Abstract
Populations of a single species may vary substantially in sexually selected traits. However, the majority of studies investigating such variation across populations have focused on traits important for pre-copulatory reproductive success, such as male ornamentation or song. Relatively fewer studies have investigated whether traits important in post-copulatory sexual selection vary across populations. In this study, we examine how sperm and ejaculate quantity and quality in the American horseshoe crab, Limulus polyphemus differ at six sites, from Maine to Florida. Horseshoe crabs from these sites differ in many respects, including average body size, age, and the risk and intensity of sperm competition as measured by the operational sex ratio. We find that sperm and ejaculate quantity and quality significantly differ across this range and that this variation persists after taking body size and age into account. Sperm traits also do not follow strictly linear latitudinal clines. Finally, we find that the site-specific operational sex ratio correlates with changes in sperm and ejaculate quantity. This latter result may indicate that the same processes that lead to variation in post-copulatory sexually selected traits across related species may also promote trait divergence across populations of the same species.
Significance statement
This study describes variation in sperm and ejaculate traits from horseshoe crabs collected at six sites from Maine to Florida. While geographic variation has been described for many traits, relatively few studies have demonstrated that sperm and ejaculate traits follow similar patterns. The variation we found does not follow simple latitudinal patterns, suggesting that other forces may be driving sperm and ejaculate trait divergence across sites. We also have data that suggest sexual selection may play a role in driving sperm trait variation, but we caution that additional sites need to be sampled.
Similar content being viewed by others
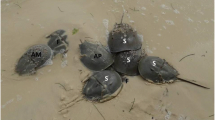
Avoid common mistakes on your manuscript.
Introduction
Morphological, physiological, and behavioral traits often show remarkable variation across populations, and what drives this variation remains an important question in evolutionary biology. Variation across populations also exists for sexually selected traits, such as male ornamentation (Endler and Houde 1995), song structure (Irwin 2000), and mating strategy (Shine and Fitzgerald 1995). However, most studies investigating sexually selected trait variation across populations have focused on traits affecting pre-copulatory reproductive success, i.e., traits that affect mate acquisition. In many systems, post-copulatory reproductive traits play an important role in determining male reproductive success (Snook 2005) but relatively fewer studies have examined how post-copulatory traits vary across populations. Sperm quantity and quality are strongly linked to fitness in systems with high levels of sperm competition (e.g. Hosken et al. 2001; Simmons and Moore 2009), but little is known about the extent to which these traits vary across populations.
A number of studies have shown that sperm competition may influence sperm quantity and quality across closely related species (e.g. Hunter and Birkhead 2002; Fitzpatrick et al. 2009; Kleven et al. 2009; Lüpold et al. 2009a, 2009b). However, while studies have shown that sperm morphology may vary across populations in a wide variety of taxa, e.g., insects (Snook 2001; Pitnick et al. 2003), sea urchins (Manier and Palumbi 2008), birds (Laskemoen et al. 2013; Lüpold 2013), and frogs (Stewart et al. 2016), relatively fewer studies have investigated how sperm quantity or quality vary across populations (but see Evans and Magurran 1999; Hettyey and Roberts 2006; Dziminski et al. 2010; Elgee et al. 2010; Lindholm et al. 2014). Documenting the variation in sperm quantity and quality across populations is a necessary first step in understanding the factors that influence this variation.
Variation in sperm and ejaculate traits has been attributed to a number of sources. Population-specific differences in the strength of sexual selection, as measured by sperm competition risk, have been hypothesized as one contributor to geographic variation in sperm and ejaculate traits (Evans and Magurran 1999; Hettyey and Roberts 2006; Manier and Palumbi 2008; Dziminski et al. 2010). Experimental manipulations have shown that the strength of sexual selection (through forced monogamy) can lead to changes in testes size across generations (Simmons and García‐González 2008). However, direct support for the hypothesis that sexual selection can lead to population variation in sperm and ejaculate traits in natural systems is lacking (see Lüpold et al. 2011). A number of studies have also investigated whether sperm and ejaculate traits vary across a latitudinal cline, but here again, the results are mixed. Sperm quantity and quality vary positively (Pitcher and Stutchbury 1998; Lüpold et al. 2011) or negatively (Pitcher and Stutchbury 1998) with latitude in some systems, while others show no effect of latitude (Pitcher and Stutchbury 1998; Snook 2001). Overall, the evidence for sperm quantity and quality variation across populations is sparse and little is known about the factors that may drive this variation. In this study, we investigate geographic variation in sperm and ejaculate quantity and quality in natural populations of the American horseshoe crab, Limulus polyphemus, and hypothesize factors that may have led to this variation.
In using horseshoe crabs, we take advantage of their broad geographic range, spanning the East Coast and parts of the Gulf of Mexico in the USA with a disjunct population in the Yucatan. Over this range, there are genetically distinct populations (King et al. 2005, 2015) that vary in a number of traits, including body size (Riska 1981; Brockmann and Smith 2009) and average age (Table 1). Both body size and age have been shown to affect sperm quantity within a population of horseshoe crabs (Sasson et al. 2012), and thus these factors may correlate with differences in sperm quantity and quality across populations. The operational sex ratio (OSR, or the ratio of mating males to females in a population, Emlen and Oring 1977) also varies across populations (Brockmann and Smith 2009). Males at sites with a high OSR face higher levels of sperm competition than males from sites with a low OSR do; this difference in sperm competition may drive sperm quantity and quality divergence.
