Abstract
Leaf spot of perennial ryegrass (Lolium perenne) caused by Bipolaris sorokiniana is an important disease in temperate regions of the world. We designed this experiment to test for the combined effects of the arbuscular mycorrhizal (AM) fungus Claroideoglomus etunicatum and the grass endophyte fungus Epichloë festucae var. lolii on growth and disease occurrence in perennial ryegrass. The results show that C. etunicatum increased plant P uptake and total dry weight and that this beneficial effect was slightly enhanced when in association with the grass endophyte. The presence in plants of both the endophyte and B. sorokiniana decreased AM fungal colonization. Plants inoculated with B. sorokiniana showed the typical leaf spot symptoms 2 weeks after inoculation and the lowest disease incidence was with plants that were host to both C. etunicatum and E. festucae var. lolii. Plants with these two fungi had much higher activity of peroxidases (POD), superoxide dismutase (SOD) and catalase (CAT) and lower values of malondialdehyde (MDA) and hydrogen peroxide (H2O2). The AM fungus C. etunicatum and the grass endophyte fungus E. festucae var. lolii have the potential to promote perennial ryegrass growth and resistance to B. sorokiniana leaf spot.
Similar content being viewed by others
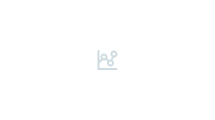
Avoid common mistakes on your manuscript.
Introduction
Field-growing plants are exposed to a wide range of microorganisms both within and above the soil. The roots of many plants, including grasses, are extensively colonized by arbuscular mycorrhizal (AM) fungi of the sub-phylum Glomeromycotina (Spatafora et al. 2016). The presence of these fungi in roots typically enhances the growth of host plants, particularly in soils with low concentrations of available phosphorus. Other reported benefits to host plants include improved plant nutrient uptake (Marschner and Dell 1994) enhanced plant resistance to biotic stresses including insect pests (Pineda et al. 2010), disease (Whipps 2004; Saldajeno and Hyakumachi 2011; Elsharkawy et al. 2012; Lee et al. 2012; Maya and Matsubara 2013; Lenoir et al. 2017), and possibly abiotic environmental stresses (Zaefarian et al. 2013).
With many grasses of the sub-family Pooideae, a very specific type of mutualistic association is made with fungi of the genus Epichloë. These fungi are exclusively seed-borne, mostly by vertical transmission (Zhang et al. 2017), but some may infect developing seed following horizontal transmission (Chung and Schardl 1997). These fungi are in all tissues apart from the roots and are located in the intercellular spaces. The nature of the association of these systemic fungal endophytes can be thought of as if these fungi were a plant tissue as their growth in vegetative tissues is fully synchronized with that of the host grass (Christensen et al. 2008). A key function of the in situ hyphae is to produce secondary metabolites, particularly alkaloids, which protect the host from non-vertebrate pests. Some of the alkaloids also adversely affect the growth of grazing livestock (Gallagher et al. 1984; Strickland et al. 1996; Popay and Hume 2011). These effects on non-vertebrate pests and grazing livestock can enhance the persistence of host plants.
In addition to the effects that the presence of an Epichloë sp. endophyte has on insects and grazing livestock, there are reports that there are effects against some pathogens (Ma et al. 2015), although as yet there are few reports of disease resistance associated with field-growing endophyte-infected grasses. There is strong field-based evidence that endophyte-infected tall fescue plants have enhanced tolerance to drought stress (West et al. 1993) and there are greenhouse-based studies of enhanced tolerance to harmful insects (Barker 1987; Vicari et al. 2002), toxicity from heavy metals, high salinity, and onset of cold conditions (Parsaeian et al. 2006; Baltruschat et al. 2008; Zhang et al. 2009). The best studied associations between Epichloë species and grasses are those with perennial ryegrass (Lolium perenne) and tall fescue (Festuca arundinacea), two species with widespread use in pasture-based agriculture (Johnson et al. 2013), and drunken horse grass (Achnatherum inebrians) (Li et al. 2004).
Perennial ryegrass is native to temperate regions of Europe but is now naturalized and widely used as pasture in temperate regions of North and South America, Japan, and China as well as in New Zealand where it is the most important grass for pastures. It is interesting that in New Zealand, there has been very strong selection pressure for endophyte-infected perennial ryegrass, especially for plants containing strains that produce three alkaloids that provide protection against herbivores: peramine, ergovaline, and lolitrem B. (Tapper and Latch 1999). Perennial ryegrass is increasing in use in parts of China (Wang and Zhao 2014). Fungal diseases are a problem with perennial ryegrass in China as in other parts of the world. Rust is one of the serious problems and another is a leaf spot disease caused by Bipolaris sorokiniana. Field trials with endophyte-infected (E+) and endophyte-free (E−) perennial ryegrass plants of the cultivar Fairway found that the presence of the Epichloë festucae var. lolii enhanced resistance against rust disease caused by Puccinia coronata (Ma and Nan 2011). There are no reports regarding the effects of the presence of this endophyte on the susceptibility of plants of this perennial ryegrass cultivar on leaf disease caused by B. sorokiniana. In another recent study involving this cultivar, the effects of the presence or absence of E. festucae var. lolii on four indicators of oxidative stress were measured in germinating seedlings exposed to five fungal pathogens, including B. sorokiniana (Ma et al. 2015). These indicators were the concentration of malondialdehyde (MDA) and proline and activity of peroxidases (POD) and superoxide dismutase (SOD). This study indicated that the presence of the fungal endophyte reduced oxidative stress of seedlings exposed to the fungal pathogens.
