2 achieves optimal neuroprotective effects in cellular models of HD through negative regulation of sterol biosynthesis (Luthi-Carter et al. 2010). Additionally, SIRT2 inhibition-dependent protective effects have been described in hepatic ischemia–reperfusion injury (Wang et al. 2017), bacterial infections, noise-induced hearing loss (Liu et al. 2019), and lethal septic shock (Zhao et al. 2015). In line with these findings, our study provides the first evidence that the SIRT2 inhibitor AK7 attenuates impaired learning and memory caused by neonatal sevoflurane anesthesia in rats.
Neuroinflammation is strongly associated with cognitive impairments caused by anesthesia exposure in the developing, adult, and aged brain (Lee et al. 2015). Pro-inflammatory cytokines such as TNF-α and IL-1β are positive mediators of inflammation. Brief (approximately 1 h) exposure to isoflurane anesthesia significantly increased serum IL-1β in children undergoing MRI examination (Whitaker et al. 2017). Ketamine anesthesia can increase the levels of inflammatory cytokines TNF-α, IL-6, and IL-1β, which would lead to neuroinflammation in the hippocampus of adult mice (Li et al. 2017). Consistent with these reports, repeated sevoflurane exposure in neonatal rats induced elevated levels of TNF-α and IL-1β in the hippocampus. Pro-inflammatory cytokines are released by activated microglia, fueling neuroinflammation and leading to cognitive impairments. In turn, pro-inflammatory cytokines can give rise to microglial activation, leading to the production of the same pro-inflammatory cytokines. Therefore, besides pro-inflammatory cytokine accumulation, microglial activation is recognized as another hallmark of neuroinflammation. We used immunofluorescent labeling with an antibody against Iba1 to identify microglia. The numbers of Iba1-immunolabeled microglial cells were elevated in the CA1 and CA3 regions of the hippocampus.
Studies have shown that SIRT2 plays a vital role in regulating inflammatory processes. SIRT2 silencing produced reductions in the SIRT2 level can significantly decrease LPS-induced upregulation of pro-inflammatory cytokine (TNF-α and IL-6) mRNA in microglial BV2 cells (Chen et al. 2015). These in vitro observations suggested that SIRT2 may be a potential pharmacological target for inflammatory-related diseases. It is reported that pharmacological inhibition of SIRT2 by AGK2 suppressed LPS-induced neuroinflammation and brain damage in mice (Wang et al. 2016). Similarly, SIRT2 inhibitor AGK2 suppressed the inflammatory responses in acute liver failure mice by inhibition of mitogen-activated protein kinase and NF-κB signaling pathways (Jiao et al. 2019). In line with these, our results verified that SIRT2 inhibition by AK7 significantly inhibited microglial activation and reduced the levels of pro-inflammatory cytokines TNF-α and IL-1β in the hippocampus. These findings suggest that SIRT2 inhibition exerts anti-inflammatory actions on sevoflurane-exposed neonatal rat brains. However, some previous published work has suggested contrasting roles of SIRT2 in modulating inflammation. SIRT2 was identified a deacetylase of the transcription factor NF-κB subunit RelA (p65) (Rothgiesser et al. 2010). p65 is hyperacetylated in SIRT2 knockdown cells after TNF-α stimulation, which results in an increase in expression of a subset of NF-κB-dependent target genes (Rothgiesser et al. 2010). AK7 treatment exacerbated neuroinflammation in experimental traumatic brain injury both in vitro and in vivo (Yuan et al. 2016). SIRT2 knockout mice showed morphological changes in microglia and an increase in pro-inflammatory cytokine in brain when received LPS (Pais et al. 2013). Mechanically, SIRT2 deacetylated p65 and inhibited NF-κB-dependent transcription in microglia (Pais et al. 2013). Moreover, SIRT2 knockout mice developed more severe clinical and histological characteristics in dextran sulfate sodium-induced colitis model (Lo Sasso et al. 2014), showed exaggeration of microvascular inflammation with sepsis in the small intestine (Buechler et al. 2017), exhibited a more severe arthritic phenotype after collagen immunization (Lin et al. 2013), and promoted aging-related and angiotensin II-induced pathological cardiac hypertrophy (Tang et al. 2017). These data confirmed a protective role for SIRT2 against the development of inflammatory processes. Collectively, SIRT2 might have different effects on inflammation depending on the inflammatory model, organ, as well as signaling pathway, and the precise role and mechanisms of SIRT2 in biological processes warrant further investigation for the rational use of SIRT2 as a potential therapeutic target.