Male horseshoe crabs have two condition-dependent alternative reproductive tactics (Brockmann and Penn 1992). Some males attach to the female and the pair arrives together at the beach to spawn; these males are on average younger and in better condition than are “attached males.” As males age and their condition deteriorates, they switch from acting as attached males to acting as “satellite males” (Brockmann 2002). These males come to the beach without females and search the spawning area for mating pairs or groups to join. Since horseshoe crabs have a terminal molt upon reaching adulthood (Shuster and Sekiguchi 2003; Smith et al. 2010), attached and satellite males only differ in condition and age, not in size. Sperm competition in horseshoe crabs occurs when these satellite males form a mating group around a spawning female and her attached male (Brockmann et al. 1994, 2015; Sasson et al. 2015). When satellite males join a spawning pair, sperm competition occurs among the satellites and between the satellites and the attached male (Brockmann et al. 2000). Since fertilization occurs externally, sperm quantity and quality are likely to play an important role in determining the outcome of sperm competition within a mating group (Stoltz and Neff 2006). Thus, variation across populations in the frequency of satellite males and mating group size may favor population differences in sperm quantity and quality.
Here, we examine the extent to which sperm quantity and quality differ for horseshoe crabs collected at six sites from Maine to Florida. We compare three measures of sperm quantity (ejaculate size, concentration, and sperm per ejaculate) and one measure of sperm quality (velocity) across sites taking into account site differences in body size and male age. We then investigate the role of the OSR in sperm trait variation.
Methods
We conducted this study in six locations along the East and Gulf coasts of the USA: Seahorse Key, Florida (FL); Sapelo Island, Georgia (GA); Slaughter Beach, Delaware (DE); Norwalk, Connecticut (CT); Wellfleet, Massachusetts (MA); and Thomas Point Beach, Maine (ME) (Fig. 1). The onset of the breeding season differs across these locations; as such, we attempted to sample each location during the middle of their respective breeding seasons when males would be most abundant. Horseshoe crabs from FL, GA, and ME are genetically isolated from each other and from our other collection sites (King et al. 2015). The horseshoe crabs from the DE and CT sites likely have a small degree of gene flow (King et al. 2005, 2015). The horseshoe crabs from MA, on the north side of Cape Cod, may differ genetically from the Massachusetts sites that were sampled by King et al. (2015) (Johnson 2016), so the degree of isolation of our MA horseshoe crabs from the mid-Atlantic population to the south is unknown.
The OSR may be used as a proxy for the level of sperm competition within populations and has been shown to affect sperm and ejaculate traits in experimental manipulations (Gage and Barnard 1996; Oppliger et al. 1998; Crudgington et al. 2009). We obtained the OSR at the FL and DE sites from Brockmann and Smith (2009) and the OSR for CT, MA, and ME from unpublished surveys (personal communication: Jennifer Mattei, Katherine Johnson, and Peter Thayer, respectively). These OSR values were calculated from breeding surveys that spanned numerous tides over one whole season (CT, MA, and ME) or were an average of the OSR taken from many tides (85+, Brockmann and Smith 2009) over multiple seasons (DE and FL). The population in GA did not have a previously established OSR. In this case, we measured the OSR by walking along the beach over 1 week (May 10, 2010–May 16, 2010) during the time of the maximum high tides counting the number of male and female horseshoe crabs within a meter of the shoreline (only reproductively active adult horseshoe crabs come to the shoreline). This survey method is the same as that used for the FL population and similar to that used at the other sites. We calculated the OSR by dividing the total number of males by the total number of females observed on the beach during this week. The OSR for the GA population should be interpreted with caution since horseshoe crabs were scarcer during the week when we collected than previously reported (personal communication: Sheri L. Johnson and Matthew D. Smith).
At all six sites, we collected spawning adult males as we came upon them when walking along the shoreline during high tide (spring tides). Females normally lay multiple batches of eggs over 20–60 min (Brockmann 1990), and so males likely spawn multiple times after arriving at the beach. When possible, we collected males shortly after they arrived on the beach (attached males) or as they joined a spawning group (satellite males) to reduce the possibility that they had exhausted their sperm stores prior to collection. We tried to collect both attached and satellite males; however, at many sites, mating groups were scarce so we collected any male that arrived at the beach, including “lone” males (i.e., unattached males that have not yet found a mating group to join; Table 1). We gave each male a unique identifying tag. We held collected males without females so that males had no opportunity to mate. Males that we collected during night tides were kept in flow-through seawater tanks and processed within 12 h of collection. However, we collected most males during the day in which case they were processed within 2 h. Before we collected sperm, we placed each horseshoe crab in a 5-gal bucket for 5–10 min. We measured each male’s body size and age (see below) and collected a sample of sperm. We returned all horseshoe crabs to the beach from which they had been collected. No mortality occurred.
The number of animals collected differed at the six sites due to differences in the number of animals spawning and the amount of time available at each site. With the exception of males that did not meet certain criteria for the sperm velocity analysis (see below) or were mistakenly not measured (N = 8), we used every horseshoe crab that we collected from the beach in the analyses. The data from Seahorse Key, FL are a subset of the data we have used in previous studies (Sasson et al. 2012, 2015).
Measurements
We measured size across the widest point on the prosoma (PW). While it is impossible to measure the age of horseshoe crabs directly, male horseshoe crabs have a terminal molt (Shuster and Sekiguchi 2003; Smith et al. 2010) and their carapace degrades with age (Shuster 1955; Penn and Brockmann 1995; Brockmann 2002). In brief, we calculated relative horseshoe crab age based on this degradation using four categorical measures: (1) prosomal color, (2) the amount of prosomal pitting, (3) the amount of prosomal mucous, and (4) lateral eye condition (for more details, see Sasson et al. 2012). We scored each measurement from 1 to 3 with 3 indicating the best condition for that measure. We then added together the four scores for an index indicating relative age for each animal: the youngest males in the best condition for every measure were ranked as 12, while the oldest males in the worst condition were ranked as 4. We categorized males in the upper quartile of the condition (10–12, N = 66) as young, males in the lower quartile of the condition (4–5, N = 75) as old, and all other males (6–9, N = 164) as middle aged (Sasson et al. 2012). We measured condition from animals collected in the field and thus it was not possible to record condition data blind with respect to latitude and site.