Roots of perennial ryegrass plants growing under field conditions are colonized by AM fungi (Zhu et al. 2000). Thus, plants which are hosts to mutualistic mycorrhiza in the roots also may be hosts to a mutualistic Epichloë sp. endophyte in the aboveground tissues. Several studies have looked at the interaction between endophytes and AM fungi in perennial ryegrass and the closely related grasses annual ryegrass (Lolium multiflorum) and tall fescue (F. arundinacea). Two studies using perennial ryegrass that looked at the effect of the presence of an epichloid endophyte on AM colonization reported that the foliage-confined endophyte reduced AM root colonization (Müller 2003; Liu et al. 2011). Similarly, the presence of Epichloë coenophiala (= Neptyphodium coenophialum) in tall fescue (Chu-Chao et al. 1992; Antunes et al. 2008) and of Epichloë occultans (= Neptyphodium occultans) in annual ryegrass (Omacini et al. 2006) reduced AM colonization. A further study showed that the presence of AM fungi in roots of perennial ryegrass with E. festucae var. lolii reduced the feeding deterrence effect against the insect pest Argentine stem weevil (Listronotis bonariensis) (Barker 1987). Another study, however, found that the presence of AM fungi in endophyte-infected perennial ryegrass reduced the survival of the angle shades moth (Phlogophora meticulosa) (Vicari et al. 2002). Based on these reports and studies in other endophyte-infected grasses (Novas et al. 2011; Larimer and Clay 2012), we undertook a study to understand the role and physiological mechanisms that each of the partners of the complex association with perennial ryegrass has on plant growth and infection by the fungal pathogen B. sorokiniana. The questions that we wanted to examine were would the presence of E. festucae var. lolii, an AM fungus species, Claroideoglomus etunicatum, and the combination of the two fungi, have beneficial effects on plant growth, and would the presence of E. festucae var. lolii and the AM fungus, either individually or in combination, provide resistance against B. sorokiniana? Our study utilized all combinations of the three types of fungi. We hypothesized that (1) the presence of C. etunicatum or E. festucae var. lolii would have beneficial effects on plant growth and increase resistance to the pathogen and (2) the presence of the AM fungus and the grass endophyte would reduce oxidative stress in perennial ryegrass such that the resistance of perennial ryegrass to B. sorokiniana will increase when plants are host to both C. etunicatum and E. festucae var. lolii versus host to each individually.
Materials and methods
Experimental design
Plants and fungi used in the study
Perennial ryegrass, with and without the systemic endophyte E. festucae var. lolii, provided by the Forage and Turfgrass Quality Supervision Testing Center, Ministry of Agriculture, China, was the grass species used in this study. The cultivar used was ‘Fairway,’ the same perennial ryegrass cultivar that had been used in a study that looked at the effect of the presence of this seed-borne fungal endophyte on indicators of oxidative stress during invasion by fungal pathogens, including B. sorokiniana (Ma et al. 2015). The E+ and E− seeds used in this current study were obtained from plants that had been confirmed as being either E+ or E− by examination of leaf sheaths stained with aniline blue for the presence or absence of hyphae characteristic of E. festucae var. lolii. These hyphae were intercellular, seldom-branched, orientated parallel to the longitudinal leaf axis, 2–3 μm in diameter, and with non-staining septa.
The AM fungus used in the study was C. etunicatum. The AM fungus species was obtained from the Institute of Plant and Environmental Resource of Chinese Academy of Agriculture. Prior to 2010, the species was classified as Glomus etunicatum. The fungus had been maintained in dried-out pots of white clover (Trifolium repens) that were stored at room temperature. The pots had been allowed to dry when the number of spores of the AM fungus had reached the concentration of ~ 100 g−1 dry soil. We prepared inoculum of the AM fungus from the dry soil and from roots of the source white clover plants. The white clover plants were removed from the soil and the roots cut into pieces approximately 0.5 cm in length.
The isolate of B. sorokiniana used in the study was isolated from locally growing perennial ryegrass plants. Leaves with symptoms characteristic of B. sorokiniana were surface-sterilized by rinsing in 75% alcohol, then in 0.3% NaClO for 1–2 min and then washed several times with distilled water and dried on sterilized filter paper. The leaf tissue was then cut into small pieces (~ 0.2 cm in length), placed on potato dextrose agar (PDA) containing penicillin and streptomycin, each at a concentration of 0.5 mL L−1 and incubated at 25 °C. The isolates were observed every day for the presence of conidia, allowing identification of the pathogen. The B. sorokiniana isolated was sub-cultured on PDA and kept for 7–10 days at 25 °C (Nan 1995). Conidia were obtained by adding sterile water to Petri dishes containing conidia-producing B. sorokiniana colonies, scraping the surface with a sterilized spreader, filtering the suspensions through four layers of sterilized cheese cloth, and using a hemocytometer to obtain a concentration of approx. 1 × 106 conidia mL−1.
Potting medium
We collected soil from the Xinglong Mountain, Lanzhou, China, to prepare the potting mix used for our experiment. The mix consisted of 50% of soil and 50% sand. Both components were dried at room temperature, and then sieved through a 2-mm sieve. The soil and sand mixture was twice sterilized via autoclaving at 121 °C for 1 h, over a period of 3 days, and then dried in an oven at 110 °C for 36 h. The mix had 19.17 mg P kg−1 plant-available P (resin extraction method) (McLaughlin et al. 1994) and a pH of 5.85.
Greenhouse study
The trial was conducted in a greenhouse and involved either E+ or E− perennial ryegrass plants growing in pots (20-cm high and 15-cm wide), containing 1500g of the potting mix. There were 16 pots of E+ and 16 pots of E− perennial ryegrass plants. Eight pots of E+ and eight pots of E− plants were inoculated with C. etunicatum, the same number of E+ and E− pots did not receive this AM fungus. Four of the eight pots of each treatment were inoculated with B. sorokiniana. There were four replicates per treatment, resulting in a total of 32 pots ryegrass.
We surface-sterilized the E+ and the E− perennial ryegrass seeds with 3% sodium hypochlorite (NaClO) for 30 s following which they were rinsed three times with sterile water. We placed the sterilized seeds on wet filter paper in the dark at 25 °C and following the onset of germination, six germinating E+ or E− seeds were transplanted into each pot containing the potting mix. Prior to the transplanting of seedlings, 20 g of the dry soil and white clover root fragments inoculum of AM fungus were added to 16 pots to provide the AM treatment. For the non-inoculated control plants (NM) treatments, 20 mL of the solutions that contains microbes excluding AM fungus were added to the soil with a previously prepared microbial filtrate (50 μm).
Each seedling was checked for the presence or absence of hyphae characteristic of E. festucae var. lolii after 2 weeks of growth. Small epidermal strips were peeled off the adaxial surface of the leaf sheaths and placed on a glass slide in 1 to 2 drops of aniline blue-lactic acid-glycerol solution, covered with a glass cover-slip, heated gently to boiling to drive out air bubbles and examined at ×100–400 magnification for the presence or absence of hyphae characteristic of epichloid endophytes (Florea et al. 2015). Each pot was thinned to three plants and maintained in a greenhouse for another 6 weeks. During those 6 weeks, the numbers of tillers and the height of each plant were recorded at weekly intervals.
