Abstract
A new voltammetric method without using high cost and health risk nanomaterials has been developed for quantitative determination of ethylvanillin and methylvanillin sum, compounds that are used as food additives. The method is based on direct electrochemical oxidation of these biologically active compounds using square wave voltammetry at carbon paste electrode with surface modified by sodium dodecyl sulfate (SDS/CPE) performed in 0.1 M phosphate pH 6.0 buffer. Working conditions such as pH value of supporting electrolyte, type of surfactant, accumulation time of surfactant, and parameters of square wave voltammetry were optimized. In comparison with bare carbon paste electrode, excellent reproducibility characterized by a relative standard deviation of approximately 0.3% was obtained at the SDS/CPE. Linear range from 1.0 × 10−6 to 2.0 × 10−5 M, limits of quantification 9.8 × 10−8 M and detection 2.9 × 10−8 M were found at pulse amplitude 70 mV and frequency 50 Hz selected as optimum for ethylvanillin quantification. For methylvanillin, a linear range from 7.0 × 10−8 to 2.0 × 10−5 M and limits of quantification 7.0 × 10−8 M and detection 2.0 × 10−8 M were also determined. The procedure was validated using standard high-performance liquid chromatography method in the analysis of selected complex foodstuffs such as commercial baking sugar, biscuits, and an alcoholic drink. The results showed that a direct voltammetric approach is economically advantageous and reliable for the determination of ethyl- and methylvanillin, which is fully comparable to the reverse phase HPLC used as the ISO standard.
Graphical abstract

Similar content being viewed by others
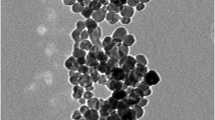
Explore related subjects
Discover the latest articles, news and stories from top researchers in related subjects.Avoid common mistakes on your manuscript.
Introduction
As known, vanillin (resp., methylvanillin, MVA), chemically 3-methoxy-4-hydroxybenzaldehyde (Fig. 1a), represents the main volatile component of the essential oil contained in natural vanilla, especially in Vanilla planifolia, Vanilla pompona, and Vanilla tahitiensis. Its content in dried vanilla pods varies from 1.5 to 3%. Due to its high price, it is also synthetically produced; the product is about 500 times cheaper than the natural one, but also less tasty. In contrast, synthetically prepared ethylvanillin (EVA), chemically 3-ethoxy-4-hydroxybenzaldehyde (Fig. 1b), is—as a flavoring agent—roughly three times stronger than MVA. For these reasons, natural vanillin is mostly replaced by synthetic EVA prepared by chemical or biochemical processes [1].
The desirable flavor and aroma properties of both MVA and EVA have led to their widespread application in food technology, pharmacies, and perfumes. Moreover, it was found that MVA has many beneficial health properties such as inhibition of the oxidation of human low density lipoproteins which causes lower rates of cardiac disease mortality [2] as well as an antisickling effect in sickle cell anemia sufferers [3]. It can be assumed that EVA has identical biological activity like MVA due to their very close molecular structures. On the other hand, it should be noted that very high periodical intake of synthetic MVA and EVA can lead to headaches, nausea, and vomiting. A toxic effect on liver and kidney was also described [4].
Evidently, determination of MVA and EVA as food additives is very desirable. Several analytical methods have been proposed for the determination of both compounds in various food samples. For example, high performance liquid chromatography (HPLC) is declared as standard method in the EU legislatives – ISO 5565 2:1999(en). As other methods, gas chromatography [5], thin layer chromatography [6], capillary electrophoresis [7], UV Vis spectrophotometry [8], and chemiluminescence [9] can be also used.
