Abstract
Heterotrophic or mixotrophic culture of microalgae is feasible alternative approach to avoid light limitation in autotrophic culture. However, only a few kinds of organic carbon sources are available for algal culture. Disaccharides, such as sucrose, are difficult to be utilized by microalgae under both heterotrophic and mixotrophic conditions. In this study, a symbiotic yeast was accidentally found in a contaminated algal suspension. The symbiotic yeast was isolated and identified as Cryptococcus sp. This yeast was able to extracellularly hydrolyze sucrose and accumulated monosaccharides in the medium. It can enhance algal growth using sucrose as the carbon source at both heterotrophic and mixotrophic modes when mix-cultured with Chlorella pyrenoidosa. The highest algal cell density of 118.8 × 106 and 151.2 × 106 cells/mL was achieved with a final algal percentage of 83.5 and 93.2% at heterotrophic and mixotrophic culture, respectively. Furthermore, the protein and lipid content was significantly enhanced by mix-culture C. pyrenoidosa with Cryptococcus YZU-1. The fatty acid accumulated in this co-culture system was suitable for the production of biodiesel. This symbiotic yeast solved the problem that C. pyrenoidosa cannot heterotrophically or mixotrophically utilize sucrose. A high algae density was obtained and the protein and lipid accumulation were also significantly enhanced. This study provided a novel approach for production of protein or lipid-rich biomass using sucrose or sucrose-rich wastes as the carbon source.
Similar content being viewed by others
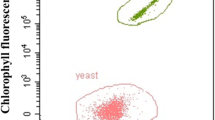
Explore related subjects
Discover the latest articles, news and stories from top researchers in related subjects.Avoid common mistakes on your manuscript.
Introduction
In recent years, microalgae represent an important raw material to produce a wide range of products, including various types of fuel, carbohydrates, pigments, proteins, biomaterials, and other bioproducts [1]. Even though microalgae can be a potential source of various bioproducts, mass cultivation of microalgal biomass is a major challenge to be overcome [2]. As the most common cultivation mode, photoautotrophic culture of microalgae faces many limitations, such as the light limitation, the high cost of the closed photobioreactor, the low biomass concentration, et al. [3]. A feasible alternative approach to avoid these issues is heterotrophic culture microalgae using organic compounds as the carbon sources. By eliminating the light requirements, much higher biomass productivity is achieved in heterotrophic culture along with a shorter culture period compared with photoautotrophic culture [4]. Besides, mixotrophic culture of microalgae can also significantly enhance cell growth and improve the efficient use of light or eliminate its requirement. Due to the excellent culture performance compared to other substrates, glucose is the most commonly used carbon source in the heterotrophic culture of microalgae. However, the high cost of glucose sometimes makes heterotrophic culture of microalgae economically unfeasible as the cost of carbon source reaching up to 80% of the total cost of the medium [5]. Besides glucose, only a few other organic carbon sources, such as glycerol and acetate, are available for algal culture. Some sugar-rich by-products or wastes are regarded as promising alternative carbon sources in fermentation industries. Thereinto, the sucrose-rich molasses and bagasse accumulated in sugar production from sugar beets or sugarcane are economical and efficient alternative carbon sources and have been widely used in the fermentation of many products, such as ethanol, succinic acid, isomaltulose, etc. [6]. Using these cheap substrates is helpful to decrease the carbon source cost in heterotrophic or mixotrophic culture of microalgae. However, sucrose, as well as other disaccharides or polysaccharides, is difficult to be utilized by microalgae under heterotrophic conditions [4, 7].
Many yeasts can utilize sucrose by extracellularly hydrolyze sucrose into monosaccharides by invertase. Furthermore, the sucrose hydrolysis rate was much higher than the monosaccharide uptake rate, thus, monosaccharide accumulation were detected [8, 9]. Based on this phenomenon, a co-culture system was developed in our previous study by mix culture of Chlorella pyrenoidosa and Rhodotorula glutinis using sucrose as the carbon source [9]. In this system, C. pyrenoidosa grew well by utilizing monosaccharides which were hydrolyzed from sucrose by R. glutinis. The highest algal cell density reached 111.48 × 106 cells/mL using 10 g/L sucrose. Although this study solved the problem that algal cells cannot heterotrophically grow in sucrose, the final cell number proportion of algal cells was quite low as the highest algal proportion was only 45% [9]. Besides, the algal cells were difficult to be separated from yeast cells. To solve these issues, cell immobilization was applied in the updated co-culture system [10]. Co-cultured with immobilized Saccharomyces cerevisiae, C. pyrenoidosa grew well in the dark using sucrose as only carbon source and a pure algal suspension with a concentration of 2.08 g/L was obtained [10]. However, cell immobilization will inevitably complicate the culture process and increase the culture cost.