Six weeks after seedling emergence, four of the pots of each of the AM fungus treatments involving either E+ or E− perennial ryegrass plants, a total of 16 pots were inoculated with B. sorokiniana. Ten milliliters of the suspension of B. sorokiniana conidia described above was sprayed onto each plant. The same amount of sterilized water was sprayed onto our control (non-inoculated) treatments. Immediately after being sprayed with either the suspension of conidia or sterilized water, each pot was covered with a black plastic bag for the following 2 days.
We conducted the experiment from April to July in a glasshouse with irradiance in the range of 180–850 mmol m−2 s−1. The average temperatures were 23–28 °C (day) and 20–25 °C (night). We applied a modified Long Ashton nutrient solution (−P) (Duan et al. 2011) to the pots every other day during the experiment.
Plant harvest and measurement
Two weeks after inoculation with B. sorokiniana, the plants were visually assessed for the presence of lesions. The incidence of lesions was noted on 25 leaves of each of four pots per treatment. Disease incidence was calculated by the number of leaves with lesions, divided 25 for each pot. The plants were then harvested by cutting the tillers at ground level. Tillers from the three plants of each pot were combined. Three subsamples of the combined tillers of each pot were taken and each was weighed. One of the samples was used to do all the analyses, another to do isolations from lesions, while the third was oven-dried to obtain the dry weight, and this was used to calculate the total aboveground dry weight of the plants of each pot. The pathogen was re-isolated with Nan’s methods (1995) as previously described. The fungi were microscopically identified based on morphological characteristics of the asexual reproductive structures and colony characters. Phosphorous and soluble protein concentrations, the activity of superoxide dismutase (SOD), peroxidase (POD), catalase (CAT), and polyphenol oxidase (PPO), enzymes associated with the response of plants to pathogen invasion, along with malondialdehyde (MDA) and hydrogen peroxide (H2O2), were determined. The oven-dried plant material was finely ground and weighed. Phosphate concentration of plant tissue was determined colorimetrically with a UV-Visible spectrophotometer (Shimadzu, Australia) at 390 nm, using the phosphovanado-molybdate method (Hanson 1950) after digestion of the dried, ground plant material (~ 0.5 g) in 5 mL of concentrated nitric acid. The activity of three of the enzymes, MDA, and hydrogen peroxide concentration were determined with an ultraviolet spectrophotometer (Pectrum, Shanghai 721 China). We determined the CAT activity with another UV spectrophotometer (PerkinElmer-Lambda 25-USA) at 240 nm by the published method of Beers and Sizer (1952). We determined the activity of SOD and POD and soluble protein content at 560, 470, and 595 nm, respectively (Li et al. 2000). We determined PPO activity at 495 nm via the method of Kumar and Khan (1982). Hydrogen peroxide content was determined at 390 nm (Velikova et al. 2000) and we determined MDA at 600, 450, and 532 nm using the method of Li et al. (2000).
We washed the roots in each pot and made three subsamples, two of approximately 0.1 g while the third was the remainder. One subsample was used to assess AM fungal colonization, the second to examine if B. sorokiniana had colonized some segments of roots using the method of Nan (1995) while the bulk of each root sample was used to determine total root dry weight. AM fungal colonization of roots was determined using the gridline intersect method of Giovannetti and Mosse (1980). After clearing in 10% KOH and staining in 1% trypan blue (Phillips and Hayman 1970), ~ 0.1 g 1-cm root segments were selected at random and observed using a compound microscope at ×400 magnification. The presence of vesicles, arbuscules, and intraradical hyphae was recorded in 25 fields of view. The possible presence of B. sorokiniana in roots was assessed by placing small pieces of surface-sterilized roots onto antibiotic PDA as done for pieces of leaves with symptoms.
Statistical analysis
All data are presented as means and standard errors of the mean of four replicates. Homogeneity of variances was tested with Levene’s test and normality of distributions with the Kolmogorov-Smirnov test. The data for percent AM colonization were ARCSIN-transformed to achieve normality. We analyzed the data using the three-factor ANOVA analysis of variance, using the statistical analysis software SPSS 19.0 (SPSS Inc., Chicago, USA). Tukey’s HSD all-pairwise comparisons were used to compare treatment means. Statistical significance was determined at P ≤ 0.05.
Results
AM fungal colonization and disease incidence
All of the perennial ryegrass plants that were examined in the E+ treatments were found to be endophyte-infected whereas none of the perennial ryegrass plants examined in the E− treatments were infected. Examination of a representative sample of roots in the AM treatment revealed the presence of structures characteristic of this association, namely vesicles, arbuscules, and non-septate hyphae. In contrast, these structures were not observed in roots of plants in the non-inoculated treatments. E+ plants had 24% less C. etunicatum root colonization on average than E− plants, and B. sorokiniana decreased C. etunicatum colonization by 17%; plants with endophyte and the pathogen had the lowest C. etunicatum colonization (Fig. 1a).
AM colonization (a) and disease incidence (b) of perennial ryegrass with (E+) and without (E−) grass endophyte and colonized by Claroideoglomus etunicatum (stippled bars) or non-mycorrhizal (open bars) at harvest. Mean ± SEM of four replicates. B = Bipolaris sorokiniana applied, NB = no B. sorokiniana. Bars topped by the same lowercase letter do not differ significantly between treatments at P ≤ 0.05 by Tukey’s HSD. See Table 1 for ANOVA results
Plants inoculated with B. sorokiniana showed typical leaf spot symptoms 2 weeks after inoculation. No leaf symptoms were present on non-inoculated plants. We re-isolated the pathogen B. sorokiniana from lesions of inoculated plants. Compared with the NM E− treatment, C. etunicatum alone or with the endophyte significantly decreased B. sorokiniana leaf spot incidence (Fig. 1b). Plants with C. etunicatum had 23% less disease incidence on average than NM plants (Fig. 1b).
Plant growth
Perennial ryegrass responded positively to the combination of E. festucae var. lolii and C. etunicatum colonization. Effects first became apparent 14 days after being transplanted into the pots with the potting mix containing, or not containing, C. etunicatum, when all seedlings with both E. festucae var. lolii and AM fungus had visibly increased height compared to E− plants with and without C. etunicatum (Supplementary Fig. S1). The presence of C. etunicatum provided a significant increase in total dry weight (DW) only with E+ plants, whereas the total dry weight of E− plants was only slightly increased. The presence of E. festucae var. lolii and the pathogen had no significant effect on the total DW of plants (Fig. 2a). Root/shoot (R/S) ratios were not different between treatments (Table 1; Supplementary Fig. S2). The effects of E. festucae var. lolii and C. etunicatum colonization on shoot DW, root DW, and root length are very similar to total DW across all treatments (Supplementary Fig. S3). We found no interaction effects between C. etunicatum, E. festucae var. lolii, and the pathogen (see Table 1 for ANOVA results on these parameters).