It is also important to mention electrochemical approaches. In this case, numerous voltammetric methods have been developed to determine the MVA only (see Table 1). These procedures offer some valuable advantages like economically available instrumentation, manipulation with small volumes, high sensitivity, and rapid analysis. However, it should be emphasized that both compounds differ structurally only by similar substituents (methyl in MVA, ethyl in EVA) which do not affect their electrochemical behavior. Thus, voltammetry is unable to distinguish these compounds and their content is therefore expressed as a mass equivalent of EVA. Furthermore, it is also important to note that their direct electrochemical oxidation on bare working electrodes lacks the required reproducibility, probably influenced by non-specific adsorption of oxidation products. It is the reason why electrode surfaces are often modified, which seems to be an excellent way to overcome this obstacle [10,11,12,13,14,15,16,17]. Such sophisticated electrochemical sensors are usually based on composite electrode materials [18] or on a glassy carbon electrode modified with various kinds of nanomaterials, in particular graphene, nanotubes, metal nanoparticles or their combinations.
This paper describes the development of the carbon paste electrode modified by thin film of sodium dodecyl sulfate (SDS/CPE) and its applications for the sensitive voltammetric determination of the sum of MVA and EVA in selected food samples. A simple modification was applied for this purpose, based on the immersion of unmodified CPE into an aqueous solution of this surfactant. Compared to the previously described sensors, the SDS/CPE provided significantly better analytical parameters. The results of this developed electrochemical method were compared to the standard reference method based on reverse phase HPLC with UV detection.
Results and discussion
Carbon paste electrode (CPE) represents a typical heterogeneous electroanalytical sensor composed of conductive graphite powder and lipophilic binder [19] which can be widely applicable in voltammetric determination of biologically active organic compounds [20]. Its field of application can be extended using simple surface modification by surfactants [21]. The principle is based on extraction of the surfactant nonpolar alkyl chain into the lipophilic binder of CPE. This leads to modification of the electrode surface by functional group of surfactants used. In this way, a nonpolar electrode surface is changed to the highly polar one [22]. Usually, significant enhancement in the sensitivity and stability of current response can be achieved.
Electrochemical behaviour of ethylvanillin
Surprisingly, all scientific papers are focused on study of electrochemical behaviour and voltammetric determination of MVA only, despite that EVA is also a significantly occurring additive in common foodstuffs. From a chemical point of view, MVA and EVA are considered p-hydroxybenzaldehyde differing by methoxy groups in their ortho positions. Using CV at SDS/CPE, it was found that this difference does not have any great impact on their electrochemical oxidation pathways, but have evident effect on their peak current responses (Fig. 2). It was observed that MVA provides higher peak current response than EVA for the same concentrations. This phenomenon can be probably explained by slower kinetics of EVA electrode reaction.
In the first scan, both compounds provide only one sensitive oxidation signal at + 0.66 V in PBS of pH 6.0 which corresponds to 2e− and 2H+ transfer with nucleophilic addition of water and sequent release of appropriate alcohol, namely methanol (MVA) and ethanol (EVA). In subsequent scans, less sensitive redox couple (o-quinone/catechol) is produced [16, 23]. According to this finding (Fig. 3), it is necessary to state that it is impossible to recognize the MVA from EVA by voltammetric techniques. Therefore, only sum of these compounds can be determined in foodstuffs.
For comparison, an increasing of oxidation peak heights at + 0.62 V after each repetition was observed within separate repetitive CV of EVA and MVA at bare CPE. This phenomenon probably caused by polymerization reaction forming a polyvanillin [24] was partially suppressed by the presence of surfactant. Mentioned polarization caused significant deterioration in repeatability. It can be therefore assumed that with certainty, a better repeatability of measurements will be achieved on the modified electrodes in the sequently used square wave voltammetry (SWV).
Values 20, 40, 60, 80, and 100 mV s−1 were set under a scan rate study of EVA. It was found that oxidation peak current linearly (R2 = 0.9950) increased with the square root of the scan rate (ν). Moreover, slope (k) value 0.5424 for linear dependency (R2 = 0.9967) of the peak current logarithm on log ν was calculated. For this finding, diffusion-controlled electrochemical oxidation reaction can be accepted. Due to very similar chemical structure of MVA, the same behaviour can be with certainty predicted.