New trends in research are moving toward the theory that microglia not only function as resident CNS macrophages but also respond to environmental changes by secreting detrimental pro-inflammatory cytokines or beneficial anti-inflammatory cytokines that act on surrounding cells (Gehrmann et al. 1995). In this regard, activated microglia can be classified into two specific states: classically activated (M1) and alternatively activated (M2) subtypes. Briefly, the M1 phenotype is a pro-inflammatory cellular state associated with an overexpression of inflammatory cytokines, whereas M2 polarized microglia release beneficial mediators, inhibiting inflammation and restoring brain homeostasis. In this study, western blotting analysis indicated that SIRT2 inhibition hindered sevoflurane-induced M1 microglial activation by attenuating the gene expression of M1 markers (iNOS and MCP-1). In addition, the levels of M2 markers (PPARγ and Arg1) in the AK7 pretreatment group were up-regulated. Our results provide support for suppression of microglial M2 polarization by sevoflurane in vitro (Pei et al. 2017). Collectively, our findings suggested that sevoflurane facilitated microglial M1 polarization and inhibited microglial M2 polarization; these effects were reversed by SIRT2 inhibitor AK7.
The present study has several limitations. First, we did not examine other possible effects of SIRT2 inhibition such as its antioxidative capacity. Therefore, further investigations are needed to understand the contributions of these effects. Second, we did not clarify a definitive signaling pathway involved in the anti-inflammatory action of SIRT2 inhibition. Moreover, other brain regions related to learning and memory such as the amygdala and striatum should also be evaluated after AK7 administration.
Conclusions
In conclusion, our results highlight a novel role for SIRT2 inhibition in sevoflurane-induced learning and memory deficits in the immature brain of rats. At the molecular level, our findings reveal that these protective actions might be associated with a decrease in microglial activation and neuroinflammation. More importantly, our results provide compelling evidence that SIRT2 inhibition by AK7 confers protective action by regulating microglial M1/M2 polarization. Studies to investigate different substrate profiles of sirtuin family members and to design highly potent and specific pharmacological inhibitors or activators could lead to the development of more effective therapies for anesthesia neurotoxicity.
Abbreviations
- NAD:
-
Nicotinamide adenine dinucleotide
- HDACs:
-
Histone deacetylases
- CNS:
-
Central nervous system
- PK:
-
Parkinson’s disease
- HD:
-
Huntington’s disease
- LPS:
-
Lipopolysaccharides
- MWM:
-
Morris water maze
- HATs:
-
Histone acetyltransferases
References
Biella G, Fusco F, Nardo E, Bernocchi O, Colombo A, Lichtenthaler SF, Forloni G, Albani D (2016) Sirtuin 2 inhibition improves cognitive performance and acts on amyloid-beta protein precursor processing in two Alzheimer’s disease mouse models. J Alzheimer’s Dis 53(3):1193–1207. https://doi.org/10.3233/jad-151135
Bilotta F, Evered LA, Gruenbaum SE (2017) Neurotoxicity of anesthetic drugs: an update. Curr Opin Anaesthesiol 30(4):452–457. https://doi.org/10.1097/aco.0000000000000482
Buechler N, Wang X, Yoza BK, McCall CE, Vachharajani V (2017) Sirtuin 2 regulates microvascular inflammation during sepsis. J Immunol Res 2017:2648946. https://doi.org/10.1155/2017/2648946
Chen H, Wu D, Ding X, Ying W (2015) SIRT2 is required for lipopolysaccharide-induced activation of BV2 microglia. NeuroReport 26(2):88–93. https://doi.org/10.1097/wnr.0000000000000305