Total dry weights (roots plus shoots) (a), shoot P concentration (b), and Total P content (roots plus shoots) (c) of perennial ryegrass with (E+) and without (E−) grass endophyte and colonized by Claroideoglomus etunicatum (stippled bars) or non-mycorrhizal (open bars) at harvest. Mean ± SEM of four replicates. B = Bipolaris sorokiniana applied, NB = no B. sorokiniana. Bars topped by the same lowercase letter do not differ significantly between treatments at P ≤ 0.05 by Tukey’s HSD. See Table 1 for ANOVA results
Phosphorous nutrition
We found significant effects of E. festucae var. lolii, AM fungal colonization, and B. sorokiniana infection on phosphorous (P) concentration in shoots (Fig. 2b) and roots (Supplementary Fig. S4). Furthermore, the grass endophyte and the inoculation with C. etunicatum separately had interaction effects on foliage P concentration (Table 1). Values for pathogen-infected plants were significantly decreased compared with those of uninfected plants except for NM E−. The presence of both C. etunicatum and E. festucae var. lolii significantly increased foliage P concentration (Fig. 2b).
Total P content followed a trend similar to total DW, and was significantly affected by the presence of each of the three fungi (Table 1). E+ plants had the highest total P content in combination with C. etunicatum (Fig. 2c). The inoculation of B. sorokiniana decreased plant P uptake in all treatments except for NM E− plants (Fig. 2c).
Enzyme activity
The activity of enzymes was significantly affected by the presence of the grass endophyte and inoculation with C. etunicatum throughout all treatments except for CAT in plants with C. etunicatum (Fig. 3; Table 1). The co-colonization with E. festucae var. lolii and C. etunicatum gave much higher values of POD activity compared to NM E− and of each of them alone (Fig. 3). The SOD and CAT values largely followed the same pattern as for POD, with the values in AM E+ plants being higher than for other plants. Similarly, the values of SOD in plants inoculated with B. sorokiniana were lower than for paired un-inoculated plants in the NM E− and AM E+ treatments. In general, the levels of PPO were similar in all treatments with the exception being the extremely high relative level in the E+ plants that were host to C. etunicatum without inoculation of B. sorokiniana (Supplementary Fig. S5).
Peroxidases (POD) enzyme activity of perennial ryegrass with (E+) and without (E−) grass endophyte and colonized by Claroideoglomus etunicatum (stippled bars) or non-mycorrhizal (open bars) at harvest. Mean ± SEM of four replicates. B = Bipolaris sorokiniana applied, NB = no B. sorokiniana. Bars topped by the same lowercase letter do not differ significantly between treatments at P ≤ 0.05 by Tukey’s HSD. See Table 1 for ANOVA results
MDA, H2O2, and soluble protein concentrations
The presence of E. festucae var. lolii and infection with B. sorokiniana, had significant effects on MDA and H2O2 concentration. Colonization with C. etunicatum, however, had no significant effects on these parameters (Fig. 4a, b; Table 1). NM E− and AM E+ without infection of B. sorokiniana gave the highest and lowest value of MDA and H2O2 concentration, respectively (Fig. 4a, b). E+ plants had 18 and 33% less MDA and H2O2 concentration on average than E− plants, respectively, and B. sorokiniana increased the MDA and H2O2 concentration by 24 and 26%, respectively (Fig. 4a, b). Soluble protein concentration is similar across the treatments except co-colonization with E. festucae var. lolii and C. etunicatum that had the highest values although this was only affected significantly by E. festucae var. lolii (Fig. 4c; Table 1). .
Malondialdehyde (MDA) concentration (a), hydrogen peroxide (H2O2) concentration (b), and soluble protein concentration (c) of perennial ryegrass with (E+) and without (E−) grass endophyte and colonized by Claroideoglomus etunicatum (stippled bars) or non-mycorrhizal (open bars) at harvest. Mean ± SEM of four replicates. B = Bipolaris sorokiniana applied, NB = no B. sorokiniana. Bars topped by the same lowercase letter do not differ significantly between treatments at P ≤ 0.05 by Tukey’s HSD. See Table 1 for ANOVA results
Discussion
Using the AM fungus C. etunicatum, the pathogen B. sorokiniana, and perennial ryegrass with and without E. festucae var. lolii in a glasshouse experiment, we revealed that perennial ryegrass plants that are host to both the foliage-confined systemic endophyte and root-confined AM fungus had improved growth, even in the presence of the foliage pathogen B. sorokiniana. The improved growth resulting from the presence of the epichloid endophyte and C. etunicatum occurred even though perennial ryegrass plants that were host to E. festucae var. lolii had lower colonization of this AM fungus than plants that did not host the endophyte. Our hypothesis that the presence of C. etunicatum or E. festucae var. lolii will increase plant growth and resistance to the pathogen was supported. The presence of C. etunicatum in the roots of endophyte-infected plants gave the best growth and best reduction in the adverse effects of the foliage pathogen, which coincided with the best uptake of P. E. festucae var. lolii alone did not affect perennial ryegrass leaf spot incidence. Our hypothesis that the resistance of perennial ryegrass to B. sorokiniana will increase when plants are host to both C. etunicatum and E. festucae var. lolii, compared to each individually was upheld. Our results reveal that plants infected with B. sorokiniana had higher values of H2O2 for most treatments, and this was especially prominent in plants host to E. festucae var. lolii. The effects on levels of MDA are similar to those on H2O2. The activity of the disease-response related enzymes, SOD, POD, CAT, and PPO, are mostly higher in treatments involving both E. festucae var. lolii and C. etunicatum than for other treatments.