Effect of surfactant
Three different types of surfactants such as anionic sodium dodecyl sulfate (SDS), cationic cetylpyridinium chloride (CPC), and nonionic Triton X-100 were tested within optimization. At first glance, it was evident that nonionic surfactant chosen is not a suitable variant, because a very high background current (~ 45 µA) was observed. In accordance with Fig. 4, it seems that SDS and CPC could be used as possible surface modifiers; however, significantly higher current response was obtained with anionic surfactant. For that reason, immersing CPE into 3.0 × 10−4 M SDS (Fig. 4a) aqueous solution for 4 min (Fig. 4b) was chosen as the optimum condition of modification.
a Dependence of peak current response (2.0 × 10−5 M EVA) on surfactant concentration (accumulated on CPE surface at 400 rpm for 5 min), measured in 0.1 M PBS pH 6 using SWV at potential amplitude 25 mV and frequency 10 Hz. b Dependence of peak current response (2.0 × 10−5 M EVA) on accumulation time of surfactant (accumulated on CPE surface from 3.0 × 10−4 M SDS and 2.0 × 10−4 M CPC at 400 rpm), measured in 0.1 M PBS pH 6 using SWV at potential amplitude 25 mV and frequency 10 Hz
Electrochemical detection of ethylvanillin and methylvanillin
Square wave voltammetry (SWV) of EVA (also MVA) provided only one oxidation signal in Britton–Robinson buffers within the pH 2–11 at the first anodic scan. Secondary anodic signal was observed in repeated measurements on the same SDS/CPE surface. For pH range from 2 to 8, the slope of the dependence of peak potentials vs. pH has a value of − 56.7 mV, which is close to the Nernstian value indicating the exchange of the same numbers of protons and electrons. Constant values of peak current were measured for higher value of pH 8. The highest value of peak current was obtained in the neutral medium. The same peak current was found also for 0.1 M PBS of pH 6, and for that reason was chosen as optimum.
The optimization of detection using SWV focused on finding the proper pulse amplitude and frequency, which affect the peak current significantly. Setting amplitude more than 70 mV did not result in increased peak heights. A similar behaviour was found for the effect of frequency higher than 50 Hz. These two parameters were used as optimal to achieve the most sensitive voltammetric method. Nevertheless, it is necessary to note that this leads to a significant shortening of the linear range. For example, linear ranges from 1.0 × 10−6 to 2.0 × 10−5 M for optimum conditions and from 5.0 × 10−6 to 1.0 × 10−4 M for pulse amplitude 20 mV and frequency 10 Hz were achieved, respectively. Figure 5 shows typical voltammograms with corresponding calibration curve. Limits of detection (LOD) and quantification (LOQ) were calculated according to the already known equations LOD = 3 s/k and LOQ = 10 s/k, respectively, where s is the standard deviation of measurement of 1.0 × 10−6 M EVA (N = 10) and k is the slope of corresponding calibration curve Ip = 0.435c − 0.095 with coefficient of determination R2 = 0.9965. Values 3.0 × 10−8 M and 1.0 × 10−7 M EVA for LOD and LOQ were calculated, respectively. For MVA, a calibration curve described by the equation Ip = 2.52c − 0.432 with coefficient of determination R2 = 0.9958 for linear range from 7.0 × 10−8 to 2.0 × 10−5 M and value of LOD 2.0 × 10−8 M were also determined. Final results are summarized together with those of previously published voltammetric methods dominantly focused on the determination of MVA (see Table 1). Except one publication [25], all other contributions have been focused on direct voltammetric determination of MVA, but it was not taken into account that the EVA is contained in higher quantities in many cases. This is one of the many reasons why this work has been arisen.