Chopra V, Quinti L, Kim J, Vollor L, Narayanan KL, Edgerly C, Cipicchio PM, Lauver MA, Choi SH, Silverman RB, Ferrante RJ, Hersch S, Kazantsev AG (2012) The sirtuin 2 inhibitor AK-7 is neuroprotective in Huntington’s disease mouse models. Cell Rep 2(6):1492–1497. https://doi.org/10.1016/j.celrep.2012.11.001
Franco R, Fernandez-Suarez D (2015) Alternatively activated microglia and macrophages in the central nervous system. Prog Neurobiol 131:65–86. https://doi.org/10.1016/j.pneurobio.2015.05.003
Fujita Y, Yamashita T (2018) Sirtuins in neuroendocrine regulation and neurological diseases. Front Neurosci 12:778. https://doi.org/10.3389/fnins.2018.00778
Ganai SA, Banday S, Farooq Z, Altaf M (2016a) Modulating epigenetic HAT activity for reinstating acetylation homeostasis: a promising therapeutic strategy for neurological disorders. Pharmacol Ther 166:106–122. https://doi.org/10.1016/j.pharmthera.2016.07.001
Ganai SA, Ramadoss M, Mahadevan V (2016b) Histone deacetylase (HDAC) inhibitors: emerging roles in neuronal memory, learning, synaptic plasticity and neural regeneration. Curr Neuropharmacol 14(1):55–71
Gehrmann J, Matsumoto Y, Kreutzberg GW (1995) Microglia: intrinsic immuneffector cell of the brain. Brain Res Rev 20(3):269–287
Harrison IF, Smith AD, Dexter DT (2018) Pathological histone acetylation in Parkinson’s disease: neuroprotection and inhibition of microglial activation through SIRT 2 inhibition. Neurosci Lett 666:48–57. https://doi.org/10.1016/j.neulet.2017.12.037
Harting K, Knoll B (2010) SIRT2-mediated protein deacetylation: an emerging key regulator in brain physiology and pathology. Eur J Cell Biol 89(2–3):262–269. https://doi.org/10.1016/j.ejcb.2009.11.006
Jiao FZ, Wang Y, Zhang WB, Zhang HY, Chen Q, Shi CX, Wang LW, Gong ZJ (2019) Protective role of AGK2 on thioacetamide-induced acute liver failure in mice. Life Sci. https://doi.org/10.1016/j.lfs.2019.05.061
Jung YJ, Lee AS, Nguyen-Thanh T, Kim D, Kang KP, Lee S, Park SK, Kim W (2015) SIRT2 regulates LPS-induced renal tubular CXCL2 and CCL2 expression. J Am Soc Nephrol 26(7):1549–1560. https://doi.org/10.1681/asn.2014030226
Lee YM, Song BC, Yeum KJ (2015) Impact of volatile anesthetics on oxidative stress and inflammation. Biomed Res Int 2015:242709. https://doi.org/10.1155/2015/242709
Li Y, Shen R, Wen G, Ding R, Du A, Zhou J, Dong Z, Ren X, Yao H, Zhao R, Zhang G, Lu Y, Wu X (2017) Effects of ketamine on levels of inflammatory cytokines IL-6, IL-1beta, and TNF-alpha in the hippocampus of mice following acute or chronic administration. Front Pharmacol 8:139. https://doi.org/10.3389/fphar.2017.00139
Lin J, Sun B, Jiang C, Hong H, Zheng Y (2013) Sirt2 suppresses inflammatory responses in collagen-induced arthritis. Biochem Biophys Res Commun 441(4):897–903. https://doi.org/10.1016/j.bbrc.2013.10.153
Liu Y, Ao L, Li Y, Zhao Y, Wen Y, Ding H (2019) The SIRT2 inhibitor AK-7 decreases cochlear cell apoptosis and attenuates noise-induced hearing loss. Biochem Biophys Res Commun 509(3):641–646. https://doi.org/10.1016/j.bbrc.2018.12.084
Lo Sasso G, Menzies KJ, Mottis A, Piersigilli A, Perino A, Yamamoto H, Schoonjans K, Auwerx J (2014) SIRT2 deficiency modulates macrophage polarization and susceptibility to experimental colitis. PLoS ONE 9(7):e103573. https://doi.org/10.1371/journal.pone.0103573
Luthi-Carter R, Taylor DM, Pallos J, Lambert E, Amore A, Parker A, Moffitt H, Smith DL, Runne H, Gokce O, Kuhn A, Xiang Z, Maxwell MM, Reeves SA, Bates GP, Neri C, Thompson LM, Marsh JL, Kazantsev AG (2010) SIRT2 inhibition achieves neuroprotection by decreasing sterol biosynthesis. Proc Natl Acad Sci USA 107(17):7927–7932. https://doi.org/10.1073/pnas.1002924107