As previously stated, a number of studies have examined the effects of the presence of an epichloid endophyte and AM fungi in host grasses. A key measureable outcome of several studies was that the presence of the foliage-confined epichloid endophyte resulted in a lower rate of root colonization by AM fungi (Chu-Chao et al. 1992; Müller 2003; Omacini et al. 2006; Attunes et al. 2008; Liu et al. 2011). Conversely, the finding of Novas et al. (2005), who studied two populations of Bromus setifolius, one with and one without endophyte association, that were grown in either the presence or the absence of AM fungi using two different sources of field soil, found that the roots of the E+ population were more extensively colonized than those of the E− populations. The differences that have been found in the different studies may be caused by differences in host genotype, Epichloë species and strain, and AM fungi species, as well as soil factors, in particular, available P content. Plants typically have higher AM fungal colonization under low P compared to high P conditions (Facelli et al. 2014). The high soil available P content of 19.18 mg kg−1 in our experiment might have affected the interactions between AM fungal colonization and grass endophyte. Moreover, our study revealed that AM fungal colonization in perennial ryegrass was decreased due to infection with B. sorokiniana, where there was the lowest AM fungal colonization when the E+ population was infected by B. sorokiniana. This finding agrees with Zambolim and Schenck (1983), who reported an average decrease of AM fungal colonization of 38% in soybean roots due to the infection of roots by Macrophomina phaseolina, Rhizoctonia solani, and Fusarium solani. This may be because of competition for colonization sites and for photosynthates from the host plants between AM fungi and the pathogens. Furthermore, Maya and Matsubara (2013) reported the relationship between AM fungi and disease severity to show a negative correlation and that competition or antagonistic effects occurred between the AM fungus Glomus fasciculatum and root pathogens Fusarium oxysporum and Colletotrichum gloeosporioides. To our knowledge, however, our study is the first that has examined how the presence of an epichloid endophyte and AM fungus affects the incidence of a foliage fungal pathogen and the growth of the host plant.
The strength of our study on the effect of two mutualist fungal symbionts and the foliage pathogen on the host grass is that all combinations of partners, including the absence of all three fungal species, were assessed. Thus, our study provides an indication of what may be happening under field conditions where perennial ryegrass plants inevitably will have root systems colonized by AM fungi. Our study contrasts with many greenhouse studies that have examined the effects of the presence of Epichloë spp. endophytes of grasses on the susceptibility of host grasses to pathogenic fungi, where the grasses were growing in heat-treated soil or potting mix (Gu et al. 2007; Tian et al. 2008; Ma and Nan 2011; Sabzalian et al. 2012; Wang et al. 2014). Plants in those trials would have been unlikely to have a functional AM fungal root flora. Based on the finding of our study that the presence of both the systemic foliage endophyte and the root-confined C. etunicatum provided maximum enhancement of plant growth, even in the presence of a foliage pathogen, it is likely that previous studies have underestimated the role that both types of fungal mutualists are having on fungal diseases under field conditions.
Our results show that the AM fungus has overlying effects in improving plant disease resistance and which may be associated with biocontrol capacities (Lopez-Raez et al. 2010). This finding agrees with Martinez-Medina et al. (2009), who studied the effect of the interaction between different species belonging to the genus Glomus sp. and the saprotrophic fungus Trichoderma harzianum in growth promotion of melon plants and in a biocontrol effect against Fusarium wilt.
It is well established that AM fungal colonization is able to enhance plant resistance and growth by increasing P uptake (Marschner and Dell 1994; Smith and Read 1996). In our experiments, plants hosting C. etunicatum had increased P uptake. The positive growth responses and the reduced effects of B. sorokiniana on plants hosting C. etunicatum are consistent with increased P uptake. There are also research that has found that AM fungi do not have a positive effect or even have negative effect by plants (Klironomos 2003; Li et al. 2008) This difference between studies shows that the effects of AM fungi on plant P uptake depend on host genotype-AM fungi combinations and environmental conditions (Gavito et al. 2002; Schroeder and Janos 2005).
Another aspect of our study was its focus on the levels of H2O2 and MDA and of enzymes associated with reducing oxidative stress resulting from attack by pathogens. The expected results would have shown that plants infected with B. sorokiniana had elevated levels of H2O2 and MDA compared with non-inoculated plants. Increased levels would provide an indication of the level of oxidative stress experienced by plants infected with B. sorokiniana. Initially, the increase in levels of H2O2 in plants will inhibit the infection by plant pathogens, but as the concentrations increase further, the effects on plants are negative resulting from damage to proteins and lipids in plant cells due to peroxidation(Bolwell and Daudi 2009).
To alleviate the damaging effects of H2O2, plants will utilize antioxidant enzymes such as POD, SOD, CAT, and PPO to protect their cells from severe damage (Zheng et al. 2010). When plants are under stress such as from pathogens, the host will start removing active oxygen by producing antioxidant protection enzymes to prevent further damage of the pathogen. In our study, however, in all treatments, including the no-AM fungus controls, the activity of SOD, POD, and CAT was greater in the non-inoculated plants than in plants inoculated with B. sorokiniana, which was contrary to our expectation. This is difficult to explain because the presence of the pathogen would be expected to increase stress and so the activities of these enzymes should increase to help reduce the levels of induced reactive oxygen species. The lower activity of SOD, POD, and CAT in plants inoculated with B. sorokiniana, however, is in agreement with a study of cyclamen plants, which reported that infection with F. oxysporum and C. gloeosporioides decreased antioxidative activity of the infected plants (Maya and Matsubara 2013). The findings are also in agreement with research that shows that SOD activity decreased when rice leaves were inoculation with R. solani, and the activity decrease was most obvious in the fourth day (Zhang et al. 2006). That consequence may be related to the increased content of cellulose and lignin when plants suffered stress (Wu et al. 2008). Not unexpectedly, in nearly all of our treatments, the levels of these enzymes were higher in the E+ than E− plants and this is in agreement with the finding of the study of exposure of seedlings of the same perennial ryegrass cultivar to B. sorokiniana and four other pathogens (Ma et al. 2015).