The main advantage can be surely considered as the very simple and cheap process of SDS/CPE preparation. Only 4 min are needed for CPE modification. Because of this, each analysis can be performed at freshly prepared working electrode, and thus eliminate the negative memory effect. The developed method has comparable analytical parameters such as previously reported approaches which are dominantly based on utilizing high cost and potential health risk nanomaterials.
Reproducibility and selectivity
Stability of peak current response of 1.0 × 10−5 M EVA for 5 repetitions was tested at the same surface of SDS/CPE, bare CPE, and at always fresh surface of bare CPE. Relevant voltammograms are shown in Fig. 6. Notwithstanding significantly lower current response at SDS/CPE, the excellent reproducibility about 0.3% relative standard deviation (RSD) was obtained. Value of 5.8% RSD was obtained for measurements at always freshly prepared CPE surface. Unlike this, the worst reproducibility 6.6% RSD for measurements at the same surface of CPE was found. Recovery of developed voltammetric method was calculated from analysis of model sample (2.0 × 10−5 M EVA) by standard addition method (5.0 × 10−6 M EVA). Value of 99.2% was obtained at SDS/CPE.
Selectivity of each voltammetric method is generally affected by presence of accompanying substances in the sample which can be electrochemically oxidized as analyte at the same voltage or chemically react with it. Generally, EVA and MVA are used in the food technology to replace an expensive vanilla extract. Because of their typical flavor, they widely occur in sweet kind of foodstuffs. Glucose, sucrose, starch, and ascorbic acid were therefore tested as possible interfering substances. In this study, a model sample containing 5.0 × 10−5 M EVA with constant 1.0 × 10−3 M of each sugar was measured. It was found that the presence of sugars slightly caused an increase in current response by 10%. In contrast with this, ascorbic acid significantly increased peak current due to the very similar oxidation potential. Nearly 30% increase was obtained when two times higher content of ascorbic acid was used. In addition, electroanalysis of completely different foodstuffs confirmed that any other interference, especially with different vitamins or minerals have not occurred.
Analysis of selected foodstuffs
Quantification of EVA and MVA sum in the foodstuff samples was carried out by standard addition method due to low and negative intercept value (q) of calibration curve − 0.095 µA. In comparison with a method of calibration curve, it should be generally known that results obtained from an analysis with the participation of sample matrix are often more accurate. Typical record obtained during voltammetric analysis of Absolut vanilla vodka is shown in Fig. 7. The corresponding electrochemical measurements were repeated minimally three times. Evaluated results have correlation coefficients higher than 0.9970 (see inset of Fig. 7).
Three examples of commercially available foodstuffs in Czech stores (Table 2) were selected as suitable samples for validation of developed electroanalytical method. Due to mutual presence of MVA and EVA in these samples, the sum of these compounds had to be expressed as mg of EVA in 100 g (or in 100 cm3 for fluids). Unfortunately, declared amounts were not listed because they are used as food ingredients. For that reason, the results obtained from direct SWV measurements of foodstuffs on the content of EVA were compared with those obtained by the reference standard HPLC analyses (ISO 5565 2:1999), where the total amount of EVA was calculated as a sum of contributions from EVA and MVA. Due to analysis of only three samples (N = 3), normality test, ANOVA or t test did not need to be applied. In this case, calculation of the recovery (%) for model sample was found to be sufficient, namely 97.5% for HPLC. A typical chromatogram of (type of foodstuff) is shown in Fig. 8. Satisfactory accordance among these fundamentally different analytical protocols only confirms the fact that the developed method can be also used in routine food analysis.