Maxwell MM, Tomkinson EM, Nobles J, Wizeman JW, Amore AM, Quinti L, Chopra V, Hersch SM, Kazantsev AG (2011) The Sirtuin 2 microtubule deacetylase is an abundant neuronal protein that accumulates in the aging CNS. Hum Mol Genet 20(20):3986–3996. https://doi.org/10.1093/hmg/ddr326
North BJ, Marshall BL, Borra MT, Denu JM, Verdin E (2003) The human Sir2 ortholog, SIRT2, is an NAD+-dependent tubulin deacetylase. Mol Cell 11(2):437–444
Outeiro TF, Kontopoulos E, Altmann SM, Kufareva I, Strathearn KE, Amore AM, Volk CB, Maxwell MM, Rochet JC, McLean PJ, Young AB, Abagyan R, Feany MB, Hyman BT, Kazantsev AG (2007) Sirtuin 2 inhibitors rescue alpha-synuclein-mediated toxicity in models of Parkinson’s disease. Science 317(5837):516–519. https://doi.org/10.1126/science.1143780
Pais TF, Szego EM, Marques O, Miller-Fleming L, Antas P, Guerreiro P, de Oliveira RM, Kasapoglu B, Outeiro TF (2013) The NAD-dependent deacetylase sirtuin 2 is a suppressor of microglial activation and brain inflammation. EMBO J 32(19):2603–2616. https://doi.org/10.1038/emboj.2013.200
Pei Z, Wang S, Li Q (2017) Sevoflurane suppresses microglial M2 polarization. Neurosci Lett 655:160–165. https://doi.org/10.1016/j.neulet.2017.07.001
Penney J, Tsai LH (2014) Histone deacetylases in memory and cognition. Science signaling 7(355):re12. https://doi.org/10.1126/scisignal.aaa0069
Rothgiesser KM, Erener S, Waibel S, Luscher B, Hottiger MO (2010) SIRT2 regulates NF-kappaB dependent gene expression through deacetylation of p65 Lys310. J Cell Sci 123(Pt 24):4251–4258. https://doi.org/10.1242/jcs.073783
Satomoto M, Makita K (2016) Anesthesia-induced neurotoxicity in an animal model of the developing brain: mechanism and therapies. Neural Regen Res 11(9):1407–1408. https://doi.org/10.4103/1673-5374.191207
Sen N (2015) Epigenetic regulation of memory by acetylation and methylation of chromatin: implications in neurological disorders, aging, and addiction. NeuroMol Med 17(2):97–110. https://doi.org/10.1007/s12017-014-8306-x
Shen X, Dong Y, Xu Z, Wang H, Miao C, Soriano SG, Sun D, Baxter MG, Zhang Y, Xie Z (2013) Selective anesthesia-induced neuroinflammation in developing mouse brain and cognitive impairment. Anesthesiology 118(3):502–515. https://doi.org/10.1097/ALN.0b013e3182834d77
Singh P, Hanson PS, Morris CM (2017) Sirtuin-2 protects neural cells from oxidative stress and is elevated in neurodegeneration. Parkinson’s Dis 2017:2643587. https://doi.org/10.1155/2017/2643587
Tang X, Chen XF, Wang NY, Wang XM, Liang ST, Zheng W, Lu YB, Zhao X, Hao DL, Zhang ZQ, Zou MH, Liu DP, Chen HZ (2017) SIRT2 acts as a cardioprotective deacetylase in pathological cardiac hypertrophy. Circulation 136(21):2051–2067. https://doi.org/10.1161/circulationaha.117.028728
Taylor DM, Balabadra U, Xiang Z, Woodman B, Meade S, Amore A, Maxwell MM, Reeves S, Bates GP, Luthi-Carter R, Lowden PA, Kazantsev AG (2011) A brain-permeable small molecule reduces neuronal cholesterol by inhibiting activity of sirtuin 2 deacetylase. ACS Chem Biol 6(6):540–546. https://doi.org/10.1021/cb100376q
Wang B, Zhang Y, Cao W, Wei X, Chen J, Ying W (2016) SIRT2 plays significant roles in lipopolysaccharides-induced neuroinflammation and brain injury in mice. Neurochem Res 41(9):2490–2500. https://doi.org/10.1007/s11064-016-1981-2
Wang J, Koh HW, Zhou L, Bae UJ, Lee HS, Bang IH, Ka SO, Oh SH, Bae EJ, Park BH (2017) Sirtuin 2 aggravates postischemic liver injury by deacetylating mitogen-activated protein kinase phosphatase-1. Hepatology 65(1):225–236. https://doi.org/10.1002/hep.28777