References
Antunes PM, Miller J, Carvalho LM, Klironomos JN, Newman JA (2008) Even after death the endophytic fungus of Schedonorus phoenix reduces the arbuscular mycorrhizas of other plants. Funct Ecol 22(5):912–918. https://doi.org/10.1111/j.1365-2435.2008.01432.x
Baltruschat H, Fodor J, Harrach BD, Niemczyk E, Barna B, Gullner G, Janeczko A, Kogel KH, Schäfer P, Schwarczinger I, Zuccaro A, Skoczowski A (2008) Salt tolerance of barley induced by the root endophyte Piriformospora indica is associated with a strong increase in antioxidants. New Phytol 180(2):501–510. https://doi.org/10.1111/j.1469-8137.2008.02583.x
Barker GM (1987) Mycorrhizal infection influences Acremonium-induced resistance to argentine stem weevil inryegrass. In: New Zealand weed and pest control Conference, Wellington, pp 199–203
Beers RF, Sizer IW (1952) A spectrophotometric method for measuring the breakdown of hydrogen peroxide by catalase. J Biol Chem 195(1):133–140
Bolwell GP, Daudi A (2009) Reactive oxygen species in plant pathogen interactions. In: Reactive oxygen species in plant signaling. Springer Berlin, Heidelberg, pp 113–133. https://doi.org/10.1007/978-3-642-00390-5_7
Christensen MJ, Bennett RJ, Ansari HA, Koga H, Johnson RD, Bryan GT, Simpson WR, Koolaard JP, Nickless EM, Voisey CR (2008) Epichloë endophytes grow by intercalary hyphal extension in elongating grass leaves. Fungal genet bio l45:84-93
Chu-Chao M, Guo B, An ZQ, Hendrix JW, Ferriss RS, Siegel MR, Dougherty CT, Burrus PB (1992) Suppression of mycorrhizal fungi in fescue by the Acremonium coenophialum endophyte. Soil Biol Biochem 24(7):633–637. https://doi.org/10.1016/0038-0717(92)90041-U
Chung KR, Schardl LC (1997) Sexual cycle and horizontal transmission of the grass symbiont, Epichloë typhina. Mycol Res 101(3):295–301. https://doi.org/10.1017/S0953756296002602
Duan TY, Facelli E, Smith SE, Smith FA, Nan ZB (2011) Differential effects of soil disturbance and plant residue retention on function of arbuscular mycorrhizal (AM) symbiosis are not reflected in colonization of roots or hyphal development in soil. Soil Biol Biochem 43(3):571–578. https://doi.org/10.1016/j.soilbio.2010.11.024
Elsharkawy MM, Shimizu M, Takahashi H, Hyakumachi M (2012) The plant growth-promoting fungus Fusarium equiseti, and the arbuscular mycorrhizal fungus Glomus mosseae, induce systemic resistance against cucumber mosaic virus in cucumber plants. Plant Soil 361(1-2):397–409. https://doi.org/10.1007/s11104-012-1255-y
Facelli E, Duan T, Smith SE, Christophersen HM, Facelli JM, Smith FA (2014) Opening the black box: outcomes of interactions between arbuscular mycorrhizal (AM) and non-host genotypes of Medicago depend on fungal identity, interplay between P uptake pathways and external P supply. Plant Cell Environ 37(6):1382–1392. https://doi.org/10.1111/pce.12237
Florea S, Schardl CL, Hollin W (2015) Detection and isolation of Epichloë species, fungal endophytes of grasses. Curr Protoc Microbiol 38:19A.1.1–19A.1.24
Gallagher RT, Hawkes AD, Steyn PS, Vleggaar R (1984) Tremorgenic neurotoxins from perennial ryegrass causing ryegrass staggers disorder of livestock: structure elucidation of lolitrem B. J Chem Soc Chem Commun 9:614–616
Gavito ME, Bruhn D, Jakobsen I (2002) Phosphorus uptake by arbuscular mycorrhizal hyphae does not increase when the host plant grows under atmospheric CO2 enrichment. New Phytol 154(3):751–760. https://doi.org/10.1046/j.1469-8137.2002.00404.x
Giovannetti M, Mosse B (1980) An evaluation of techniques for measuring vesicular arbuscular mycorrhizal infection in roots. New Phytol 84(3):489–500. https://doi.org/10.1111/j.1469-8137.1980.tb04556.x
Gu YX, Wang DJ, Hu YG (2007) The effect of endophytic fungus on curvularia lunate in Festuca arundinacea. Chin J Grassl 29:112–115
Hanson WC (1950) The photometric determination of phosphorus in fertilizers using the phosphovanado-molybdate complex. J Sci Food Agric 1(6):172–173. https://doi.org/10.1002/jsfa.2740010604
Johnson LJ, Bonth ACMD, Briggs LR, Caradus JR, Finch SC, Fleetwood DJ, Fletcher LR, Hume DE, Johnson RD, Popay AJ, Tapper BA, Simpson WR, Voisey CR, Card SD (2013) The exploitation of epichloae endophytes for agricultural benefit. Fungal Divers 60(1):171–188. https://doi.org/10.1007/s13225-013-0239-4
Klironomos JN (2003) Variation in plant response to native and exotic arbuscular mycorrhizal fungi. Ecol 84(9):2292–2301. https://doi.org/10.1890/02-0413
Kumar KB, Khan P (1982) Peroxidase and polyphenol oxidase in excised ragi (Eleusine corocana) leaves during senescence. Indian J Expe Biol 20:412–416
Larimer AL, Clay K (2012) Consequences of simultaneous interactions of fungal endophytes and arbuscular mycorrhizal fungi with a shared host grass. Oikos 121(12):2090–2096. https://doi.org/10.1111/j.1600-0706.2012.20153.x
Lee BR, Muneer S, Jung WJ, Aviced JC, Ourryd A, Kim TH (2012) Mycorrhizal colonization alleviates drought- induced oxidative damage and lignification in the leaves of drought-stressed perennial ryegrass (Lolium perenne). Physiol Plant 145(3):440–449. https://doi.org/10.1111/j.1399-3054.2012.01586.x
Lenoir I, Fontaine J, Tisserant B, Laruelle F, Lounèshadj SA (2017) Beneficial contribution of the arbuscular mycorrhizal fungus, Rhizophagus irregularis, in the protection of Medicago truncatula roots against benzo[a]pyrene toxicity. Mycorrhiza 27(5):465–476. https://doi.org/10.1007/s00572-017-0764-1
Li HS, Sun Q, Zhao SJ, Zhang WH (2000) Principle and techniques of botanic, chemical and physiological experiments, 1st edn. Senior Education Press, Beijing
Li CJ, Nan ZB, Paul VH, Liu Y (2004) A new Neotyphodiums pecies symbiotic with drunken horse grass (Achnatherum inebrians) in China. Mycotaxon 90:141–147