HPLC analysis of 10 mg dm−3 standards (solid), baking sugar (dashed), alcoholic drink (dotted line), and biscuit (insert). Ascentis Express C18 (150 × 3 mm, 2.7 μm) core-shell column (Supelco), mobile phase: acetonitrile/water (3:7), flow rate 0.4 cm3 min−1, sample volume 1 mm3, temperature 35 °C, detection at 310 nm
Conclusion
In this paper, a completely new electroanalytical method for sum of EVA and MVA determination in different foodstuffs was developed. This method is based on direct voltammetric oxidation of EVA and MVA at SDS/CPE performed in 0.1 M PBS of pH 6.0. Presence of sodium dodecyl sulfate on CPE surface causes excellent reproducibility of the measurement because it prevents negative passivation of the electrode surface. It is also necessary to conclude that SDS/CPE represents a very cheap way than the existing voltammetric methods utilizing different nanomaterials. In the comparison with the standard HPLC method, the electrochemical approach offers significant benefits. For example, lower consumption of organic solvents, easier sampling preparation, shorter time of analysis, and more simple instrumentation can be considered. It can be assumed that EVA has identical biological activity like MVA due to their very close molecular structures.
Experimental
Reagents
Analytical standards of MVA and EVA originating from Sigma-Aldrich (St. Louis, MI, USA.) were used to prepare their 0.01 M stock solutions in 50% ethanol. Sodium dodecyl sulfate, cetylpyridinium chloride, and Triton X-100 purchased from the same company were tested as surface modifiers. Other reagents needed for preparation of supporting electrolytes such as 0.1 M Britton–Robinson buffer (boric acid, 98% glacial acetic acid, 85% phosphoric acid, and sodium hydroxide) and 0.1 M phosphate buffer of pH 6 (KH2PO4 and Na2HPO4·12·H2O) were from Lach-Ner, s.r.o. (Neratovice, Czech Republic). The stock solutions for HPLC were prepared in 1:1 aqueous methanol; diluting with the mobile phase provided the working standard solutions with adequate response of the UV detector (approximately 0.5–100 mg dm−3). Demineralized water treated with a SG Ultra Clear UV water purification system (SG, Hamburg, Germany) with electric resistivity > 18.3 MΩ cm was used for preparing all the mentioned solutions.
Preparation of modified carbon paste electrode
Unmodified carbon paste electrode (CPE) was prepared by mixing 0.4 g graphite powder type CR-5 (particle size ~ 5 µm) from Maziva (Týn nad Vltavou, Czech Republic) with 0.1 g silicon oil (SO) type MV 8000 from Lučební závody (Kolín, Czech Republic) in a ceramic mortar for 10 min. Chemically, SO is polydimethylsiloxane of low viscosity and is used commonly as pasting liquid. Resulting homogeneous carbon paste was packed into the cavity of Teflon® piston holder. Freshly prepared CPE should not be employed generally in any experiments due to their rather unstable electrochemical behaviour caused by an incomplete homogenization, especially when SO was used. Fresh CPE has therefore been ripened in laboratory conditions for 1 day. As described previously [42], CPEs can be used for following handling only when they succumb to such self-homogenization process. Smoothing of the electrode surface with a damp filter paper and rinsing with demineralized water were subsequently done. CPE was modified with a thin layer of surfactant by immersing into 10 cm3 of 3.0 × 10−4 M SDS at 400 rpm of the magnetic stir bar for 4 min.
Apparatus
All electrochemical measurements were carried out in a 50 cm3 glass cell at laboratory conditions. Typical three-electrode system consisting the SDS/CPE (working), Ag/AgCl/3.0 M KCl (reference), and platinum sheet (auxiliary) electrodes connected to potentiostat Autolab PGSTAT101 (Metrohm) compatible with software Nova version 1.11 was used. All potentials are referred to the reference electrode mentioned above.