In many microalgal cultivation systems, microalgae always co-exist with bacteria or other microorganisms [11]. Besides, the relationships between microalgae, bacteria, fungi, and other microorganisms are quite complex which probably include cooperation, competition, parasitism, mutualism, commensalism, syntrophy, and antagonism [12, 13]. However, simple synthetic microbial cooperation systems have been widely developed enlightened by natural communities and they can help to enhance the biomass and product accumulation in certain cases [13]. Compared to an axenic culture of microalgae, co-culture algal cells with other microorganism may be also beneficial to improve biomass productivity based on the synergistic mutualism between them [11]. The synergistic mutualism effect between microalgae and other microorganism is usually through the metabolites and resources exchange or signal communication [11]. Metabolites and resource exchange is the most commonly used mechanism in co-culture of microalgae with other microorganism, such as the CO2/O2 exchange between algae and co-cultured bacteria [14], nitrogen supply for microalgae by mix cultured with nitrogen-fixing cyanobacteria [15], providing indole-3-acetic acid (IAA) to promote algal growth by co-culture with bacteria which can secrete IAA [11], providing vitamin-B12 to synthesize methionine for vitamin auxotrophic algae [16, 17], etc. Besides, co-cultured microalgae with fungal is also a promising algal harvesting method as some filamentous fungal species have self or induced flocculation activities and can easily harvest algal cells via bio-flocculation [18].
In this study, a symbiotic yeast was accidentally found in a contaminated algal suspension. The contaminated C. pyrenoidosa can well grow using sucrose as the sole carbon source under dark condition. The symbiotic yeast was separated and identified firstly. Then, it was used in the co-culture of C. pyrenoidosa using sucrose as the sole carbon source at both dark (heterotrophic culture) and light (mixotrophic culture) conditions. Cell growth, sucrose utilization, and cell composition were measured under different algal/yeast ratio. This study aimed to provide an alternative approach for the heterotrophic culture microalgae using sucrose. It has great potential for decreasing the cost of carbon source in the heterotrophic culture of microalgae.
Materials and methods
Microorganism strains and inoculum culture
Chlorella pyrenoidosa was purchased from Freshwater Algae Culture Collection at the Institute of Hydrobiology (FACHB) and stored in agar slants BG-11 medium. The stored cells were first activated in 250 mL Erlenmeyer flasks containing 100 mL liquid BG-11 medium and incubated at 25 °C in an orbital shaker at 120 rpm. Algal cells were photoautotrophically cultured under a 16 h light period per day with 35 μmol m−2 s−1 for 2 weeks. The pre-cultured cells were then transferred to a heterotrophic system of liquid BG-11 medium with 1% glucose and cultivated for 5 days under a dark environment at 25 °C in an orbital shaker at 150 rpm. The heterotrophic cultured cells were used as the inoculum for following pure culture and co-culture experiments.
Separation and identify of symbiotic yeast
The symbiotic yeast was separated from a contaminated culture of C. pyrenoidosa. The contaminated algal cells were originally kept in our lab as usual culture, 25 °C and a 16 h light period per day with 35 μmol m−2 s−1 illumination. They were found well grown in sucrose-contained medium. Single colonies of the symbiotic yeast were obtained by serial streaking of the culture broth on YPD agar medium (1% glucose, 1% peptone, 0.5% yeast extract, and 2% agar). The isolated colonies were further subcultured three times on YPD plates by incubating at 25 °C for 48 h. The cellular morphology and colony characteristics of the obtained cells were observed using a microscope (Olympus BX53, Japan) and a stereomicroscope (Leica EZ4W, Germany), respectively. To further identify the cell, the genome DNA was extracted using a Rapid Yeast Genomic DNA Isolation Kit (Sangon Biotech, China) according to the manufacturer’s instruction. PCR amplification of 26S rDNA gene fragments using two specific primers (NL-1: 5′-GCATATCAATAAGCGGAGGAAAAG-3′; NL-4: 5′-GGTCCGTGTTTCAAGACGG-3′). After been detected using electrophoresis, the PCR products were sequenced. Multiple sequence alignment and phylogenetic analysis was conducted using BLAST and neighbor-joining method (MEGA 7.0), respectively.