Li HY, Smith FA, Dickson S, Holloway RE, Smith SE (2008) Plant growth depressions in arbuscular mycorrhizal symbiosis: not just caused by carbon drain? New Phytol 178(4):852–862. https://doi.org/10.1111/j.1469-8137.2008.02410.x
Liu Q, Parsons AJ, Xue H, Fraser K, Ryan GD, Newman JA, Rasmussen S (2011) Competition between foliar Neotyphodium lolii endophytes and mycorrhizal Glomus spp. fungi in Lolium perenne depends on resource supply and host carbohydrate content. Funct Ecol 25(4):910–920. https://doi.org/10.1111/j.1365-2435.2011.01853.x
López-Ráez JA, Verhage A, Fernández I, García JM, Azcón-Aguilar C, Flors V, Pozo MJ (2010) Hormonal and transcriptional profiles highlight common and differential host responses to arbuscular mycorrhizal fungi and the regulation of the oxylipin pathway. J Exp Bot 61(10):2589–2601. https://doi.org/10.1093/jxb/erq089
Ma MZ, Nan ZB (2011) Effect of fungal endophytes against rust disease of perennial ryegrass (Lolium perenne) on growth and physiological indices. Acta Pratacult Sin 20:150–156
Ma MZ, Christensen MJ, Nan ZB (2015) Effects of the endophyte Epichloë festucae var. lolii of perennial ryegrass (Lolium perenne) on indicators of oxidative stress from pathogenic fungi during seed germination and seedling growth. Eur J Plant Pathol 141(3):571–583. https://doi.org/10.1007/s10658-014-0563-x
Marschner H, Dell B (1994) Nutrient uptake in mycorrhizal symbiosis. Plant Soil 159(1):89–102. https://doi.org/10.1007/BF00000098
Martínez-Medina A, Pascual JA, Lloret E, Roldán A (2009) Interactions between arbuscular mycorrhizal fungi and Trichoderma harzianum, and their effects on Fusarium wilt in melon plants grown in seedling nurseries. J Sci Food Agr 89(11):1843–1850. https://doi.org/10.1002/jsfa.3660
Maya MA, Matsubara Y (2013) Tolerance to Fusarium wilt and anthracnose diseases and changes of antioxidative activity in mycorrhizal cyclamen. Crop Prot 47:41–48. https://doi.org/10.1016/j.cropro.2013.01.007
McLaughlin MJ, Lancaster PA, Sale PG, Uren NC, Peverill KI (1994) Comparison of cation/anion exchange resin methods for multi-element testing of acidic soils. Soil Res 32(2):229–240. https://doi.org/10.1071/SR9940229
Müller J (2003) Artificial infection by endophytes affects growth and mycorrhizal colonisation of Lolium perenne. Funct Plant Biol 30(4):419–424. https://doi.org/10.1071/FP02189
Nan ZB (1995) Fungicide seed treatments of sainfoil control seed-borne and root-invading fungi. New Zeal J Agr Res 38(3):413–420. https://doi.org/10.1080/00288233.1995.9513144
Novas MV, Cabral D, Godeas AM (2005) Interaction between grass endophytes and mycorrhizas in Bromus setifolius from Patagonia, Argentina. Symbiosis 40:23–30
Novas MV, Iannone LJ, Godeas AM, Scervino JM (2011) Evidence for leaf endophyte regulation of root symbionts: effect of Neotyphodium, endophytes on the pre-infective state of mycorrhizal fungi. Symbiosis 55(1):19–28. https://doi.org/10.1007/s13199-011-0140-4
Omacini M, Eggers T, Bonkowski M, Gang AC, Jones TH (2006) Leaf endophytes affect mycorrhizal status and growth of co-infected and neighbouring plants. Funct Ecol 20(2):226–232. https://doi.org/10.1111/j.1365-2435.2006.01099.x
Parsaeian M, Mirlouhi AF, Almajid RA, Baradaran M (2006) The morphological changes affected by endophytes on induction of cold tolerance in two species of festuca. Iranian J Crop Sci. 8:139–152
Phillips JM, Hayman DS (1970) Improved procedures for clearing roots and staining parasitic and vesicular-arbuscular mycorrhizal fungi for rapid assessment of infection. T British Mycol Soc 55(1):158–IN118. https://doi.org/10.1016/S0007-1536(70)80110-3
Pineda A, Zheng SJ, van Loon JJ, Pieterse CM, Dicke M (2010) Helping plants to deal with insects: the role of beneficial soil-borne microbes. Trends Plant Sci 15(9):507–514. https://doi.org/10.1016/j.tplants.2010.05.007
Popay AJ, Hume DE (2011) Endophytes improve ryegrass persistence by controlling insects. Pasture Persistence-Grassl Res PractSer 15:149–156
Sabzalian MR, Mirlohi A, Sharifnabi B (2012). Reaction to powdery mildew fungus, Blumeria graminis, in Endophyte-infected and endophyte-free tall and meadow fescues. Australas plant path 41:565–572
Saldajeno MGB, Hyakumachi M (2011) The plant growth-promoting fungus Fusarium equiseti and the arbuscular mycorrhizal fungus Glomus mosseae stimulate plant growth and reduce severity of anthracnose and damping-off diseases in cucumber (Cucumis sativus) seedlings. Ann Appl Biol 159(1):28–40. https://doi.org/10.1111/j.1744-7348.2011.00471.x
Schroeder MS, Janos DP (2005) Plant growth, phosphorus nutrition, and root morphological responses to arbuscular mycorrhizas, phosphorus fertilization, and intraspecific density. Mycorrhiza 15(3):203–216. https://doi.org/10.1007/s00572-004-0324-3
Smith SE, Read DJ (1996) Mycorrhizal symbiosis, 3rd edn. Academic, London
Spatafora JW, Chang Y, Benny GL, Lazarus K, Smith ME, Berbee ML (2016) A phylum-level phylogenetic classification of zygomycete fungi based on genome-scale data. Mycologia 108(5):1028–1046. https://doi.org/10.3852/16-042
Strickland JR, Bailey EM, Abney LK, Oliver JW (1996) Assessment of the mitogenic potential of the alkaloids produced by endophyte (Acremonium coenophialum)-infected tall fescue (Festuca arundinacea) on bovine vascular smooth muscle in vitro. J Anim Sci 74(7):1664–1671. https://doi.org/10.2527/1996.7471664x
Tapper BA, Latch GCM (1999) Selection against toxin production in endophyte-infected perennial ryegrass. Grassl Res Pract. Ser 7:107–111
Tian P, Nan ZB, Li CJ German(2008) Effect of the endophyte Neotyphodium lolii on susceptibility and host physiological response of perennial ryegrass to fungal pathogens. Eur J Plant Pathol 122(4):593–602. https://doi.org/10.1007/s10658-008-9329-7