Sperm collection
We placed each horseshoe crab dorsal side down on a table and applied 10 V at 0.5 A of electricity with an electro-stimulator for 10 s to the area around each gonopore (Sasson et al. 2012). We collected the ejaculate that flowed from the gonopores with a micropipette and placed each male’s ejaculate in individually marked micro-centrifuge tubes. These tubes were kept on an ice pack until we completed all sperm measurements (<1 h from time of collection).
Sperm and ejaculate measurements
We were interested in differences in sperm and ejaculate traits that may directly affect fitness rather than in sperm morphology, which has been more commonly studied. To that end, we measured four aspects of sperm and ejaculate quantity and quality: ejaculate size, sperm concentration, total sperm per ejaculate, and sperm velocity (see Sasson et al. 2012 for a detailed description of each measurement). Since we measured sperm and ejaculate traits within an hour of collection, it was not possible to record these data blind with respect to collection site. However, we analyzed all sperm and ejaculate traits except for ejaculate size (see below) blind with respect to the condition and mating tactic of the male.
Ejaculate size
Many studies have shown that relative testis size (also known as the gonadal somatic index) or the amount of testis tissue can reflect sperm production and may increase with selection via sperm competition (e.g., Gage et al. 1995; Neff et al. 2003; Firman et al. 2015). Unfortunately, horseshoe crab testicular tissues are intermixed with hepatic tissue (Benham 1884; Fahrenbach 1973), making it impossible to measure testis size directly. Instead, we measured ejaculate size which previous work has shown stays relatively consistent across individual male horseshoe crabs over time (Sasson et al. 2012). While this measurement does not quantify the total ejaculate available for each male, it does allow us to compare the relative amount of ejaculate immediately available across males. We stimulated each gonopore for 10 s, measured the amount of ejaculate released (μl), and then averaged the output from the two sides as a measure of ejaculate size.
Sperm concentration
Sperm concentration measures the number of spermatozoa/ml of ejaculate (e.g., Levitan et al. 1991; Benzie and Dixon 1994). Since horseshoe crab ejaculate is highly concentrated, we serially diluted each sample and fixed it with 2 % glutaraldehyde. We placed 10 μl of the fixed sample on an improved Neubauer hemocytometer and counted the number of spermatozoa within a given area on the slide. The number of spermatozoa counted in that area can then be used to calculate the number of spermatozoa/ml. We measured each sample twice and averaged the two values for each male.
Total sperm ejaculated
Ejaculate size and sperm concentration are two components of sperm quantity. A male’s ejaculate may be large but if that ejaculate is not highly concentrated then that male may still release fewer sperm than a male with a smaller but more concentrated ejaculate would. Thus, these two measurements can be combined to measure the total number of spermatozoa in the animal’s ejaculate (ejaculate size (in ml) × sperm concentration).
Sperm velocity
We measured sperm velocity using a subset of the sperm collected. We serially diluted the sample and activated the sperm by adding MgCl2 and shaking vigorously (Clapper and Brown 1980b). We then immediately placed 10 μl of the sample on an improved Neubauer hemocytometer slide and recorded 25 s of sperm movement with a black-and-white high-speed camera (Prosilica EC650) mounted on a phase-contrast microscope. We used the computer-assisted sperm analysis (CASA) add-on developed for ImageJ (Wilson-Leedy and Ingermann 2007) (v1.42d, National Institutes of Health) to measure sperm velocity from the first 10 s of the videos. All sperm velocity measurements are given as mean curvilinear velocity (VLC), a measurement of the point-to-point path of the sperm (Rurangwa et al. 2004). In order to eliminate non-sperm and account for the effect of movement due to water flow on the slide, only objects larger than five pixels and traveling faster than 25 μm/s in the video were used in our analysis. Additionally, we excluded any sample that contained fewer than 25 motile sperm (N = 22).
Statistical analyses
Before examining sperm quantity and quality, we determined whether horseshoe crabs from the six sites differed in body size and male age, factors that are known to influence sperm quantity and quality in the FL population (Sasson et al. 2012, 2015). We used an ANOVA to compare body size and a chi-square test to compare the number of males in each age category at the different collection sites.
To test whether sperm quantity and quality differed across our collection sites, we used an ANOVA with post hoc comparisons using Tukey’s HSD test. We log-transformed ejaculate size, total sperm ejaculated, and sperm velocity and square-root transformed sperm concentration before all analyses.
To account for effects of body size on sperm traits, we used an ANCOVA model with each sperm trait as the response variable, collection site as the explanatory variable, and body size as a covariate. We log-transformed body size before analysis for all models. We tested for an interaction effect between collection site and body size in these models but interaction effects were removed from the final model when p value was >0.20 (Tomkins and Simmons 2002). We conducted post hoc tests in these models using Tukey’s HSD.
We know that male age also correlates with sperm traits (Sasson et al. 2012). In order to test whether the variation in sperm traits across sites was due to differences in male age, we ran similar ANCOVA models with each sperm trait as the response variable, collection site as the explanatory variable, and age as a covariate. Since we did not find males from all ages at each collection site (see results), we could not test for an interactive effect between collection site and age. We conducted post hoc site comparisons using Tukey’s HSD.
We are also interested in other factors that might explain variation in sperm traits across populations. One such factor may be latitude. To test whether ejaculate and sperm traits follow a simple latitudinal gradient, we ran linear and non-linear regressions (quadratic and cubic) with latitude as the independent variable and the ejaculate or sperm trait as the dependent variable. We report the results of the linear regression. We also report a non-linear regression if that regression better fits the data than the linear regression, as measured by a lower AIC score.
Finally, we are interested in how variation in sperm traits might be explained by the OSR. For these analyses, we used standard least square regression models with each sperm trait as the dependent variable and OSR, body size, and OSR × body size interactions as explanatory variables. Any interaction term with a p value <0.20 (Tomkins and Simmons 2002) was removed from the final model.
All statistical analyses were conducted in JMP 12.0 (SAS Institute, Cary, NC, U.S.A.).