Whitaker EE, Christofi FL, Quinn KM, Wiemann BZ, Xia JC, Tobias JD, Bissonnette B (2017) Selective induction of IL-1beta after a brief isoflurane anesthetic in children undergoing MRI examination. J Anesth 31(2):219–224. https://doi.org/10.1007/s00540-016-2294-y
Wu Z, Li X, Zhang Y, Tong D, Wang L, Zhao P (2018) Effects of sevoflurane exposure during mid-pregnancy on learning and memory in offspring rats: beneficial effects of maternal exercise. Front Cell Neurosci 12:122. https://doi.org/10.3389/fncel.2018.00122
Yuan F, Xu ZM, Lu LY, Nie H, Ding J, Ying WH, Tian HL (2016) SIRT2 inhibition exacerbates neuroinflammation and blood-brain barrier disruption in experimental traumatic brain injury by enhancing NF-kappaB p65 acetylation and activation. J Neurochem 136(3):581–593. https://doi.org/10.1111/jnc.13423
Zhang MQ, Ji MH, Zhao QS, Jia M, Qiu LL, Yang JJ, Peng YG, Yang JJ, Martynyuk AE (2015) Neurobehavioural abnormalities induced by repeated exposure of neonatal rats to sevoflurane can be aggravated by social isolation and enrichment deprivation initiated after exposure to the anaesthetic. Br J Anaesth 115(5):752–760. https://doi.org/10.1093/bja/aev339
Zhao T, Alam HB, Liu B, Bronson RT, Nikolian VC, Wu E, Chong W, Li Y (2015) Selective inhibition of SIRT2 improves outcomes in a lethal septic model. Curr Mol Med 15(7):634–641
Funding
This work was supported by the National Nature Science Foundation of China (Nos. 81870838, 81671311), the Key Research and Development Program of Liaoning Province (No. 2018225004), and the Outstanding Scientific Fund of Shengjing Hospital (No. 201708).
Author information
Authors and Affiliations
Contributions
ZW and PZ conceived and designed experiments. ZW, YZ (Yi Zhang) and YZ (Yinong Zhang) performed experiments, generated and analyzed the data. ZW wrote the manuscript with the help of PZ. All authors read and approved the final manuscript.
Corresponding author
Ethics declarations
Conflict of interest
The authors declare that they have no conflict of interest.
Ethical Approval
All applicable international, national, and/or institutional guidelines for the care and use of animals were followed. All procedures performed in studies involving animals were in accordance with the ethical standards of the Animal Ethics Committee of Shengjing Hospital of China Medical University, where these studies were conducted. This article does not contain any studies with human participants performed by any of the authors.
Additional information
Publisher's Note
Springer Nature remains neutral with regard to jurisdictional claims in published maps and institutional affiliations.
Electronic supplementary material
Below is the link to the electronic supplementary material.

Supplementary Fig.
1 Expressions of acetylated α-tubulin in the hippocampus of rats in each group. (a) Representative western blotting images for the expression levels of acetylated α-tubulin. (b) Quantification of acetylated α-tubulin normalized to α-tubulin. n = 5 per group. Data are expressed as mean ± SD of control mean values. One-way ANOVA followed by Tukey post hoc multiple comparison tests was used for data analysis. **P < 0.01, compared with CON group; ##P < 0.01, compared with SEV group. Supplementary material 1 (JPEG 172 kb)
Rights and permissions
About this article
Cite this article
Wu, Z., Zhang, Y., Zhang, Y. et al. Sirtuin 2 Inhibition Attenuates Sevoflurane-Induced Learning and Memory Deficits in Developing Rats via Modulating Microglial Activation. Cell Mol Neurobiol 40, 437–446 (2020). https://doi.org/10.1007/s10571-019-00746-9
Received:
Accepted:
Published:
Issue Date:
DOI: https://doi.org/10.1007/s10571-019-00746-9