Sampling
Three different kinds of foodstuffs such as vanilla sugar for baking (Castelo) available in Lidl Czech Republic v.o.s., vanilla biscuits (Opavia), and alcoholic drink (Absolut Vanilia) from Tesco Stores Czech Republic a.s. were chosen as illustrative samples for analysis. A sample of vanilla sugar was prepared by dissolving 1.0 g of the product in a 25 cm3 volumetric flask using 0.1 M phosphate pH 6 buffer (PBS) containing 10% ethanol (v/v). After that, 2 cm3 of this sample solution was added to 18 cm3 PBS and analyzed by the standard addition method (three consecutive additions of 100 mm3 EVA stock solution). For biscuits, usually four pieces were crushed in a ceramic mortar for 10 min. Then, 10 g of the resulting mixture was transferred to a 100 cm3 volumetric flask, filled with PBS and sonicated for 30 min. The final extract was filtered through a vacuum pump due to the presence of the fiber causing the clogging of the filter paper. Finally, 20 cm3 of this filtrate was analyzed as in the previous case. Ethanol beverage analysis using a voltammetric method did not require any sophisticated sampling procedure. In this case, only 0.5 cm3 of the sample was added to 19.5 cm3 of PBS and analyzed by the above-mentioned protocol.
Measurements
Electrochemical behaviour of 5.0 × 10−4 M EVA at bare CPE and SDS/CPE in different supporting electrolytes was compared with that for MVA by cycling voltammetry (CV) from − 0.2 to + 1.0 V at potential step 5 mV, scan rate 50 mV s−1, and for minimally 3 repetitions. Voltammetric determination of EVA in foodstuffs selected was carried out by square wave voltammetry (SWV) at following conditions: potential window from 0 to + 1.0 V, potential step 5 mV, potential amplitude 70 mV, and frequency 50 Hz. Each analysis was minimally ten times repeated and final results are therefore shown as arithmetic means. Otherwise, all fundamental changes in the experimental parameters described above are specified below, in the legends of the corresponding figures.
Standard reference method
The sample of alcoholic drink was placed into ultrasonic bath for 10 min and injected into the HPLC system without any dilution. Approximately, 1 g of vanilla sugar sample was dissolved in 50 cm3 aqueous methanol (1:1) and placed into ultrasonic bath for a period of 10 min. Approximately, 2 g of biscuit sample was dissolved in 50 cm3 aqueous methanol (1:1) and placed into ultrasonic bath for a period of 10 min. All samples were filtered through 0.45 μm membrane filter before HPLC analysis.
An Agilent 1290 Infinity liquid chromatograph (Agilent, Palo Alto, CA, USA) equipped with a binary pump, a degasser, an auto-sampler, a diode-array UV detector and a thermostated column compartment was used. The DAD-UV detector signal was monitored at 310 nm and full UV spectra were recorded for peak identification. An Ascentis Express C18 (2.7 µm, 150 × 3.0 mm) core-shell column (Supelco, Bellafonte, PA, USA) was used for separation with a flow rate 0.4 cm3 min−1. All chromatographic separations were carried out at 40 °C and 1 mm3 injection volume was used in all analyses. Separation was achieved using an isocratic elution of 30% acetonitrile and 70% water.
References
Walton NJ, Mayer MJ, Narbad A (2003) Phytochemistry 63:505
Teissedre PL, Waterhouse AL (2000) J Agric Food Chem 48:3801
Farthing D, Sica D, Abernathy C, Fakhry I, Roberts JD, Abraham DJ (1999) J Chromatogr B 726:303