Results
Differences between sites
We found many differences between our six collection sites (Table 1). Male horseshoe crabs differed significantly in size with the largest males from GA and the smallest from ME (ANOVA, F 5, 231 = 354.1, p < 0.0001, Table 1). Body size does not follow a strict latitudinal gradient: horseshoe crabs increase in size from FL to GA and then are progressively smaller in more northern populations as others have found (e.g., Smith and Brockmann 2014). We also found significant differences between sites in the proportion of animals in different age categories (Pearson’s chi-squared test : χ 2 = 131.5, p < 0.0001). Horseshoe crabs from Florida had the highest percentage of young males within the population, whereas MA was the only site with no young males (Table 1).
Ejaculate size
Ejaculate size varied widely (Fig. 2a, ANOVA, F 5, 260 = 40.4, p < 0.0001). The animals in GA and DE had much larger ejaculates than horseshoe crabs from the other sites did, while the males in ME had the smallest ejaculates (Table 2). We still found significant differences between sites in ejaculate size after controlling for body size but found no significant interactions between collection site and body size (ANCOVA, Table 3). The horseshoe crabs from DE had significantly larger ejaculates than males from CT, MA, and ME (Tukey’s HSD, Fig. 2a); males from all other locations did not significantly differ from each other (Tukey’s HSD, Fig. 2a). After accounting for age differences between sites, we again find significant differences across sites in ejaculate size (Table 4), with males from GA and DE having significantly larger ejaculates than males from other sites (Tukey’s HSD, Fig. 2a).
Sperm trait variation across populations. Ejaculate size (a), concentration (b), sperm per ejaculate (c), and velocity (d) were significantly different at different sites. Capital letters indicate significant differences using ANOVA analyses. Numbers indicate significant differences in sperm traits at different sites from the ANCOVA analyses with body size as a covariate. Greek letters indicate significant differences in sperm traits at different sites from the ANCOVA analyses with age as a covariate. Error bars indicate standard error. Non-transformed data shown in figures
Ejaculate size did not follow a linear latitudinal gradient (linear regression, N = 265, r 2 = 0.0, p = 0.43). Instead, ejaculate size correlated with latitude non-linearly (cubic regression, N = 265, adjusted r 2 = 0.41, p < 0.0001).We found a positive correlation between the OSR and ejaculate size after accounting for body size (Table 5).
Sperm concentration
Sperm concentration differed significantly between sites (Fig. 2b, ANOVA, F 5, 284 = 22.6, p < 0.0001). Horseshoe crabs from DE and GA had more concentrated sperm than did animals from the other sites (Table 2). After accounting for body size, we still found a strong effect of site on sperm concentration (ANCOVA, Table 3). When body size was taken into consideration, horseshoe crabs from DE had the most concentrated sperm while those from CT, MA, and ME had the least concentrated sperm (Tukey’s HSD, Fig.2b). Differences across the sites remained when accounting for male age as well (Table 4): males from DE had the most concentrated sperm while males from MA had the least concentrated sperm (Tukey’s HSD, Fig. 2b).
Sperm concentration had a weak negative linear correlation with latitude (linear regression, N = 289, r 2 = 0.02, p < 0.01) and was more strongly correlated with latitude non-linearly (quadratic regression, N = 289, adjusted r 2 = 0.21, p < 0.0001). We saw no effect of the OSR on sperm concentration (Table 5).
Sperm per ejaculate
Horseshoe crabs from different sites differed in the number of sperm that were present in their ejaculates (Fig. 2c, ANOVA, F 5, 256 = 43.5, p < 0.0001). The males from GA and DE had the greatest number of sperm while the horseshoe crabs from ME had the fewest sperm per ejaculate (Table 2). We also found an effect of body size on sperm per ejaculate but this result was not statistically significant (ANCOVA, Table 3). However, there was a significant population × body size interaction effect: the males in DE had the most sperm per ejaculate while those from ME had the fewest (Fig. 2c). Variation across sites remained when age was taken into account (Table 4); males from GA and DE had the most sperm per ejaculate while males from ME had the fewest sperm in their ejaculates (Tukey’s HSD, Fig. 2c).
Total sperm per ejaculate more strongly correlated with latitude non-linearly (cubic regression, N = 261, adjusted r 2 = 0.42, p < 0.0001) than linearly (linear regression, N = 261, r 2 = 0.02, p = 0.02). We found that the OSR significantly affected the total sperm per ejaculate (Table 5).
Sperm velocity
We found differences across sites in average sperm velocity (Fig. 2d, ANOVA, F 5, 163 = 4.42, p < 0.001). Horseshoe crabs from CT had the fastest sperm, although they did not differ significantly from the horseshoe crabs in DE and MA. The horseshoe crabs in FL, GA, and ME had the slowest sperm (Table 2). While we saw no significant effect of body size, we did find a significant site × body size interaction effect (ANCOVA, Table 3). Males from CT had faster sperm than males from FL in this analysis, but all other sites did not differ in sperm velocity (Fig. 2d). After accounting for age, we still found significant differences across sites in sperm velocity (Table 4), with males from CT having faster sperm than males from FL, GA, and ME (Tukey’s HSD, Fig. 2d).
Sperm velocity did not significantly correlate with latitude linearly (linear regression, N = 169, r 2 = 0.01, p = 0.11) and only weakly correlated with latitude non-linearly (quadratic regression, N = 169, adjusted r 2 = 0.05, p = 0.03). OSR was not significantly correlated with sperm velocity (Table 5).