To investigate the carbon source preference of the isolated yeast, sucrose, glucose, and fructose was used at a concentration of 10 g/L. BG-11 medium added carbon source was used as the medium. Cell growth and sugar utilization was measured every 12 h. Cell density was determined by count the cell numbers using a hemacytometer and a microscope (Olympus BX53, Japan) and the sugar was analyzed using a HPLC according to the method reported by Wang et al. [9].
Co-culture of microalgae and yeast
Liquid BG-11 medium added 10 g/L sucrose was used in following experiments. The initial algal cell density was 2 × 106 cells/mL. Five initial ratios of microalgae and yeast, 20:1, 30:1, 40:1, 50:1, and 60:1, were set based on cell number. The cultures were incubated at 25 °C in an orbital shaker at 150 rpm under both heterotrophic and mixotrophic (35 μmol m−2 s−1) conditions.
Analytic methods
Cell growth was determined by count the cell numbers using a hemacytometer and a microscope (Olympus BX53, Japan). Carbohydrate, lipid, and fatty acid were analyzed according to the method reported by Wang et al. [9]. Protein was calculated through measurement of total nitrogen content. In detail, added 2 mL alkaline potassium persulfate into 1 mL algal broth and the mixture was treated at 121 °C for 30 min. After cool down to room temperature, 1 mL 10% HCl (v/v) was added into the mixture. Absorbance at 220 nm and 275 nm was measured using a UV spectrophotometer (Philes, China). Protein content was calculated based on a standard curve which was made using bovine serum albumin.
Statistical analysis
Data were presented as mean ± standard error of the mean based on three parallel experiments. The statistical significances were analyzed by one-way analysis of variance (ANOVA) (P < 0.05) using SPSS statistics 17.0 (IBM, USA).
Results and discussion
Isolation and identification of the symbiotic yeast
The colony and cellular morphology of the isolated symbiotic yeast are displayed in Fig. 1a, b. The colony was oyster white and the surface of the colony was smooth and moist. The cell was oval in shape with a size of about 2–5 μm. The colony and cellular morphology were in line with the characteristics of yeast [19]. To further identify this microorganism, the neighbor-jointing phylogenetic tree based on 26S rDNA analysis was constructed (Fig. 1c). The microorganism showed 99% similarity with Cryptococcus uzbekistanensis strain ML114 belonging to Cryptococcus sp. Based on above results, this isolated symbiotic yeast was identified as a Cryptococcus sp. and named Cryptococcus YZU-1.
Sucrose, glucose, and fructose were tested in the culture of Cryptococcus YZU-1. Cells grown in glucose reached the highest cell density while fructose present the worst culture performance (Fig. 2a). The result of sugar analysis also indicated that the uptake rate of fructose was lower than that of glucose and sucrose (Fig. 2b). In the culture of Cryptococcus YZU-1 using sucrose, accumulation of glucose and fructose was detected during the culture period. This result suggested that like many other yeast species, such as Phaffia rhodozyma [8], Rhodotorula glutinis [9], S. cerevisiae [20] and Schwanniomyces occidentalis [21], Cryptococcus YZU-1 was able to secrete invertase and extracellularly hydrolyze sucrose into glucose and fructose. Besides, the extracellular glucose was exhausted at 36 h while extracellular fructose kept a high concentration after 48 h. This indicated that glucose was used in preference for Cryptococcus YZU-1 rather than fructose similar with other yeast species [8, 9].