Han XS (2002) Sichuan Chem indust Erod Control 5:36
Perez-Silva A, Odoux E, Brat P, Ribeyre F, Rodriguez-Jimenes G, Robles-Olvera V (2006) Food Chem 99:728
Gerasimov AV, Gornova NV, Rudometova NV (2003) J Anal Chem 58:677
Ohashi M, Omae H, Hashida M, Sowa Y, Imai S (2007) J Chromatogr A 1138:262
Longares-Patron A, Canizares-Macias MP (2006) Talanta 69:882
Timotheou-Potamia M, Calokerinos AC (2007) Talanta 71:208
Bettazzi F, Palchetti I, Sisalli S, Mascini M (2006) Anal Chim Acta 555:134
Kong DJ, Shen SF, Yu HY, Wang JD, Chen NS (2010) Chin J Inorg Chem 26:817
Luque M, Luque-Perez E, Rios A, Valcarcel M (2000) Anal Chim Acta 410:127
Peng J, Hou C, Hu X (2012) Int J Electrochem Sci 7:1724
Shang L, Zhao F, Zeng B (2014) Food Chem 151:53
Zheng D, Hu C, Gan T, Dang X, Hu S (2010) Sens Actuators B Chem 148:247
Deng P, Xu Z, Zeng R, Ding C (2015) Food Chem 180:156
Crevillen AG, Avila M, Pumera M, Gonzalez MC, Escarpa A (2007) Anal Chem 79:7408
Sýs M, Khaled E, Metelka R, Vytřas K (2017) J Serb Chem Soc 82:865
Švancara I, Vytřas K, Barek J, Zima J (2001) Crit Rev Anal Chem 31:311
Zima J, Švancara I, Barek J, Vytřas K (2009) Crit Rev Anal Chem 39:204
Rizk M, Attia AK, Elshahed MS, Farag AS (2015) J Electroanal Chem 743:112
Digua K, Kauffmann JM, Delplancke JL (1994) Electroanal 6:451
Lee JHQ, Lauw SJL, Webster RD (2016) Electrochim Acta 211:533
Amarasekara MS, Wiredu B, Razzaq A (2012) Green Chem 14:2395
Uddin W, Hu G, Hu L, Shen X, Fang Z, Zhang Y, Song J (2017) Int J Electrochem Sci 12:178
Chethana BK, Basavanna S, Naik YA (2012) J Chem Pharm Res 4:538
Yardim Y, Gulcan M, Şentürk Z (2013) Food Chem 141:1821
Jiang L, Ding Y, Jiang F, Li L, Mo F (2014) Anal Chim Acta 833:22
Xinying MA (2014) Int J Electrochem Sci 9:3181
Li J, Feng H, Li J, Jiang J, Feng Y, He L, Qian D (2015) Electrochim Acta 176:827
Liu Y, Liang Y, Lian H, Zhang C, Peng J (2015) Int J Electrochem Sci 10:4129
Silva TR, Brondani D, Zapp E, Vieira IC (2015) Electroanalysis 27:465
Vilian ATE, Puthiaraj P, Kwak CH, Hwang S, Huh YS, Ahn W, Han Y (2016) Appl Mater Interfaces 8:12740
Ali HS, Abdullah AA, Pınar PT, Yardım Y, Şentürk Z (2017) Talanta 170:384
Filik H, Avan AA, Mümin Y (2017) Food Anal Methods 10:31
Kalaiyarasi J, Meenakshi S, Pandian K, Gopinath SCB (2017) Microchim Acta 184:2131
Khalilzadeh MA, Arab Z (2017) Curr Anal Chem 13:81
Wu W, Yang L, Zhao F, Zeng B (2017) Sens Actuators B Chem 239:481
Alpar N, Yardım Y, Şentürk Z (2018) Sens Actuators B Chem 257:398
Durán GM, Llorent-Martínez EJ, Contento AM, Ríos A (2018) Microchim Acta 185:204
Cheraghi S, Taher MA, Karimi-Maleh H (2018) J Food Compost Anal 62:254
Sýs M, Žabčíková S, Červenka L, Vytřas K (2017) Potravinarstvo Slovak J Food Sci 11:96
Acknowledgements
The support received from the Faculty of Chemical Technology, University of Pardubice (project no. SGS-2018-001) is gratefully acknowledged.
Author information
Authors and Affiliations
Corresponding author
Rights and permissions
About this article
Cite this article
Farag, A.S., Sýs, M., Hájek, T. et al. Voltammetric determination of ethylvanillin and methylvanillin sum at carbon paste electrode modified by sodium dodecyl sulfate in selected foodstuffs. Monatsh Chem 149, 1945–1953 (2018). https://doi.org/10.1007/s00706-018-2266-z
Received:
Accepted:
Published:
Issue Date:
DOI: https://doi.org/10.1007/s00706-018-2266-z