Discussion
Male horseshoe crabs differ in sperm quantity and quality as measured from natural populations across six sites from ME to FL. We know from work within one population of horseshoe crabs (FL) that body size and male age correlate with ejaculate size (Sasson et al. 2012), and studies from other species have also shown body size to correlate with ejaculate traits (Gage 1994; Lüpold 2013). Since the horseshoe crabs at our six collection sites significantly differed in body size and age, we conducted analyses that evaluated whether significant variation in sperm traits from the six sites remained when these differences were taken into account. We found that collection sites differed in ejaculate size (DE had significantly larger ejaculates than CT, MA, and ME), sperm concentration (DE had significantly more concentrated sperm than CT, MA, and ME), and sperm per ejaculate (DE had significantly more sperm per ejaculate than ME) when controlling for body size. There were also significant site by body size interaction effects for sperm per ejaculate and sperm velocity, indicating that the magnitude of the effect of body size on these traits differs across sites. We also found that sperm trait differences persist across sites when accounting for the effect of age. What might account for these patterns?
The sperm competition risk hypothesis (Parker 1982, 1998) predicts that increased sperm competition risk selects for sperm traits that increase post-copulatory reproductive competitiveness (i.e., sperm quantity and quality). OSR provides an estimate of sperm competition risk for horseshoe crabs spawning in our populations. While the OSR did not differ greatly for most of our sites (2.08–2.38), DE clearly stands apart (Table 1): males in DE likely face much more sperm competition than do males from any of the other locations. Strikingly, when body size is controlled for, males from the DE population have the largest ejaculates, the most concentrated sperm, and the most sperm per ejaculate (Fig. 2). Our analyses indicate that the OSR plays a significant role in the variation we see in ejaculate size and sperm per ejaculate (Table 5), even after accounting for the effects of body size. Taken together, these data suggest that the increased sperm and ejaculate quantity found in the DE population may be due to high post-copulatory selective pressures faced by males in this population, which support the predictions of Parker. However, we also found that males from GA have large ejaculates and high numbers of sperm per ejaculate despite the lower OSR of that population. It may be that factors we did not consider also influence sperm concentration. Alternatively, the OSR for the GA population may be less reliable than the OSR estimate from the other sites, since few horseshoe crabs were present the week we conducted our surveys at that site. A more complete census of the GA population would do much to resolve this potential issue.
None of the sperm traits were linearly correlated with latitude, i.e., the central sites, DE and GA, had larger ejaculates and more concentrated sperm than those to the north, CT, MA, ME, or to the south, FL (Table 2). This means that temperature, the length of the season, and other correlates of latitude are unlikely to explain the inter-population differences we observed. While we tried to collect males during the middle of the typical breeding season at each site, such precise timing is difficult when the start and end dates of a season may vary across years. Thus, for example, if our sampling occurred at the beginning of the season at one site but late in the season at another site, one might expect the earlier-season males to have larger ejaculates, all other factors being equal. Another possible explanation for the latitudinal patterns we see is that locations may differ in resource quantity or quality. Males from locations where resources are abundant may be able to devote more resources to sperm production. The horseshoe crab population in the mid-Atlantic states is many times larger than any other population (e.g. Shuster and Botton 1985), suggesting that resources in Delaware Bay are likely more abundant than elsewhere. However, no study has systematically compared the quality of diets of horseshoe crabs across these various locations, and so this resource-abundance hypothesis remains to be tested.
Body size, OSR, and age were all factors correlated with sperm and ejaculate quantity. Unfortunately, it is difficult to measure the relative strength of the association of each of these factors with sperm and ejaculate quantity and quality with samples from only six collection sites. Our ability to infer the effect of the OSR on these traits is further hindered by the fact that the OSR from only one population (DE) differed markedly from the rest of the locations we sampled. Sampling from additional locations, especially those with distinct OSRs (e.g. the horseshoe crab population in the Yucatan, Brockmann and Smith 2009), would do much to allow us to determine how these factors may be acting in concert or conflict to influence sperm trait variation.
Our one measure of sperm quality, sperm velocity, varied little across the six sites. Males from CT had the fastest sperm (Table 2). However, this result may simply be an effect of a small sample size. We excluded a higher percentage of individuals from Connecticut (66 %) than from any other population due to low numbers of motile sperm, leaving only 13 individuals from CT in our sperm velocity analysis (Table 1). Thus, it may be that only the CT males with the most motile sperm were analyzed, leading to our finding of faster sperm in that population. Why so many individuals from that population had few or no motile sperm is unclear. It is also unclear why sperm velocity overall showed less variation across the sites than did the other ejaculate and sperm traits. Horseshoe crab sperm are quiescent until activated by chemical cues from eggs (Clapper and Brown 1980b), and the sperm only detect these chemical cues when in close proximity to the eggs (Clapper and Brown 1980a). Thus, differences across horseshoe crabs in sperm velocity may not increase fertilization success as strongly as variation in other traits. However, this hypothesis has yet to be tested in horseshoe crabs and sperm velocity has been shown to be an important factor in competitive reproductive success in other externally fertilizing species (e.g., Levitan 2000; Gage et al. 2004; Rudolfsen et al. 2008). Sperm velocity may also be constrained by sperm morphology. If increases in sperm velocity require morphological changes to the sperm that are energetically costly or induce trade-offs with other sperm traits (Snook 2005), selection may act against changes that would reduce sperm quantity.
It is unknown how much of the variation we found across populations in ejaculate or sperm quantity and sperm quality is influenced by heritable variation, a plastic response to the immediate competitive environment, or a combination of both. Sperm traits have been shown to be heritable in a number of systems (Morrow and Gage 2001; Birkhead et al. 2005; Simmons and Moore 2009; Sasson et al. 2016), but they can also change rapidly through plastic responses based on the level of sperm competition (e.g., Gage et al. 1995; Gage and Barnard 1996; Wedell and Cook 1999; Immler et al. 2010). The variation we see could be due to selection on genes that affect sperm and ejaculate quantity and quality; alternatively, variation in levels of sperm competition may lead to population-specific optima through plastic changes in the regulation of sperm production. This could occur, for instance, if males from locations with a high OSR allocated relatively more resources to sperm production than males from locations with a low OSR. Data collected within the Florida population did not find evidence for rapid adjustments of sperm to the immediate competitive environment (Sasson et al. 2015), but that finding does not discount the possibility that consistently high levels of sperm competition could induce adjustments of sperm or ejaculate traits between populations. One way to test these two hypotheses would be to conduct a common garden experiment with males from multiple locations. Unfortunately, horseshoe crabs take many years to mature and are difficult to rear to adulthood (Schreibman and Zarnoch 2009), so such an experiment would be challenging.