Co-culture of C. pyrenoidosa and Cryptococcus YZU-1
The growth curves of algal cells co-cultured with Cryptococcus YZU-1 at different initial ratio under heterotrophic and mixotrophic cultures are displayed in Fig. 3a, b, respectively. C. pyrenoidosa cannot survive at heterotrophic culture using sucrose as the sole carbon source. And even under mixotrophic culture, the growth of C. pyrenoidosa was equivalent to that in autotrophic culture. These results have been confirmed in many algal species, including Chlamydomonas reinhardtii, Phormidium sp., C. zofingiensis, and Botryococcus braunii [6, 22,23,24]. When co-cultured with Cryptococcus YZU-1, C. pyrenoidosa could effectively accumulate algal biomass at both heterotrophic and mixotrophic cultures. The highest algal cell density, 118.8 × 106 cells/mL, was achieved with an initial algae/yeast ratio of 30:1 at heterotrophic culture while the highest algal cell density, 151.2 × 106 cells/mL, was achieved with an initial algae/yeast ratio of 40:1 at mixotrophic culture. As the comparison, the final cell density in mixotrophic culture without yeast was only 30.7 × 106 cells/mL. It has been reported that some algal species, including Chlorella sp. Y8-1 and B. braunii, could significantly accumulate biomass using sucrose at mixtrophic cultures [24, 25]. In contrast, some studies reported that the growth of C. pyrenoidosa and C. reinhardtii wild-type strain CC-124 was poor in sucrose even under mixtrophic cultures [6, 7]. Still, the mechanism of sucrose utilization in green algae remains unclear [7]. In other microorganisms, sucrose was utilized through different mechanisms, which were mainly divided into two pathways: (1) the disaccharide was first hydrolyzed by the specific extracellular hydrolase into monosaccharide and then, the cells utilize the monosaccharide through the hexose transport system; and (2) the disaccharide was directly transported into intracellular by specific disaccharide carrier and then hydrolyzed and assimilated within cells [26, 27]. It was speculated that C. pyrenoidosa used in this study may lack both extracellular hydrolase and sucrose transporter, thus, cells were difficult to growth using sucrose either at heterotrophic culture or mixotrophic culture.
Beside cell density, high algal proportion was obtained at the end of co-culture (Fig. 3c). In heterotrophic cultures, the final algal proportion in all cultures was more than 83% except that of culture at an initial alga/yeast ratio of 20:1. In mixotrophic cultures, the final algal proportion in all cultures was no less than 91% except that of culture at an initial alga/yeast ratio of 20:1, 88%. The obtained final algal proportion in this study was significantly higher than our previous study [9]. In the co-culture of C. pyrenoidosa with R. glutinis using sucrose as the sole carbon source at heterotrophic mode, the highest final algal proportion was only 45% even the initial algal proportion was as high as 95.24% [9]. Unlike our previous study, in current study, C. pyrenoidosa was the dominant species in the co-culture system all through the culture period. This was mainly due to the low growth rate of Cryptococcus YZU-1. As shown in Fig. 3A, the final cell density of Cryptococcus YZU-1 reached 115.9 × 106, 134.7 × 106, and 109.2 × 106 cells/mL using sucrose, glucose, and fructose, respectively. As the contrast, the final cell density of 186.1 × 106, 248.3 × 106, and 229.0 × 106 cells/mL were obtained in the culture of R. glutinis using sucrose, glucose, and fructose, respectively. The lower final yeast cell density indicated that yeast cells utilize fewer nutrients, including carbon source, nitrogen source and other substrates. This is benefit for the accumulation of more algal biomass.
Carbohydrate utilization during the culture period
In the pure culture of C. pyrenoidosa using sucrose, no sucrose was utilized by algal cells at heterotrophic mode (Fig. 4a). In the mixotrophic culture, the sucrose concentration was decreased from 10 to 7 g/L after 6 days (Fig. 4b). However, no monosaccharides were detected in the medium. It was hypothesized that the decreasing of sucrose was mainly due to the sucrose absorption by algal cells as the cell biomass slightly increased through photosynthesize process in mixotrophic culture. When co-cultured C. pyrenoidosa with Cryptococcus YZU-1, sucrose was efficiently utilized in both heterotrophic cultures and mixotrophic cultures and it was exhausted at the end of the culture (Fig. 4a, b). Besides, abundant monosaccharides were accumulated in the medium during the culture process (Fig. 4c–f). Furthermore, the concentration of glucose was much lower than that of fructose in all cultures. The highest glucose concentration in all cultures was less than 1 g/L and its final concentration was less than 0.4 g/L. In contrast, fructose was continual accumulated during the culture process in all cultures. It has been investigated that glucose was used in preference for Cryptococcus YZU-1 rather than fructose (Fig. 2b). And for C. pyrenoidosa, glucose was also a better carbon source compared with many other organic carbon sources [4, 7]. When glucose was presented in the medium, fructose was difficult to be utilized by both algal and yeast cells. Therefore, glucose was kept in a low level while fructose was continual accumulated with the hydrolysis of sucrose.