Velikova V, Yordanov I, Edreva A (2000) Oxidative stress and some antioxidant systems in acid rain-treated bean plants: protective role of exogenous polyamines. Plant Sci 151(1):59–66. https://doi.org/10.1016/S0168-9452(99)00197-1
Vicari M, Hatcher PE, Ayres PG (2002) Combined effect of foliar and mycorrhizal endophytes on an insect herbivore. Ecol 83(9):2452–2464. https://doi.org/10.1890/0012-9658(2002)083[2452:CEOFAM]2.0.CO;2
Wang JT, Zhao EZ (2014) The grass planting situation in 2013 in China. China Anim Ind 24:33–34
Wang XY, Zhou Y, Ren AZ, Gao YB (2014) Effect of endophyte infection on fungal disease resistance of Leymus chinensis. Acta Ecol Sin 34:6789–6796
West CP, Izekor E, Turner KE, Elmi AA (1993) Endophyte effects on growth and persistence of tall fescue along a water-supply gradient. Agron J 85(2):264–270. https://doi.org/10.2134/agronj1993.00021962008500020019x
Whipps JM (2004) Prospects and limitations for mycorrhizas in biocontrol of root pathogens. Can J Bot 82(8):1198–1227. https://doi.org/10.1139/b04-082
XL W, XP G, MY S, Yue JJ (2008) Study on contents of cellulose, lignin and activities of POD, PAL in excised bamboo shoots of phyllostachysedulis. Forest Res 21:697–701
Zaefarian F, Rezvani M, Ardakani MR, Rejali F, Miransari M (2013) Impact of mycorrhizae formation on the phosphorus and heavy metal uptake of alfalfa. Commun Soil Sci Plan 44(8):1340–1352. https://doi.org/10.1080/00103624.2012.756505
Zambolim L, Schenck NC (1983) Reduction of the effects of pathogenic, root-infecting fungi on soybean by the mycorrhizal fungus, Glomus mosseae. Phytopathology 73(10):1402–1405. https://doi.org/10.1094/Phyto-73-1402
Zhang GL, Dai QG, Zhang HC, Ling L, Diao SY (2006) Influences of silicon and inoculation with Rhizoctonia solanion membrane lipid peroxidation and defense enzyme activities in rice leaves. J Yangzhou Univ 27:49–53
Zhang XX, Li CJ, Nan ZB (2009) Effects of cadmium stress on growth and anti-oxidative systems in Achnatherum inebrians symbiotic with Neotyphodium gansuense. J Hazard Mater 175:703–709
Zhang W, Card SD, Mace WJ, Christensen MJ, Mcgill CR, Matthew C (2017) Defining the pathways of symbiotic Epichloë colonization in grass embryos with confocal microscopy. Mycologia 109(1):153–161. https://doi.org/10.1080/00275514.2016.1277469
Zheng Y, Liu YH, Ge JY, Wang XY, Liu LJ, ZB B, Liu P (2010) Resveratrol protects human lens epithelial cells against H2O2 induced oxidative stress by increasing catalase, SOD-1, and HO-1 expression. Mol Vis 16:1467–1474
Zhu YG, Laidlaw AS, Christie P, Hammond MER (2000) The specificity of arbuscular mycorrhizal fungi in perennial ryegrass-white clover pasture. Agric Ecosyst Environ 77(3):211–218. https://doi.org/10.1016/S0167-8809(99)00087-0
Acknowledgements
This research was financially supported by the National Natural Science Foundation of China (31100368), China Agriculture Research System (CARS-22 Green manure), and the National Basic Research Program of China (973) (2014CB138702).
Author information
Authors and Affiliations
Corresponding author
Electronic supplementary material
Supplementary Fig S1
Shoot height of perennial ryegrass with (E+) and without (E−) grass endophyte and colonized by Claroideoglomus etunicatum (AM) or non-mycorrhizal (NM) before Bipolaris sorokiniana inoculation. Mean ± SEM of four replicates. Markers adjacent to the same lowercase letter do not differ significantly at the same day between treatments at P ≤ 0.05 by Tukey’s HSD (DOCX 60 kb)
Supplementary Fig S2
Root/shoot ratio of perennial ryegrass with (E+) and without (E−) grass endophyte and colonized by Claroideoglomus etunicatum (stippled bars) or non-mycorrhizal (open bars) at harvest. Mean ± SEM of four replicates. B = Bipolaris sorokiniana applied, NB = no B. sorokiniana. Bars topped by the same lowercase letter do not differ significantly between treatments at P ≤ 0.05 by Tukey’s HSD. See Table 1 for ANOVA results (DOCX 48 kb)
Supplementary Fig S3
Shoot dry weight (a), root dry weight (b), and root length (c) of perennial ryegrass with (E+) and without (E−) grass endophyte and colonized by Claroideoglomus etunicatum (stippled bars) or non-mycorrhizal (open bars) at harvest. Mean ± SEM of four replicates. B = Bipolaris sorokiniana applied, NB = no B. sorokiniana. Bars topped by the same lower case letter do not differ significantly between treatments at P ≤ 0.05 by Tukey’s HSD. See Table 1 for ANOVA results (DOCX 152 kb)
Supplementary Fig S4
Root P concentration of perennial ryegrass with (E+) and without (E−) grass endophyte and colonized by Claroideoglomus etunicatum (stippled bars) or non-mycorrhizal (open bars) at harvest. Mean ± SEM of four replicates. B = Bipolaris sorokiniana applied, NB = no B. sorokiniana. Bars topped by the same lowercase letter do not differ significantly between treatments at P ≤ 0.05 by Tukey’s HSD. See Table 1 for ANOVA results (DOCX 47 kb)
Supplementary Fig S5
Superoxide dismutase (SOD) (a), catalase (CAT) (b), and polyphenol oxidase (PPO) (c) enzyme activity of perennial ryegrass with (E+) and without (E−) grass endophyte and colonized by Claroideoglomus etunicatum (stippled bars) or non-mycorrhizal (open bars) at harvest. Mean ± SEM of four replicates. B = Bipolaris sorokiniana applied, NB = no B. sorokiniana. Bars topped by the same lowercase letter do not differ significantly between treatments at P ≤ 0.05 by Tukey’s HSD. See Table 1 for ANOVA results (DOCX 128 kb)
Rights and permissions
About this article
Cite this article
Li, F., Guo, Y., Christensen, M.J. et al. An arbuscular mycorrhizal fungus and Epichloë festucae var. lolii reduce Bipolaris sorokiniana disease incidence and improve perennial ryegrass growth. Mycorrhiza 28, 159–169 (2018). https://doi.org/10.1007/s00572-017-0813-9
Received:
Accepted:
Published:
Issue Date:
DOI: https://doi.org/10.1007/s00572-017-0813-9