In this study, we have shown that sperm and ejaculate quantity and quality vary across populations of horseshoe crabs and have suggested possible factors that may influence sperm trait divergence in this system. Sexual selection, through sperm competition risk, may in part drive sperm and ejaculate trait evolution across populations, although sampling from additional locations with a wider range of OSRs is needed to fully evaluate this hypothesis. If validated, these results may suggest that the same processes that lead to variation in sperm quantity and quality across closely related species (Gomendio and Roldan 1991; Gage 1994; Stockley et al. 1997; Balshine et al. 2001; Byrne et al. 2003; Rowe and Pruett-Jones 2011) may occur across populations. Factors other than sexual selection may affect post-copulatory trait divergence across species, including phylogenetic relatedness, and different reproductive morphology and physiology (Gomendio and Roldan 1991; Stockley et al. 1996; Hettyey and Roberts 2006). Examining the role of sexual selection in sperm trait divergence across populations of a single species may avoid many of these confounding factors and warrants additional research.
References
Balshine S, Leach BJ, Neat F, Werner NY, Montgomerie R (2001) Sperm size of African cichlids in relation to sperm competition. Behav Ecol 12:726–731
Benham WBS (1884) On the testis of Limulus. Trans Linn Soc Lond 2:363–366
Benzie J, Dixon P (1994) The effects of sperm concentration, sperm: egg ratio, and gamete age on fertilization success in crown-of-thorns starfish (Acanthaster planci) in the laboratory. Bio Bull 186:139–152
Birkhead TR, Pellatt EJ, Brekke P, Yeates R, Castillo-Juarez H (2005) Genetic effects on sperm design in the zebra finch. Nature 434:383–387
Brockmann HJ (1990) Mating-behaviour of horseshoe crabs, Limulus polyphemus. Behaviour 114:206–220
Brockmann HJ (2002) An experimental approach to altering mating tactics in male horseshoe crabs (Limulus polyphemus). Behav Ecol 13:232–238
Brockmann HJ, Penn D (1992) Male mating tactics in the horseshoe crab, Limulus polyphemus. Anim Behav 44:653–665
Brockmann HJ, Smith MD (2009) Reproductive competition and sexual selection in horseshoe crabs. In: Tanacredi JT, Botton ML, Smith DR (eds) Biology and conservation of horseshoe crabs. Springer, New York, NY, pp 199–221
Brockmann HJ, Colson T, Potts W (1994) Sperm competition in horseshoe crabs (Limulus polyphemus). Behav Ecol Sociobiol 35:153–160
Brockmann HJ, Nguyen C, Potts W (2000) Paternity in horseshoe crabs when spawning in multiple-male groups. Anim Behav 60:837–849
Brockmann HJ, Johnson SL, Smith MD, Sasson D (2015) Mating tactics of the American horseshoe crab (Limulus polyphemus). In: Changing global perspectives on horseshoe crab biology, conservation and management. Springer., pp 321–351
Byrne PG, Simmons LW, Roberts JD (2003) Sperm competition and the evolution of gamete morphology in frogs. Proceedings of the Royal Society of London Series B-Biological Sciences 270:2079–2086
Clapper DL, Brown GG (1980a) Sperm motility in the horseshoe-crab, Limulus polyphemus L.1. sperm behavior near eggs and motility initiation by egg extracts. Dev Biol 76:341–349
Clapper DL, Brown GG (1980b) Sperm motility in the horseshoe-crab, Limulus polyphemus L.2. partial characterization of a motility initiating factor from eggs and the effects of inorganic cations on motility initiation. Dev Biol 76:350–357
Crudgington HS, Fellows S, Badcock NS, Snook RR (2009) Experimental manipulation of sexual selection promotes greater male mating capacity but does not alter sperm investment. Evolution 63:926–938
Dziminski MA, Roberts JD, Beveridge M, Simmons LW (2010) Among-population covariation between sperm competition and ejaculate expenditure in frogs. Behav Ecol 21:322–328
Elgee K, Evans J, Ramnarine I, Rush S, Pitcher T (2010) Geographic variation in sperm traits reflects predation risk and natural rates of multiple paternity in the guppy. J Evol Biol 23:1331–1338
Emlen ST, Oring LW (1977) Ecology, sexual selection, and evolution of mating systems. Science 197:215–223
Endler JA, Houde AE (1995) Geographic-variation in female preferences for male traits in Poecilia reticulata. Evolution 49:456–468
Evans JP, Magurran AE (1999) Geographic variation in sperm production by Trinidadian guppies. Proc R Soc Lond Ser B-Biol Sci 266:2083–2087
Fahrenbach W (1973) Spermiogenesis in horseshoe crab Limulus polyphemus. Journal of Morphology 140:31–51
Firman RC, Garcia‐Gonzalez F, Thyer E, Wheeler S, Yamin Z, Yuan M, Simmons LW (2015) Evolutionary change in testes tissue composition among experimental populations of house mice. Evolution 69:848–855
Fitzpatrick JL, Montgomerie R, Desjardins JK, Stiver KA, Kolm N, Balshine S (2009) Female promiscuity promotes the evolution of faster sperm in cichlid fishes. P Natl Acad Sci USA 106:1128–1132
Gage MJG (1994) Associations between body-size, mating pattern, testis size and sperm lengths across butterflies. Proc R Soc Lond Ser B-Biol Sci 258:247–254
Gage A, Barnard C (1996) Male crickets increase sperm number in relation to competition and female size. Behav Ecol Sociobiol 38:349–353
Gage MJG, Stockley P, Parker GA (1995) Effects of alternative male mating strategies on characteristics of sperm production in the Atlantic salmon (Salmo salar): theoretical and empirical investigations. Philos T R Soc B 350:391–399