Cell composition
The main cell components, protein and lipid, of cells cultured in different conditions were analyzed. Pure culture of C. pyrenoidosa using sucrose as the carbon source at mixotrophic culture was set as control due to algal cells were unable to survive at heterotrophic condition. As shown in Fig. 5, the protein content of control was 41.05%, which is lower than the protein content of mixed cell biomass obtained in co-culture at both heterotrophic and mixotrophic modes. Besides, higher protein content of the mixed cell biomass was achieved at cultures with a higher initial yeast proportion in both heterotrophic and mixotrophic cultures. However, the protein content of Cryptococcus YZU-1 was only 28.36%, which is much lower than that of C. pyrenoidosa. From the above results, we concluded that the protein content of C. pyrenoidosa was significantly increased by mixed culture with Cryptococcus YZU-1. It has been widely reported that the synergistic mutualism between microalgae and yeast was beneficial for the accumulation of biomass as well as some specific cell components. Rajapitamahuni et al. [28] reported that the protein content increased to 30% in co-culture of C. variabilis ATCC 12198 with siderophore-producing bacterium Idiomarina loihiensis RS14 compared with axenic microalgae. Besides, Li et al. [29] reported that the co-cultivation system of C. pyrenoidosa and R. glutinis gained 59.8% (w/w) protein content and the enhancement of protein accumulation was mainly due to the oxygen/carbon dioxide exchange balance.
Apart from protein, the lipid content of cells obtained in the co-culture system was also higher than that of control. The highest lipid content reached 27.1% (w/w) and 28.2% (w/w) in heterotrophic and mixotrophic cultures, respectively, while the lipid content of control was only 23.5% (w/w). This result indicated that the lipid content of C. pyrenoidosa was also significantly enhanced by mixed culture with the yeast considering Cryptococcus sp. is not belonging to oleaginous yeast and its lipid content was only 5.8% (w/w). Similar result has been widely observed in the mixed culture of Spirulina platensis and R. glutinis [30], C. vulgaris and R. glutinis [31], Scenedesmus obliquus and R. glutinis [32], C. pyrenoidosa and R. glutinis [9], C. pyrenoidosa and immobilized S. cerevisiae [10]. The enhancement of lipid accumulation was more likely based on the synergistic effects on substance exchange between microalgae and the symbiotic yeast. Seven kinds of fatty acids with 16 and 18 carbon atoms were detected in the cells (Fig. 6a). It has been reported that fatty acids with 16 and 18 carbon atoms are the ideal components for biodiesel [33]. Among these fatty acids, palmitic acid (16:0) and linoleic acid (18:2) are most abundant components which account for more than 20% of the total fatty acid in all cultures. However, the fatty acid profile of cells obtained in co-culture system was distinct from that of control as the percentage of palmitic acid (16:0), oleic acid (18:1), and linoleic acid (18:2) in co-culture system was higher than that of control while with a lower percentage of hexadecadienoic acid (16:2) and linolenic acid (18:3). As shown in Fig. 6b, a higher percentage of monounsaturated fatty acids (MUFAs) was achieved in co-culture system, while cells in control has a higher polyunsaturated fatty acids (PUFAs) percentage. It has been reported that the fatty acid composition changes during various growth phases. Higher amount of saturated fatty acids (SFAs) and MUFAs were obtained in the late stationary growth phase with simultaneous lowering down of PUFAs [34]. In this study, the culture period in mixed culture was shorter than that of control and the growth reached stationary phase at 5 days while the growth period in autotrophic culture usually needs 2 weeks. Deshmukh et al. [34] reported that a mixture of various types of fatty acids, including SFA, MUFA and PUFA is more desirable for the production of biodiesel rather than higher percentage of just one type of fatty acid as the presence of only saturated or polyunsaturated fatty acids in biodiesel will lead to extreme values of properties. This indicated that the fatty acid accumulated in this co-culture system is suitable for the production of biodiesel.
The protein and lipid productivity in this study was compared with that of cells cultured using equivalent glucose [35]. As shown in Table 1, higher final biomass concentration was achieved in mixed culture at same culture mode. Besides, the protein content obtained in this study was significantly higher than that of pure culture using glucose. It has been reported that C. zofingiensis accumulated more starch when cultured using glucose at both heterotrophic and mixotrophic conditions compared with that of cells cultured at autotrophic culture [36]. This result showed that the mixed culture using sucrose as the carbon source is beneficial to protein accumulation compared with cultures using glucose. Combined the higher biomass with higher protein content, the protein productivities in this study were 2.28 and 1.75 times higher than that of cultures using glucose at heterotrophic and mixotrophic cultures, respectively. The lipid content in this study was also comparable to that of cultures using glucose. However, the lipid productivity in this study was much higher due to the higher biomass concentration. The lipid productivities reached 124.3 and 165.4 mg/L/day at heterotrophic and mixotrophic cultures, respectively.