Gage MJG, Macfarlane CP, Yeates S, Ward RG, Searle JB, Parker GA (2004) Spermatozoal traits and sperm competition in Atlantic salmon: relative sperm velocity is the primary determinant of fertilization success. Currt Biol 14:44–47
Gomendio M, Roldan ERS (1991) Sperm competition influences sperm size in mammals. Proc R Soc Lond Ser B-Biol Sci 243:181–185
Hettyey A, Roberts JD (2006) Sperm traits of the quacking frog, Crinia georgiana: intra- and interpopulation variation in a species with a high risk of sperm competition. Behav Ecol Sociobiol 59:389–396
Hosken DJ, Garner TWJ, Ward PI (2001) Sexual conflict selects for male and female reproductive characters. Currt Biol 11:489–493
Hunter FM, Birkhead TR (2002) Sperm viability and sperm competition in insects. Currt Biol 12:121–123
Immler S, Pryke SR, Birkhead TR, Griffith SC (2010) Pronounced within-individual plasticity in sperm morphometry across social environments. Evolution 64:1634–1643
Irwin DE (2000) Song variation in an avian ring species. Evolution 54:998–1010
Johnson KT (2016) Population genetic analysis of Atlantic horseshoe crabs (Limulus polyphemus) in Coastal Massachusetts. University of Massachusetts
King TL, Eackles MS, Spidle AP, Brockmann HJ (2005) Regional differentiation and sex-biased dispersal among populations of the horseshoe crab Limulus polyphemus. T Am Fish Soc 134:441–465
King TL, Eackles MS, Aunins AW, Brockmann HJ, Hallerman E, Brown BL (2015) Conservation genetics of the American horseshoe crab (Limulus polyphemus): allelic diversity, zones of genetic discontinuity, and regional differentiation. In: Changing global perspectives on horseshoe crab biology, conservation and management. Springer., pp 65–96
Kleven O, Fossøy F, Laskemoen T, Robertson RJ, Rudolfsen G, Lifjeld JT (2009) Comparative evidence for the evolution of sperm swimming speed by sperm competition and female sperm storage duration in passerine birds. Evolution 63:2466–2473
Laskemoen T, Albrecht T, Bonisoli-Alquati A, Cepak J, de Lope F, Hermosell IG, Johannessen LE, Kleven O, Marzal A, Mousseau TA, Møller AP, Robertson RJ, Rudolfsen G, Saino N, Vortman Y, Lifjeld JT (2013) Variation in sperm morphometry and sperm competition among barn swallow (Hirundo rustica) populations. Behav Ecol Sociobiol 67:301–309
Levitan DR (2000) Sperm velocity and longevity trade off each other and influence fertilization in the sea urchin Lytechinus variegatus. Proc R Soc Lond Ser B-Biol Sci 267:531–534
Levitan DR, Sewell MA, Chia F-S (1991) Kinetics of fertilization in the sea urchin Strongylocentrotus franciscanus: interaction of gamete dilution, age, and contact time. Biol Bull 181:371–378
Lindholm AK, Head M, Brooks R, Rollins L, Ingleby F, Zajitschek SR (2014) Causes of male sexual trait divergence in introduced populations of guppies. J Evol Biol 27:437–448
Lüpold S (2013) Ejaculate quality and constraints in relation to sperm competition levels among eutherian mammals. Evolution 67:3052–3060
Lüpold S, Linz GM, Birkhead TR (2009a) Sperm design and variation in the New World blackbirds (Icteridae). Behav Ecol Sociobiol 63:899–909
Lüpold S, Linz GM, Rivers JW, Westneat DF, Birkhead TR (2009b) Sperm competition selects beyond relative testes size in birds. Evolution 63:391–402
Lüpold S, Westneat DF, Birkhead TR (2011) Geographical variation in sperm morphology in the red-winged blackbird (Agelaius phoeniceus). Evol Ecol 25:373–390
Manier MK, Palumbi SR (2008) Intraspecific divergence in sperm morphology of the green sea urchin, Strongylocentrotus droebachiensis: implications for selection in broadcast spawners. Bmc Evol Biol 8:283
Morrow EH, Gage MJG (2001) Sperm competition experiments between lines of crickets producing different sperm lengths. Proc R Soc Lond Ser B-Biol Sci 268:2281–2286
Neff BD, Fu P, Gross MR (2003) Sperm investment and alternative mating tactics in bluegill sunfish (Lepomis macrochirus). Behav Ecol 14:634–641
Oppliger A, Hosken D, Ribi G (1998) Snail sperm production characteristics vary with sperm competition risk. Proc R Soc Lond Ser B-Biol Sci 265:1527–1534
Parker GA (1982) Why are there so many tiny sperm - sperm competition and the maintenance of 2 sexes. J Theor Biol 96:281–294
Parker GA (1998) Sperm competition and the evolution of ejaculates: towards a theory base. In: Birkhead TR, quot f-f, Cambria, quot, p.MsoNormal lMdM, pt f-s, quot f-f, Roman, quot TN, Section dS, Møller AP (eds) Sperm Competition and Sexual Selection. Academic Press, pp 3 - 54
Penn D, Brockmann HJ (1995) Age-biased stranding and righting in male horseshoe crabs, Limulus polyphemus. Anim Behav 49:1531–1539
Pitcher TE, Stutchbury BJM (1998) Latitudinal variation in testis size in six species of North American songbirds. Can J Zoolog 76:618–622
Pitnick S, Miller GT, Schneider K, Markow TA (2003) Ejaculate-female coevolution in Drosophila mojavensis. Proc R Soc Lond Ser B-Biol Sci 270:1507–1512
Riska B (1981) Morphological variation in the horseshoe-crab Limulus polyphemus. Evolution 35:647–658
Rowe M, Pruett-Jones S (2011) Sperm competition selects for sperm quantity and quality in the Australian Maluridae. PLoS One 6:e15720–e15720
Rudolfsen G, Figenschou L, Folstad I, Kleven O (2008) Sperm velocity influence paternity in the Atlantic cod (Gadus morhua L.). Aquac Res 39:212–216