Suitable application of this system
Compared with co-culture with free Rhodotorula glutinis, the final algal proportion was greatly increased [9]. Besides, the culture process is quite simpler than co-culture with immobilized yeast [10]. Although a small percentage of yeast was still remained in the algal suspension, this culture system is suitable for the production of various algal-based products, e.g. lipids, protein, by heterotrophic or mixotrophic culture of microalgae using sucrose or sucrose-rich wastes at any scales. This may be helpful to decrease the cost of carbon source in the heterotrophic or mixotrophic cultivation of microalgae.
Conclusions
A symbiotic yeast was isolated from a contaminated algal suspension and identified as Cryptococcus sp. By extracellularly hydrolyze sucrose into monosaccharides, this yeast was able to enhance algal growth using sucrose as the sole carbon source at both heterotrophic and mixotrophic modes. The highest algal cell density of 118.8 × 106 and 151.2 × 106 cells/mL was achieved with a final algal cell percentage of 83.5 and 93.2% at heterotrophic and mixotrophic culture, respectively. Besides, the protein and lipid content was significantly enhanced by mixed C. pyrenoidosa with Cryptococcus YZU-1 and the fatty acid accumulated in this co-culture system is suitable for the production of biodiesel. The strategy provided in this study is suitable for the production of various algal-based products by heterotrophic culture of microalgae using sucrose or sucrose-rich wastes, e.g. waste molasses and sugarcane bagasse, at any scales. Besides, the cost of carbon source in the heterotrophic cultivation of microalgae may also be decreased.
Abbreviations
- IAA:
-
Indole-3-acetic acid
- MUFA:
-
Monounsaturated fatty acid
- PUFA:
-
Polyunsaturated fatty acid
- SFA:
-
Saturated fatty acid
References
Chandra R, Iqbal HMN, Vishal G, Lee HS, Nagra S (2019) Algal biorefinery: a sustainable approach to valorize algal-based biomass towards multiple product recovery. Bioresour Technol 278:346–359. https://doi.org/10.1016/j.biortech.2019.01.104
Hu J, Nagarajan D, Zhang Q, Chang JS, Lee DJ (2018) Heterotrophic cultivation of microalgae for pigment production: a review. Biotechnol Adv 36:54–67. https://doi.org/10.1016/j.biotechadv.2017.09.009
Zhan J, Rong J, Wang Q (2017) Mixotrophic cultivation, a preferable microalgae cultivation mode for biomass/bioenergy production, and bioremediation, advances and prospect. Int J Hydrogen Energy 42:8505–8517. https://doi.org/10.1016/j.ijhydene.2016.12.021
Perez-Garcia O, Escalante FME, de-Bashan LE, Bashan Y (2011) Heterotrophic cultures of microalgae: metabolism and potential products. Water Res 45:11–36. https://doi.org/10.1016/j.watres.2010.08.037
Li X, Xu H, Wu Q (2007) Large-scale biodiesel production from microalga Chlorella protothecoides through heterotrophic cultivation in bioreactors. Biotechnol Bioeng 98:764–771. https://doi.org/10.1002/bit.21489
Wu L, Wu S, Qiu J, Xu C, Li S, Xu H (2017) Green synthesis of isomaltulose from cane molasses by Bacillus subtilis WB800-pHA01-palI in a biologic membrane reactor. Food Chem 229:761–768. https://doi.org/10.1016/j.foodchem.2017.03.001
Zhang W, Zhang P, Sun H, Chen M, Lu S, Li P (2014) Effects of various organic carbon sources on the growth and biochemical composition of Chlorella pyrenoidosa. Bioresour Technol 173:52–58. https://doi.org/10.1016/j.biortech.2014.09.084
Kilian SG, Sutherland FCW, Meyer PS, Du Preez JC (1997) Transport-limited sucrose utilization and neokestose production by Phaffia rhodozyma. Biotechnol Lett 18:975–980
Wang SK, Wu Y, Wang X (2016) Heterotrophic cultivation of Chlorella pyrenoidosa using sucrose as the sole carbon source by co-culture with Rhodotorula glutinis. Bioresour Technol 220:615–620. https://doi.org/10.1016/j.biortech.2016.09.010
Wang SK, Wang X, Tao HH, Sun XS, Tian YT (2018) Heterotrophic culture of Chlorella pyrenoidosa using sucrose as the sole carbon source by co-culture with immobilized yeast. Bioresour Technol 249:425–430. https://doi.org/10.1016/j.biortech.2017.10.049
Dao GH, Wu GX, Wang XX, Zhang TY, Zhan XM, Hu HY (2018) Enhanced microalgae growth through stimulated secretion of indole acetic acid by symbiotic bacteria. Algal Res 33:345–351. https://doi.org/10.1016/j.algal.2018.06.006
Xue LG, Shang H, Ma P, Wang X, He XY, Niu JB, Wu JL (2018) Analysis of growth and lipid production characteristics of Chlorella vulgaris in artificially constructed consortia with symbiotic bacteria. J Basic Microbiol 58:358–367. https://doi.org/10.1002/jobm.201700594
Amor DR, Bello MD (2019) Bottom-up approaches to synthetic cooperation in microbial communities. Life 9:22. https://doi.org/10.3390/life9010022
Smith RT, Bangert K, Wilkinson SJ, Gilmour DJ (2015) Synergistic carbon metabolism in a fast growing mixotrophic freshwater microalgal species Micractinium inermum. Biomass Bioenergy 82:73–86. https://doi.org/10.1016/j.biombioe.2015.04.023
Foster RA, Kuypers MMM, Vagner T, Paerl RW, Musat N, Zehr JP (2011) Nitrogen fixation and transfer in open ocean diatom-cyanobacterial symbioses. ISME J 5:1484–1493. https://doi.org/10.1038/ismej.2011.26
Haines KC, Guillard RRL (1974) Growth of vitamin B12-requiring marine diatoms in mixed laboratory cultures with vitamin B12-producing marine bacteria. J Phycol 10:245–252. https://doi.org/10.1111/j.1529-8817.1974.tb02709.x
Tandon P, Jin Q, Huang L (2017) A promising approach to enhance microalgae productivity by exogenous supply of vitamins. Microb Cell Fact 16:219. https://doi.org/10.1186/s12934-017-0834-2
Zhou W, Cheng Y, Li Y, Wan Y, Liu Y, Lin X, Ruan R (2012) Novel fungal pelletization-assisted technology for algae harvesting and wastewater treatment. Appl Biochem Biotechnol 167:214–228. https://doi.org/10.1007/s12010-012-9667-y
Shen P, Chen X (2006) Microbiology, 2nd edn. Higher Education Press, Beijing
Fontana A, Ghommidh C, Guiraud JP, Navarro JM (1992) Continuous alcoholic fermentation of sucrose using flocculating yeast. The limits of invertase activity. Biotechnol Lett 14:505–510. https://doi.org/10.1007/BF01023176
Costaglioli P, Meilhoc F, Janatova I, Klein R, Masson J (1997) Secretion of invertase from Schwanniomyces occidentalis. Biotechnol Lett 19:623–627. https://doi.org/10.1023/A:1018326512461
Francisco EC, Franco TT, Wagner R, Jacob-Lopes E (2014) Assessment of different carbohydrates as exogenous carbon source in cultivation of cyanobacteria. Bioprocess Biosyst Eng 37:1497–1505. https://doi.org/10.1007/s00449-013-1121-1
Sun N, Wang Y, Li YT, Huang JC, Chen F (2008) Sugar-based growth, astaxanthin accumulation and carotenogenic transcription of heterotrophic Chlorella zofingiensis (Chlorophyta). Process Biochem 43:1288–1292. https://doi.org/10.1016/j.procbio.2008.07.014
Zhang HF, Wang WL, Li YG, Yang WJ, Shen GM (2011) Mixotrophic cultivation of Botryococcus braunii. Biomass Bioenerg 35:1710–1715. https://doi.org/10.1016/j.biombioe.2011.01.002
Lin TS, Wu JY (2015) Effect of carbon sources on growth and lipid accumulation of newly isolated microalgae cultured under mixotrophic condition. Bioresour Technol 184:100–107. https://doi.org/10.1016/j.biortech.2014.11.005
Reid SJ, Abratt VR (2005) Sucrose utilisation in bacteria: genetic organization and regulation. Appl Microbiol Biotechnol 67:312–321. https://doi.org/10.1007/s00253-004-1885-y