Rurangwa E, Kime DE, Ollevier F, Nash JP (2004) The measurement of sperm motility and factors affecting sperm quality in cultured fish. Aquaculture 234:1–28
Sasson DA, Johnson SL, Brockmann HJ (2012) The role of age on sperm traits in the American horseshoe crab, Limulus polyphemus. Anim Behav 84:975–981
Sasson DA, Johnson SL, Brockmann HJ (2015) Reproductive tactics and mating contexts affect sperm traits in horseshoe crabs (Limulus polyphemus). Behav Ecol Sociobiol 69:1769–1778
Sasson DA, Munoz PR, Gezan SA, Miller CW (2016) Resource quality affects weapon and testis size and the ability of these traits to respond to selection in the leaf‐footed cactus bug, Narnia femorata. Ecol Evol 6:2098–2108
Schreibman MP, Zarnoch CB (2009) Aquaculture methods and early growth of juvenile horseshoe crabs (Limulus polyphemus). In: Tanacredi JT, Botton ML, Smith DR (eds) Biology and conservation of horseshoe crabs. Springer, New York
Shine R, Fitzgerald M (1995) Variation in mating systems and sexual size dimorphism between populations of the Australian python Morelia spilota (Serpentes, Pythonidae). Oecologia 103:490–498
Shuster CN (1955) On morphometric and serological relationships within the Limulidae, with particular reference to Limulus polyphemus (L.). New York University
Shuster CN, Botton ML (1985) A contribution to the population biology of horseshoe crabs, Limulus polyphemus (L.), in Delaware Bay. Estuaries 8:363–372
Shuster CJ, Sekiguchi K (2003) Growing up takes about ten years and eighteen stages. In: Shuster CJ, Barlow R, Brockmann H (eds) The American horseshoe crab. Harvard University Press, Cambridge, pp 103–132
Simmons LW, García‐González F (2008) Evolutionary reduction in testes size and competitive fertilization success in response to the experimental removal of sexual selection in dung beetles. Evolution 62:2580–2591
Simmons LW, Moore AJ (2009) Evolutionary quantitative genetics of sperm. In: Birkhead TR, Hosken DJ, Pitnick S (eds) Sperm biology: an evolutionary perspective. Academic, Oxford, UK
Smith DR, Brousseau LJ, Mandt MT, Millard MJ (2010) Age and sex specific timing, frequency, and spatial distribution of horseshoe crab spawning in Delaware Bay: insights from a large-scale radio telemetry array. Curr Zool 56:563–574
Smith MD, Brockmann HJ (2014) The evolution and maintenance of sexual size dimorphism in horseshoe crabs: an evaluation of six functional hypotheses. Anim Behav 96:127–139
Snook RR (2001) Absence of latitudinal clines in sperm characters in North American populations of Drosophila subobscura (Diptera : Drosophilidae). Pan-Pac Entomol 77:261–271
Snook RR (2005) Sperm in competition: not playing by the numbers. Trends Ecol Evol 20:46–53
Stewart KA, Wang R, Montgomerie R (2016) Extensive variation in sperm morphology in a frog with no sperm competition. BMC Evol Biol 16:1
Stockley P, Gage MJG, Parker GA, Møller AP (1996) Female reproductive biology and the coevolution of ejaculate characteristics in fish. Proc R Soc Lond Ser B-Biol Sci 263:451–458
Stockley P, Gage MJG, Parker GA, Møller AP (1997) Sperm competition in fishes: the evolution of testis size and ejaculate characteristics. Am Nat 149:933–954
Stoltz JA, Neff BD (2006) Sperm competition in a fish with external fertilization: the contribution of sperm number, speed and length. J Evol Biol 19:1873–1881
Tomkins JL, Simmons LW (2002) Measuring relative investment: a case study of testes investment in species with alternative male reproductive tactics. Anim Behav 63:1009–1016
Wedell N, Cook PA (1999) Butterflies tailor their ejaculate in response to sperm competition risk and intensity. Proc R Soc Lond Ser B-Biol Sci 266:1033–1039
Wilson-Leedy JG, Ingermann RL (2007) Development of a novel CASA system based on open source software for characterization of zebrafish sperm motility parameters. Theriogenology 67:661–672
Acknowledgments
We especially thank Melissa Clark for her help in collecting samples from FL to MA. We received additional help at various sampling sites from, but not limited to, Gracie Townsend and Brian Silliman (GA); Gary Kraemer (DE); Jennifer Mattei (CT); Sarah Martinez and Katherine Johnson (MA); and Pete Thayer, Kristen Aaltonen, and Eric Aaltonen (ME). We also thank the Stolarz Foundation and the National Science Foundation (IOB 06-41750) whose grants made sampling at these sites possible. Finally, we thank two anonymous reviewers for their many helpful comments on this manuscript.
Author information
Authors and Affiliations
Corresponding author
Ethics declarations
Funding
This study was funded by the National Science Foundation (IOB 06-41750) and the Stolarz Foundation.
Conflict of interest
The authors declare that they have no competing interests.
Ethical approval
All applicable international, national, and/or institutional guidelines for the care and use of animals were followed.
Additional information
Communicated by N. Wedell
Rights and permissions
About this article
Cite this article
Sasson, D.A., Brockmann, H.J. Geographic variation in sperm and ejaculate quantity and quality of horseshoe crabs. Behav Ecol Sociobiol 70, 1715–1725 (2016). https://doi.org/10.1007/s00265-016-2177-0
Received:
Revised:
Accepted:
Published:
Issue Date:
DOI: https://doi.org/10.1007/s00265-016-2177-0