Seiboth B, Pakdaman BS, Hartl L, Kubicek CP (2007) Lactose metabolism in filamentous fungi: how to deal with an unknown substrate. Fungal Biol Rev 1:42–48. https://doi.org/10.1016/j.fbr.2007.02.006
Rajapitamahuni S, Bachani P, Sardar RK, Mishra S (2019) Co-cultivation of siderophore-producing bacteria Idiomarina loihiensis RS14 with Chlorella variabilis ATCC 12198, evaluation of micro-algal growth, lipid, and protein content under iron starvation. J Appl Phycol 31:29–39. https://doi.org/10.1007/s10811-018-1591-2
Li H, Zhong Y, Lu Q, Zhang X, Wang Q, Liu H, Diao Z, Yao C, Liu H (2019) Co-cultivation of Rhodotorula glutinis and Chlorella pyrenoidosa to improve nutrient removal and protein content by their synergistic relationship. RSC Adv 9:14331–14342. https://doi.org/10.1039/C9RA01884K
Xue F, Miao J, Zhang X, Tan T (2010) A new strategy for lipid production by mix cultivation of Spirulina platensis and Rhodotorula glutinis. Appl Biochem Biotechnol 160:498–503. https://doi.org/10.1007/s12010-008-8376-z
Zhang Z, Ji H, Gong G, Zhang X, Tan T (2014) Synergistic effects of oleaginous yeast Rhodotorula glutinis and microalga Chlorella vulgaris for enhancement of biomass and lipid yields. Bioresour Technol 164:93–99. https://doi.org/10.1016/j.biortech.2014.04.039
Yen HW, Chen PW, Chen LJ (2015) The synergistic effects for the co-cultivation of oleaginous yeast-Rhodotorula glutinis and microalgae-Scenedesmus obliquus on the biomass and total lipids accumulation. Bioresour Technol 184:148–152. https://doi.org/10.1016/j.biortech.2014.09.113
Xu H, Miao X, Wu Q (2006) High quality biodiesel production from microalga Chlorella protothecoides by heterotrophic growth in fermenters. J Biotechnol 126:499–507. https://doi.org/10.1016/j.jbiotec.2006.05.002
Deshmukh S, Kumar R, Bala K (2019) Microalgae biodiesel: a review on oil extraction, fatty acid composition, properties and effect on engine performance and emissions. Fuel Process Technol 191:232–247. https://doi.org/10.1016/j.fuproc.2019.03.013
Wang SK, Wang X, Miao J, Tian YT (2018) Tofu whey wastewater is a promising basal medium for microalgae culture. Bioresour Technol 253:79–84. https://doi.org/10.1016/j.biortech.2018.01.012
Zhang Z, Sun D, Wu T, Li Y, Lee Y, Liu J, Chen F (2017) The synergistic energy and carbon metabolism under mixotrophic cultivation reveals the coordination between photosynthesis and aerobic respiration in Chlorella zofingiensis. Algal Res 25:109–116. https://doi.org/10.1016/j.algal.2017.05.007
Acknowledgements
This work was financially supported by the National Natural Science Foundation of China (no. 31802323), Natural Science Foundation of Jiangsu Province, China (no. BK20170495), and High-level Talent Support Program of Yangzhou University.
Author information
Authors and Affiliations
Contributions
YT and XW carried out the experiments, analyzed the data and drafted the manuscript. YC contributed analytic methods. SW designed the experiments, analyzed the data and drafted the manuscript. All authors read and approved the final manuscript.
Corresponding author
Ethics declarations
Conflict of interest
The authors declare that they have no conflict of interest.
Additional information
Publisher's Note
Springer Nature remains neutral with regard to jurisdictional claims in published maps and institutional affiliations.
Rights and permissions
About this article
Cite this article
Tian, YT., Wang, X., Cui, YH. et al. A symbiotic yeast to enhance heterotrophic and mixotrophic cultivation of Chlorella pyrenoidosa using sucrose as the carbon source. Bioprocess Biosyst Eng 43, 2243–2252 (2020). https://doi.org/10.1007/s00449-020-02409-2
Received:
Accepted:
Published:
Issue Date:
DOI: https://doi.org/10.1007/s00449-020-02409-2